Abstract
This research article investigates the one-pot synthesis of gold and silver chloride nanoparticles functionalized by fruit extract of Crataegus pinnatifida as reducing and stabilizing agents and their possible roles as novel anti-inflammatory agents. Hawthorn (C. pinnatifida) fruits are increasingly popular as raw materials for functional foods and anti-inflammatory potential agents because of abundant flavonoids. The reduction of auric chloride and silver nitrate by the aqueous fruit extract led to the formation of gold and silver chloride nanoparticles. The nanoparticles were further characterized by field emission transmission electron microscopy indicated that CP-AuNps and CP-AgClNps were hexagonal and cubic shape, respectively. According to X-ray diffraction results, the average crystallite sizes of CP-AuNps and CP-AgClNps were 14.20 nm and 24.80 nm. The biosynthesized CP-AgClNps served as efficient antimicrobial agents against Escherichia coli and Staphylococcus aureus. Furthermore, CP-AuNps and CP-AgClNps enhanced the DPPH radical scavenging activity of the fruit extract. Lastly, MTT assay of nanoparticles demonstrated low toxicity in murine macrophage (RAW264.7). Biosynthesized nanoparticles also reduced the production of the inflammatory cytokines including nitric oxide and prostaglandin E2 in lipopolysaccharide-induced RAW264.7 cells. Altogether, these findings suggest that CP-AuNps and CP-AgClNps can be used as novel drug carriers or biosensors with intrinsic anti-inflammatory activity.
Introduction
Green methodology for the synthesis of metallic nanoparticles and their applications have been garnering enthusiasm from the scientific community due to the unique physiochemical features of nano-sized materials [Citation1]. Gold and silver chloride nanoparticles, in particular, are of great scientific interests because of their wide biomedical applications as antimicrobial agents, drug delivery agents, and biosensors [Citation1]. An important and challenging task in nanoparticle synthesis is the development of a simple, rapid, and eco-friendly methodology with tunable morphology, specific biological activities, and simplicity of bio-conjugation with active constituents [Citation2]. Recently, various inorganic nanoparticles such as metals, metal oxides, metal sulphides and metal chlorides have been successfully synthesized by green synthesis; a number of approaches have been established for the synthesis of silver chloride nanoparticles (AgClNps) by chemical method [Citation3], host-guest nanocomposite material [Citation4], radiation [Citation5], photochemical methods [Citation6], and electro-spinning [Citation7]. Gold nanoparticles (AuNps), on the other hands, have been profoundly manufactured over the years by Brust-Schiffrin and Turkevich methods [Citation8]. Nevertheless, these methodologies employ high energy consumption and/or highly reactive and toxic chemicals [Citation9]. Alternatively, biological synthesis of metal nanoparticles utilizes natural products which are rich in biological diversity such as flavonoids, triterpenoids, essential oils, etc. Among several biological species, various plant extracts and microorganisms have been extensively studied for this favourable substitute [Citation10,Citation11]. This green route circumvents the side effects and the energy consumption of chemical and physical methods by avoiding the utilization of toxic chemicals and creating hazardous by products.
For the first time, we report the implementation of Crataegus pinnatifida fruit extract for the biosynthesis of gold (CP-AuNps) and silver chloride (CP-AgClNps) nanoparticles. Hawthorn (C. pinnatifida) is a traditional Chinese medicinal herb which belongs to the Rosaceae family and is widely distributed in the north of China [Citation12,Citation13]. From a pharmacological point of view, hawthorn fruits possess favourable therapeutic properties, such as cardiovascular protective, anti-inflammatory, antioxidant, and antimicrobial, due to their various active compounds; so far over 150 active constituents have been isolated from C. pinnatifida [Citation13]. Thus, this phytochemical-rich extract is favourable for the synthesis of multifunctional nanoparticles for biomedical applications. By exploiting the anti-inflammatory properties of flavonoids present in dried fruit extract, we aim to synthesize biologically active CP-AuNps and CP-AgClNps and investigate their cytotoxicity in murine macrophage (RAW264.7) and lipopolysaccharide (LPS)-induced RAW264.7 cells as well as their ability to induce cellular expression towards the regulation of pro-inflammatory cytokines, which are critical in the inflammatory response system [Citation14].
The biosynthesized nanoparticles were characterized by ultra–violet visible (UV–Vis) spectroscopy, field emission transmission electron microscopy (FE-TEM), energy-dispersive X-ray (EDX) spectroscopy, elemental mapping, X-ray powder diffraction (XRD), selected area electron diffraction (SAED), dynamic light scattering (DLS), and Fourier transform-infrared (FT-IR) spectroscopy. Furthermore, CP-AuNps and CP-AgClNps were investigated for their antioxidant activity against 2,2-diphenyl-1-picrylhydrzyl (DPPH) free radicals. Additionally, the antimicrobial properties of CP-AgClNps were tested against pathogenic Staphylococcus aureus and Escherichia coli.
Materials and methods
Materials
The dried fruits of C. pinnatifida were obtained from Ginseng Resource bank, Kyung Hee University, South Korea. Gold (III) chloride trihydrate (HAuCl4·3H2O), silver nitrate (AgNO3), DPPH, and 3,4,5-trihydroxybenzoic acid (gallic acid) were purchased from Sigma-Aldrich Chemicals, St. Louis, MA, USA. The pathogenic bacterial strains Staphylococcus aureus [ATCC 6538] and Escherichia coli [ATCC 10798] were employed as model Gram-positive and Gram-negative bacteria, respectively. Standard Neomycin (NEO30) discs (30 µg/disc) were purchased from Oxoid Ltd., England, UK.
