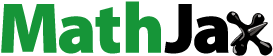
Abstract
Niclosamide (NCS) is an oral anthelminthic drug having low solubility and hence low bioavailability. Current investigation shows an approach to fabricate solid lipid nanoparticles (SLNs) of NCS and evaluated for pharmaceutical, in vitro and in vivo characterization. NFM-3 showed particle size 204.2 ± 2.2 nm, polydispersity index 0.328 ± 0.02 and zeta potential –33.16 ± 2 mV. Entrapment efficiency and drug loading capacity were 84.4 ± 0.02% and 5.27 ± 0.03%, respectively. Scanning electron microscopy image indicated that particles were nanoranged. DSC and P-XRD results showed change in physicochemical properties of NCS. FT-IR spectra confirmed compatibility between NCS and excipients. The drug release profile showed sustained release (93.21%) of NCS in 12 h. Different kinetic models showed zero-order kinetics and Case-II transport mechanism. Study showed maximum stability at refrigerated temperature. In vivo pharmacokinetic study showed 2.15-fold increase in NCS peak plasma concentration as solid lipid nanoparticle formulation (NFM-3) compared to commercial product while relative bioavailability was 11.08. Results including in vitro and in vivo release studies of NCS confirmed that SLNs system is suitable to improve oral delivery of NCS with increased aqueous solubility, permeability and finally bioavailability.
Introduction
Swift advancement in nanoparticles fabrication of identical size, composition and shape has started revolution in the field of pharmaceutical sciences. The assimilation of drugs into nanocarrier