Preparation of C. Pinnatifida fruit extract
Crataegus pinnatifida fruit powder was obtained after washing the fruits with distilled water and grinding the dried fruit. Then, 10 g of dried fruit powder was suspended into 100 ml of sterile water and autoclaved for 60 min at 100 °C to leach out natural plant products. Finally, the resulting suspension was filtered using a Whatman filter paper and centrifuged at 10,000 rpm for 10 min to remove suspended solids. The total volume of filtrate was maintained at 100 ml and stored at 4 °C for further use.
Determination of the total phenolic and total flavonoids content of C. pinnatifida extract
Total phenolic content of extract solution was determined by a modified Folin–Ciocalteu method according to Baba and Malik [Citation15]. Briefly, 1 ml the extract solution was mixed thoroughly with 1 ml of 2 N Folin–Ciocalteu reagent and 1 ml of 10% (w/v) sodium carbonate. The resulting mixture was kept 30 min in the dark and the absorbance was measured at 750 nm. The total phenolic content was calculated from the calibration curve and the results were expressed as mg of gallic acid equivalent per mL.
Total flavonoids content of extract solution was determined by a modified chromogenic method [Citation16]. In brief, 0.5 ml extract solution was mixed thoroughly with 1 ml of 95% ethanol. Next, 0.25 ml of sodium nitrite solution (4%, w/v) was added to the mixture. After 6 min of reaction, 0.25 ml of aluminium nitrate solution (10%, w/v) was added. Similarly, 1.5 ml of sodium hydroxide solution (4%, w/v) was added to the reaction mixture 6 min later. At the end of the reaction, the absorbance was measured at 510 nm. The total phenolic content was calculated from the calibration curve and the results were expressed as mg of rutin equivalent per mL.
Synthesis of CP-AuNps and CP-AgClNps
The obtained fruit extract was solely employed to synthesize CP-AuNps and CP-AgClNps. HAuCl4·3H2O (gold salt) and AgNO3 (silver salt) were dissolved into the aqueous fruit extract (10%, v/v) with a final concentration of 1 mM in the corresponding reaction mixtures. The reaction mixtures were then incubated at 80 °C in an oil bath to initiate the reduction of Au3+ and Ag+ into Au and Ag atoms. After some time, colour change was subsequently observed, which indicated the formation of nanoparticles in the reaction mixtures. After the synthesis, the nanoparticles were purified by repeated high speed centrifugation at 16,000 rpm for 15 min and washing with distilled water. Finally, supernatant was removed and nanoparticles were air-dried overnight and obtained in powder form for the ensuing characterization.
Characterization of CP-AuNps and CP-AgClNps
Each aliquot from the reaction mixtures was scanned in the range of 300–800 nm by a UV–vis spectrophotometer (Ultrospec 2100 Pro, Amersham Biosciences) using a 1 cm quartz cuvette to confirm the presence of nanoparticles in the coloured mixtures. The morphology and elemental composition of metallic nanoparticles were analysed by FE-TEM, EDX, SAED, and elemental mapping with a JEM-2100 F (JEOL, Peabody, MA, USA) electron microscope operated at 200 kV. The specimens were prepared by placing droplets of nanoparticle suspension onto a copper grid coated with a carbon film. The samples were subsequently dried in an oven at 60 °C and transferred to FE-TEM for analysis. SAED patterns were also obtained to study the crystallite structure of the biosynthesized nanoparticles. DLS data were obtained by a zeta potential and particle size analyser ELSZ-2000 series (Otsuka Electronics Photal, Osaka, Japan). Pure water with a refractive index of 1.3328, viscosity of 0.8878, and dielectric constant of 78.3 was used as a dispersive medium. XRD analysis was performed on an energy dispersive X-ray spectrometer (D8 Advance, Bruker, Germany). The running conditions for the instrument were 40 kV and 40 mA. Cu-Kα radiation (λ = 1.54 Å) was used for the scans. Samples were scanned over the 2θ range of 20 – 80° at a scanning rate of 6°/min with an interval of 0.02°. The average crystal size diameter of metal nanoparticles was calculated by Debye–Scherrer equation: D = 0.9λ/β cos θ, where D is the crystallite size in nm, λ is the wavelength of Cu-Kα radiation in nm, β is the full width at half maximum (FWHM) in radians, and θ is the half of the Bragg angle in radians. FTIR was performed using a FTIR spectrophotometer (PerkinElmer Inc., Waltham, MA, USA) over the range of 4000–450 cm−1 at a resolution of 4 cm−1. All characterization experiments were conducted at room temperature.
In vitro biological studies of CP-AuNps and CP-AgClNps
Antimicrobial activity assay
Determination of CP-AgClNps antimicrobial activity by disc diffusion test
The antimicrobial activities of CP-AuNps and CP-AgClNp were evaluated against pathogenic microorganisms E. coli and S. aureus using the disc diffusion method. In this assay, the Mueller–Hinton agar (MHA) plates were spread evenly with 100 μL of overnight culture of pathogenic bacteria in lysogeny broth (LB) medium. An optical density (OD) of 0.1 was achieved by broth dilution. Commercial Neomycin 30 µg/disc was maintained as positive control. Each sterile paper disc was deluged by 30 μL (500, 1000, 1500 µg/mL) of freshly prepared and purified nanoparticle suspension on MHA plates. The plates were incubated at 37 °C for 24 h. After the incubation period, the zones of inhibition around each disc were measured [Citation17,Citation18]. Experiments were subjected to triplications to calculate mean and standard errors.
Determination of minimum inhibitory concentration (MIC) and minimum bactericidal concentration (MBC) for CP-AgClNps
Minimum inhibitory concentration (MIC), defined as the lowest concentration of material that inhibits the growth of pathogens, was determined based on liquid batch cultures containing varying concentration of CP-AgClNps in suspension (1–100 μg/mL). 0.5 ml of LB media containing CP-AgClNps were prepared and then inoculated with 1 ml of the freshly prepared E. coli and S. aureus to maintain initial bacterial concentration of 103–104 CFU/mL. The bacterial cultures were incubated in an orbital shaker at 180 rpm and room temperature for 24 h. Bacterial growth, measured in absorbance, was recorded by UV–Vis spectrophotometer at 600 nm. The negative controls (LB media without nanoparticles) indicated the microbial growth profile in the absence of nanoparticles. All the experiments were carried out in triplicate.
Minimum inhibitory concentration, or the lowest concentration of nanoparticles that kills 99.9% of the pathogen, was also determined. The presence of viable microorganisms was tested and the lowest concentration causing bactericidal effect was reported as MBC as suggested by Avadi et al. [Citation19]. To test for bactericidal effect, 10 μL from each MIC test flask were inoculated on nutrient agar and incubated at 25 °C for 24 h. The nanoparticle concentration causing bactericidal effect was selected based on the absence of colonies on the agar plate.
DPPH radical scavenging assay
Antioxidant assay of biosynthesized CP-AuNps, CP-AgClNps, and aqueous C. pinnatifida fruit extract were evaluated using a modified method of DPPH analysis [Citation8,Citation20]. Each specimen of 20 µL in distilled water was mixed with 180 µL of 1 mM methanolic DPPH solution. Different concentrations of furit extract, CP-AuNps and CP-AgClNps (100, 250, 500, 1000, 1500, 2000 µg/mL) were adopted. The reaction mixture was sonicated in dark condition at room temperature for 30 min. The absorbance values were recorded at 517 nm using UV–Vis spectrophotometer (UV–Vis). The radical scavenging activity was expressed as percentage inhibition: [(Absorbancecontrol – Absorbancesample)/Absorbancecontrol] × 100, where Absorbancesample is the absorbance of the DPPH solution with nanoparticles and Absorbancecontrol is the absorbance of the DPPH solution without nanoparticles. Gallic acid of potent antioxidant was used as positive control [Citation21]. Experiments were subjected to triplications to calculate mean and standard errors.
In vitro MTT cell cytotoxicity assay
In vitro cytotoxicity assay of nanoparticles was performed with a method previously reported [Citation22,Citation23]. Briefly, RAW264.7 cells (KCLB, Seoul, Korea) were cultured in Dulbecco’s modified eagles medium (DMEM) (Gibco-BRL, Grand Island, NY, USA) supplemented with 10% fetal bovine serum (FBS) and 1% penicillin/streptomycin (P/S) (WelGENE Inc., Daegu, Korea) at 37 °C in a humidified atmosphere containing 5% CO2 and 95% air. In vitro cytotoxicity of CP-AuNps and CP-AgClNps in RAW264.7cells was evaluated using 3-(4, 5-dimethyl-2-thiazolyl)-2, 5-diphenyl-2H tetrazolium bromide (MTT) (Life Technologies, Eugene, OR, USA) assay [Citation24]. Cell lines were seeded at a density of 1 × 104 per well in a 96-well plate (Corning Costar, Lowell, NY, USA). Next, the wells were treated with different concentrations of nanoparticles (0, 1, 10, 50, 100 and 200 µg/mL) at 37 °C for 24 h at 90% confluences. After incubation, 10 μL of MTT (5 mg/mL, PBS) assay solution was added to each well. The wells were further incubated at 37 °C for 4 h; viable cells would then convert MTT into purple-coloured formazan. The resulting insoluble formazan precipitate was dissolved by 100 μL of DMSO and quantified with an absorbance maximum at 570 nm by an Enzyme-Linked Immunosorbent Assay (ELISA) reader (Bio-Tek Instruments, Inc., Winooski, VT, USA). The optical density of formazan formed in untreated cells (negative control) represents 100% cell viability. Similarly, in vitro cytotoxicity of nanoparticles in LPS-stimulated RAW264.7 cells were performed. Experiments were subjected to triplications to calculate mean and standard errors.
In vitro measurement of NO and PGE2 production in LPS-induced RAW264.7 cells assay
Measurement of pro-inflammatory cytokines nitric oxide (NO) and prostaglandin E2 (PGE2) was made possible by LPS stimulation of RAW264.7 macrophages. Cells were plated in 96-well plates at 1 × 105 cells/well, pretreated with different concentrations of CP-AuNps and CP-AgClNps for 1 h, and then stimulated with 1 µg/mL LPS (Sigma-Aldrich, St. Louis, MO, USA). After incubation for a day, the culture supernatants were collected. Production of NO was determined by colorimetric Griess reaction: briefly a known volume (100 µL) of pre-mixed Griess reagent (Sigma-Aldrich, St. Louis, MO, USA) was added into each culture supernatant of equal volume, and incubated for 10 min. The absorbance of each sample was read at 540 nm against a standard curve of sodium nitrite using a Synergy 2 multimode microplate reader. Quantification of PGE2 was performed using an enzyme-linked immunosorbent assay (ELISA) kit (R&D Systems, Minneapolis, MN, USA) according to the protocol supplied by the manufacturer.
Results and discussion
Synthesis of nanoparticles
To the best of our knowledge, this is the first report to highlight the ability of dried fruit extract of C. pinnatifida to reduce and stabilize metal nanoparticles. The reduction of gold and silver salt was visually evident from the colour change of the reaction mixture after the incubation period at 80 °C as shown from the insets from . The synthesis of CP-AuNps and CP-AgClNps was monitored visually by a change in colour to reddish brown and dark brown, respectively. The reduction of Au3+ to Au° was completed within 2 min of incubation at 80 °C. The reaction mixture completely turned to a reddish brown which resembles the collective oscillations of free electrons in a nanoparticle suspension upon interaction with light, and specific for CP-AuNps [Citation25]. Thus, the appearance of a reddish brown colour in the reaction mixture indicated the formation of CP-AuNps. The characteristic surface plasmon resonance (SPR) band of the CP-AuNps was observed in the UV–vis spectrum, which showed a maximum absorption peak at 550 nm for the CP-AuNps () [Citation10]. For CP-AgClNps, the reaction mixture completely turned to yellow brown after 50 min of incubation at 80 °C. Similarly, the bright brown colour was due to the excitation of surface plasmon resonance (SPR) of the synthesized CP-AgClNps (Mulvaney. 1996). In addition, an intense peak at 426 nm was observed which corresponds to the formation of CP-AgClNps () [Citation26].
Characterization of nanoparticles
The results obtained from FE-TEM analysis give a clear indication regarding the configuration of the nanoparticles. FE-TEM analysis indicated that CP-AuNps and CP-AgClNps were hexagonal and cubic shape, respectively ()). Based on FE-TEM results, a higher frequency of hexagonal and cubic nanoparticles were observed in comparison to other nanoparticles, such as spherical or rod-shaped nanoparticles. The nanoparticle shape is greatly affected by the nature of the surfactant, pH, temperature, and pressure [Citation27]. Spherical shape is achieved by nanoparticles to attain the minimum surface energy in lieu of additional energy. However, the shape of nanoparticles is also dependent on the interactions of biomolecules present in the reaction mixtures (i.e. C. pinnatifida fruit extract) and the preparation method. The temperature conditions (80 °C) and the plant biomolecules, which further act as stabilizers, may give rise to different non-spherical shapes of CP-AuNps and CP-AgClNps. Their purity and elemental composition were analysed through EDX and elemental mapping (). As shown in EDX spectra, strong signals were observed at 2.3 (gold), 2.6 (chloride), and 3 (silver) keV which are characteristic peaks of elemental gold, chloride, and silver. The results were in line with previous reports of EDX spectra of gold and silver chloride nanoparticles [Citation25]. Other signals reported at 8 keV originated from elemental carbon and copper which are exploited at FE-TEM grids. The elemental mapping results indicate maximum distribution of gold, silver and chloride element in the purified nanoproducts [Citation28]. The distribution profile of the biosynthesized CP-AuNps and CP-AgClNps was studied using dynamic light scattering (DLS) method by particle size analyser according to intensity, number, and volume (). The Z-average hydrodynamic diameters of CP-AuNps and CP-AgClNps were approximately 172.2 and 312.2 nm, respectively. The polydispersity index of CP-AuNps was found to be 0.16, while that of CP-AgClNps was 0.17. Additionally, XRD analyses were performed to confirm the monocrystalline nature of CP-AuNps and CP-AgClNps (). The CP-AuNps exhibited intense peaks in the whole spectrum of 2θ value ranging from 20 to 80° corresponding to [111], [200], [220] and [311] (). This pattern was similar to the Braggs’s reflection of gold nanocrystals indicating that the purified product is composed of pure crystalline gold [Citation29]. The X-ray diffraction pattern of CP-AgClNps displayed intense peaks in the whole spectrum of 2θ value ranging from 20 to 80 corresponding to [111], [200], [220], [311], [222] and [420] planes of the cubic silver chloride nanoparticles (ICDD file no.00-001-1013) (). The average crystallite size was determined from X-ray diffraction analysis data using the Scherrer equation. The crystallite average sizes were calculated to be 14.20 nm and 24.80 nm for CP-AuNps and CP-AgClNps, respectively. In addition, the selected area electron diffraction (SAED) pattern revealed the crystalline nature of the biosynthesized nanoparticles ()). The contradiction in the average size diameter obtained from Scherrer equation and DLS could be attributed to the fact that the hydrodynamic diameter takes into account the inclusion of plant biomolecules around the nanoparticles as a colloidal suspension, as opposed to the core metallic diameter in its dried form determined by Scherrer. Lastly, FTIR is an important tool for the identification of functional groups and interactions between said molecules and the nanoparticle surfaces [Citation30]. FTIR analysis revealed visible bands at 3247.25, 2923.07, 1619.75 and 1075.42 cm−1 for CP-AuNps, and at 3424.89, 2923.07, 1618.81 and 1048.05 cm−1 for CP-AgClNps () [Citation31]. Bands in the range of 3247.25 and 3424.89 cm−1 represent O–H and/or N–H stretching. Peak recorded at 2923.07 cm−1 represents C-H aromatic stretching. The peaks at 1619.75 and 1618.81 cm−1 could be attributed to C = C aromatic stretching. Peaks ranging from 1075.42 to 1048.08 cm−1 indicate the presence of C–O stretching. Previous reported that flavonoids, which are rich in O–H, C–H and C = C functional groups, were the main active compounds found in the hot-water extraction from dried fruit of C. pinnatifida [Citation13,Citation32]. Thus, it is suggested that the formation of the self-assembled C. pinnatifida functionalized CP-AuNps and CP-AgClNps may be contributed to the non-covalent interactions of these aforementioned biomolecules detected in C. pinnatifida extract on the surface of these well-dispersed metallic nanoparticles.
Figure 2. Physicochemical characterization of CP-AuNps (a) and CP-AgClNps (b). Notes: Field emission transmission electron micrographs showed that CP-AuNps and CP-AgClNps are hexagon and square-spherical shape (a-i, a-iii, b-i, and b-iii) and the selected area electron diffraction pattern revealed the crystalline nature of the biosynthesized nanoparticles (a-ii and b-ii). Elemental mapping (a-iv, b-iv and b-v) and energy dispersive X-ray spectroscopy (a-v and b-vi) confirmed the purity of the nanoparticles.
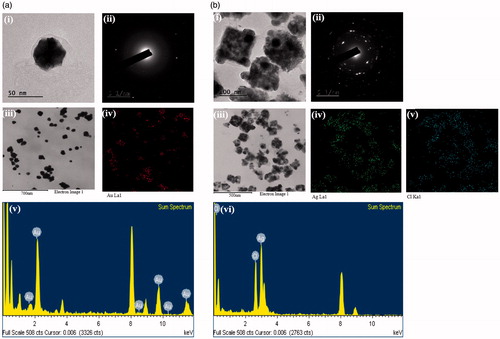
In vitro biological studies results of CP-AuNps and CP-AgClNps
Antimicrobial activity of CP-AgClNps
The antimicrobial activity of gold and silver nanoparticles has been widely characterized and exploited commercially as antimicrobial agents in consumer products, antimicrobial coatings, and biomedical devices [Citation33,Citation34]. In our study, disc diffusion test was employed to check the antimicrobial activities of biosynthesized CP-AgClNps, as compared against standard antibiotics. Results of the assay have been shown in ). The pathogenic strains E. coli (Gram-negative) and S. aureus (Gram-positive) were shown to be sensitivity to both NEO30 and CP-AgClNps; the latter showed a dose-dependent antimicrobial activity. Increase in concentration of CP-AgClNps resulted in larger zone of inhibition. MIC and MBC for CP-AgClNps against E. coli and S. aureus were also evaluated in . According to the results, the MIC90 for E. coli and S. aureus were 5 µg/mL and 10 µg/mL, respectively, and the MBC for E. coli and S. aureus were 10 µg/mL and 50 µg/mL, respectively. These findings support the previous reports of antimicrobial activity of AgNps against E. coli and S. aureus [Citation35]. The MIC and MBC of AgNPs from Zizyphus spina christi L leaves extract were calculated to be 46 and 57 µg/ml against S. aureus and 59 and 82 µg/ml against E. coli [Citation17]. Comparing with these findings, CP-AgClNps showed stronger antimicrobial activity than the reported silver nanoparticles [Citation17].
Figure 6. Antimicrobial activity of nanoparticles in comparison with antibiotics against Escherichia coli [ATCC 10798] (a), Staphylococcus aureus [ATCC 6538] (b). Zone of inhibition of CP-AgClNps and Neomycin (N30) as standard antibiotics as control against E. coli and S. aureus (c). Values of MIC and MBC for CP-AgClNps against E. coli and S. aureus (d). Error bars represent the standard deviation (n = 3).
![Figure 6. Antimicrobial activity of nanoparticles in comparison with antibiotics against Escherichia coli [ATCC 10798] (a), Staphylococcus aureus [ATCC 6538] (b). Zone of inhibition of CP-AgClNps and Neomycin (N30) as standard antibiotics as control against E. coli and S. aureus (c). Values of MIC and MBC for CP-AgClNps against E. coli and S. aureus (d). Error bars represent the standard deviation (n = 3).](/cms/asset/feffaac3-3dda-4522-9560-f0f808cfe3a2/ianb_a_1376674_f0006_c.jpg)
The improved antimicrobial activity could be due to the amount of silver ions released from the nanoparticles which act as reservoirs against bacteria [Citation36]. Additionally, the antimicrobial activity may also be contributed to the flavonoid functionalized on the nanoparticle surfaces; some studies have reported the strong antimicrobial activity of various flavonoids found in fruit extract [Citation37]. In the case of CP-AuNps, no activity was observed at the same concentrations. A previously reported study demonstrated the antimicrobial activity of silver chloride nanoparticles against gram negative bacteria [Citation34]. Herein, the biosynthesized CP-AgClNps successfully inhibited the growth of both gram negative and positive bacteria. The biosynthesis of CP-AgClNPs and their antimicrobial potential will be helpful in providing an insight in the development of viable antimicrobial agents in near future.
In vitro DPPH radical scavenging activity of CP-AuNps and CP-AgClNps
The antioxidant activity of the fruit extract, biosynthesized CP-AuNps and CP-AgClNps was evaluated in vitro [Citation38]. DPPH reducing ability of fruit extract, CP-AuNps and CP-AgClNps was assessed colometrically at 517 nm. DPPH is composed of stable free-radical molecules and is readily reduced by accepting hydrogen or electron from fruit extract and nanoparticles [Citation21]. The results of the findings are summarized in . The results on the effect of different concentrations of fruit extract, CP-AuNps, and CP-AgClNps revealed that they possessed free radical scavenging activity in a dose-dependent manner. Ascorbic acid was maintained as positive control. The antioxidant activity of CP-AuNps and CP-AgClNps were found to be higher than that of fruit extract with the same concentration. At concentrations below 1500 µg/mL, the scavenging activity of CP-AgClNps was higher than the activity of CP-AuNps, however as the concentrations were increased to 2000 µg/mL the activity displayed an almost flat response. Finally at 2000 µg/mL, the percentage inhibition of CP-AuNps is 52.10%, while the percentage inhibition of CP-AgClNps is 43.92%. The IC50 of CP-AuNps is 1.926 mg/mL as determined by linear interpolation. The imparted antioxidant activity may be due to the enhancement of fruit extract onto the surface of nanoparticles. The total phenolic and flavonoid contents of the fruit extract of C. pinnatifida were calculated accordingly; using the chromogenic methods, results showed that the total phenolic content in extract solution were 2.0 ± 0.16 mg/mL and the total flavonoids contents were 1.6 ± 0.28 mg/mL. In this study, we report that the biosynthesized CP-AuNps and CP-AgClNps by dried fruit extract of C. pinnatifida possess enhanced antioxidant activity and demonstrate potentials as free radical scavengers in biomedical applications.
In vitro cytotoxicity of CP-AuNps and CP-AgClNps in RAW264.7 cells
The in vitro cytotoxicity of CP-AuNps and CP-AgClNps was examined in murine macrophage (RAW264.7) by MTT assay. RAW264.7cells showed cell viability after 24 h treatment with 1, 10, 50, 100 and 200 µg/mL of CP-AuNps and CP-AgClNps. As shown in , CP-AuNps at concentrations ranging from 1 to 100 µg/mL had no effect on the viability of RAW264.7 cells. Similarly, CP-AgClNps at concentrations ranging from 1 to 50 µg/mL did not exhibit any significant cytotoxicity against RAW264.7 cells. Subsequent experiments were performed at 100 µg/mL for CP-AuNps and 50 µg/mL for CP-AgClNps. Significant cytotoxicity (< 50% cell viability) caused by nanoparticles was observed at 200 µg/mL and 100 µg/mL for CP-AuNps and CP-AgNps, respectively. Similar studies have reported that silver nanoparticles caused higher cytotoxicity than gold nanoparticles at the same concentrations against various cell lines. Likewise, CP-AgClNps were found to be more toxic than CP-AuNps against pre-diseased RAW264.7 cells [Citation22,Citation39].
Figure 8. MTT assay was used to examine the effect of various concentrations of CP-AuNps (a) and CP-AgClNps (b) on the RAW264.7cells. Notes: The cells were incubated with the nanoparticles for 24 h, and the experiment was repeated three times independently. Error bars represent the standard deviation (n ≥ 3). ***p < .001 versus control (untreated group).
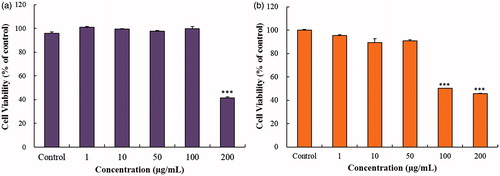
Effects of CP-AuNps and CP-AgClNps on the production of NO and PGE2 in LPS-induced RAW264.7 cells
NO and PGE2 have been known to play crucial roles in the inflammatory-signalling pathway as pro-inflammatory cytokines [Citation40,Citation41]. As shown in , NO and PGE2 expressions were increased significantly as RAW264.7 cells were stimulated by LPS (1 µg/mL). The production levels of NO and PGE2 were suppressed by CP-AuNps and CP-AgClNps in LPS-stimulated RAW264.7 cells in a dose-dependent manner. In case of nitrate production, the treatment of CP-AuNps at 50 µg/mL decreased NO production well below the level attained by positive control, L-NMMA (50 µM) (). Furthermore, the treatment of CP-AuNps and CP-AgClNps at different concentrations resulted in a dose-dependent decrease of PGE2 expression in LPS-stimulated RAW264.7 cells (). CP-AuNps were demonstrated superior pro-inflammatory cytokine suppression than CP-AgNps at the same concentrations. Thus, these results suggest that CP-AuNps and CP-AgNps greatly ameliorate the release of NO and PGE2 in LPS-induced RAW264.7 cells. Further research was required to elucidate the activity of these nanoparticles on the inflammatory response of both iNOS and COX-2 through the NF-κb signalling. Altogether, these findings suggest that CP-AuNps and CP-AgClNps can be used as novel drug carriers with intrinsic anti-inflammatory activity to sites of inflammation.
Figure 9. Effects of CP-AuNps and CP-AgClNps on LPS-induced expression of nitric oxide (NO) (a) and PGE2 production (b) in RAW 264.7 cells. Notes: The experiment was repeated at least three times. Error bars represent the standard deviation (n ≥ 3). ##.001< p ≤ .01 and ###p ≤ .001 versus control cells; *.01< p ≤ .05, **.001< p ≤ .01 and ***p ≤ .001 versus LPS-treated cells.
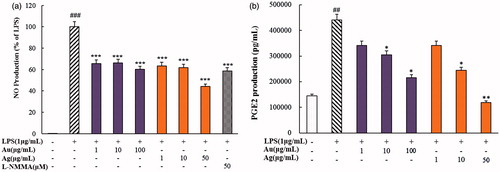
Conclusions
A one-pot synthesis of CP-AuNps and CP-AgClNps functionalized by aqueous fruit extract of C. pinnatifida was carried out in this study. This facile methodology highlights the use of green chemistry without hazardous by-product and non-toxic chemicals. The biosynthesized CP-AgClNps served as efficient antimicrobial agents against pathogenic strains E. coli and S. aureus. Furthermore, CP-AuNps and CP-AgClNps also showed superior scavenging activity against DPPH free radicals than aqueous fruit extract with the same concentrations. Lastly, in vitro cytotoxicity of nanoparticles was examined in murine macrophage (RAW264.7) and lipopolysaccharide (LPS)-induced RAW264.7 cells. CP-AuNps and CP-AgClNps expressed anti-inflammatory effects through reduction of the inflammatory cytokines including nitric oxide (NO) and prostaglandin E2 (PGE2). Altogether, these findings suggest that CP-AuNps and CP-AgClNps can be used as novel drug carriers with intrinsic anti-inflammatory activity to sites of inflammation. The results obtained from physicochemical characterization, MTT, antimicrobial, and antioxidant activity of nanoparticles support their plausible application in medical field. In the future, selection of such medicinal plants with high therapeutic importance will create a new platform for the nanoparticles synthesis.
Disclosure statement
No potential conflict of interest was reported by the authors.
Additional information
Funding
References
- Raveendran P, Fu J, Wallen SL. Completely “green” synthesis and stabilization of metal nanoparticles. J Am Chem Soc. 2003;125:13940–13941.
- Kemp MM, Kumar A, Mousa S, et al. Synthesis of gold and silver nanoparticles stabilized with glycosaminoglycans having distinctive biological activities. Biomacromolecules. 2009;10:589–595.
- Li L, Zhu YJ. High chemical reactivity of silver nanoparticles toward hydrochloric acid. J Colloid Interface Sci. 2006;303:415–418.
- Zhao L, Wang Y, Chen Z, et al. Preparation, characterization, and optical properties of host–guest nanocomposite material SBA-15/AgI. Physica B. 2008;403:1775–1780.
- Choi M, Shin K-H, Jang J. Plasmonic photocatalytic system using silver chloride/silver nanostructures under visible light. J Colloid Interface Sci. 2010;341:83–87.
- Hu C, Lan Y, Qu J, et al. Ag/AgBr/TiO2 visible light photocatalyst for destruction of azodyes and bacteria. J Phys Chem B. 2006;110:4066–4072.
- Bai J, Li Y, Li M, et al. Electrospinning method for the preparation of silver chloride nanoparticles in PVP nanofiber. Appl Surf Sci. 2008;254:4520–4523.
- Markus J, Mathiyalagan R, Kim YJ, et al. Intracellular synthesis of gold nanoparticles with antioxidant activity by probiotic Lactobacillus kimchicus DCY51T isolated from Korean kimchi. Enzyme Microb Technol. 2016;95:85–93.
- Dahl JA, Maddux BL, Hutchison JE. Toward greener nanosynthesis. Chem Rev. 2007;107:2228–2269.
- Elavazhagan T, Arunachalam KD. Memecylon edule leaf extract mediated green synthesis of silver and gold nanoparticles. Int J Nanomedicine. 2011;6:1265–1278.
- Wang C, Kim YJ, Singh P, et al. Green synthesis of silver nanoparticles by Bacillus methylotrophicus, and their antimicrobial activity. Artif Cell Nanomed Biotechnol. 2015;44:1127–1132.
- Wang C, Lu B, Hou G. Chemical constituent, pharmacological effects and clinical application of Crataegus pinnatifida. Strait Pharm J. 2010;3:75–78.
- Wu J, Peng W, Qin R, et al. Crataegus pinnatifida: chemical constituents, pharmacology, and potential applications. Molecules. 2014;19:1685–1712.
- Ahn S, Singh P, Castro-Aceituno V, et al. Gold nanoparticles synthesized using Panax ginseng leaves suppress inflammatory-mediators production via blockade of NF-κB activation in macrophages. Artif Cell Nanomed Biotechnol. 2017;45:270–276.
- Baba SA, Malik SA. Determination of total phenolic and flavonoid content, antimicrobial and antioxidant activity of a root extract of Arisaema jacquemontii Blume. J Taibah Univ Sci. 2015;9:449–454.
- Lin Y, Lu MF, Liao HB, et al. Content determination of the flavonoids in the different parts and different species of Abelmoschus esculentus L. by reversed phase-high performance liquid chromatograph and colorimetric method. Phcog Mag. 2014;10:278.
- Halawani EM. Rapid biosynthesis method and characterization of silver nanoparticles using Zizyphus spina christi leaf extract and their antibacterial efficacy in therapeutic application. J Biomater Nanobiotechnol. 2017;8:22–35.
- Soshnikova V, Kim YJ, Singh P, et al. Cardamom fruits as a green resource for facile synthesis of gold and silver nanoparticles and their biological applications. Artif Cell Nanomed Biotechnol. Forthcoming. [cited 2017 Mar 14]:[10 p.]. DOI:10.1080/21691401.2017.1296849
- Avadi M, Sadeghi A, Tahzibi A, et al. Diethylmethyl chitosan as an antimicrobial agent: Synthesis, characterization and antibacterial effects. Eur Polym J. 2004;40:1355–1361.
- Ramya S, Shanmugasundaram T, Balagurunathan R. Biomedical potential of actinobacterially synthesized selenium nanoparticles with special reference to anti-biofilm, anti-oxidant, wound healing, cytotoxic and anti-viral activities. J Trace Elem Med Biol. 2015;32:30–39.
- Mishra K, Ojha H, Chaudhury NK. Estimation of antiradical properties of antioxidants using DPPH assay: a critical review and results. Food Chem. 2012;130:1036–1043.
- Wang C, Mathiyalagan R, Kim YJ, et al. Rapid green synthesis of silver and gold nanoparticles using Dendropanax morbifera leaf extract and their anticancer activities. Int J Nanomed. 2016;11:3691–3701.
- Huo Y, Singh P, Kim YJ, et al. Biological synthesis of gold and silver chloride nanoparticles by Glycyrrhiza uralensis and in vitro applications. Artif Cell Nanomed Biotechnol. Forthcoming. [cited 2017 Apr 4]; [13 p.]. DOI:10.1080/21691401.2017.1307213
- Ahn S, Siddiqi MH, Noh H-Y, et al. Anti-inflammatory activity of ginsenosides in LPS-stimulated RAW 264.7 cells. Sci Bull. 2015;60:773–784.
- Geethalakshmi R, Sarada D. Gold and silver nanoparticles from Trianthema decandra: synthesis, characterization, and antimicrobial properties. Int J Nanomed. 2012;7:5375–5384.
- Tang Y, Subramaniam VP, Lau TH, et al. In situ formation of large-scale Ag/AgCl nanoparticles on layered titanate honeycomb by gas phase reaction for visible light degradation of phenol solution. Appl Catal B-Environ. 2011;106:577–585.
- Mulvaney P. Surface plasmon spectroscopy of nanosized metal particles. Langmuir. 1996;12:788–800.
- Lifeng Q, Xu ZR, Jiang X, et al. Preparation and antibacterial activity of chitosan nanoparticles. Carbohydr Res. 2004;339:2693–2700.
- Sharma B, Purkayastha DD, Hazra S, et al. Biosynthesis of gold nanoparticles using a freshwater green alga, Prasiola crispa. Mater Lett. 2014;116:94–97.
- Kong J, Yu S. Fourier transform infrared spectroscopic analysis of protein secondary structures. Acta Biochim Biophys Sinica. 2007;39:549–559.
- Li R, Dong W, He H, et al. Isolation, characterization and phase transformation of new ginsenoside compound k hydrate and methanol solvates. Cryst Res Technol. 2012;47:377–384.
- Chu C-Y, Lee M-J, Liao C-L, et al. Inhibitory effect of hot-water extract from dried fruit of Crataegus pinnatifida on low-density lipoprotein (LDL) oxidation in cell and cell-free systems. J Agric Food Chem. 2003;51:7583–7588.
- Dar MA, Ingle A, Rai M. Enhanced antimicrobial activity of silver nanoparticles synthesized by Cryphonectria sp. evaluated singly and in combination with antibiotics. Nanomed-Nanotechnol Biol Med. 2013;9:105–110.
- Dhas TS, Kumar VG, Karthick V, et al. Facile synthesis of silver chloride nanoparticles using marine alga and its antibacterial efficacy. Spectroc Acta Pt A-Molec Biomolec Spectr. 2014;120:416–420.
- Paredes D, Ortiz C, Torres R. Synthesis, characterization, and evaluation of antibacterial effect of Ag nanoparticles against Escherichia coli O157: H7 and methicillin-resistant Staphylococcus aureus (MRSA). Int J Nanomed. 2014;9:1717–1729.
- Feng Q, Wu J, Chen G, et al. A mechanistic study of the antibacterial effect of silver ions on Escherichia coli and Staphylococcus aureus. J Biomed Mater Res. 2000;52:662–668.
- Cushnie TT, Lamb AJ. Antimicrobial activity of flavonoids. Int J Antimicrob Agents. 2005;26:343–356.
- Debnath R, Purkayastha DD, Hazra S, et al. Biogenic synthesis of antioxidant, shape selective gold nanomaterials mediated by high altitude lichens. Mater Lett. 2016;169:58–61.
- Abbai R, Mathiyalagan R, Markus J, et al. Green synthesis of multifunctional silver and gold nanoparticles from the oriental herbal adaptogen: Siberian ginseng. Ijn. 2016;11:3131–3143.
- Su Y-W, Chiou W-F, Chao S-H, et al. Ligustilide prevents LPS-induced iNOS expression in RAW 264.7 macrophages by preventing ROS production and down-regulating the MAPK, NF-κB and AP-1 signaling pathways. Int Immunopharmacol. 2011;11:1166–1172.
- Tomita Y, Rikimaru-Kaneko A, Hashiguchi K, et al. Effect of anionic and cationic n-butylcyanoacrylate nanoparticles on NO and cytokine production in Raw264. 7 cells. Immunopharmacol Immunotoxicol. 2011;33:730–737.
- Patra JK, Baek KH. Green nanobiotechnology: factors affecting synthesis and characterization techniques. J Nanomater. 2014;2014:417305. DOI:10.1155/2014/417305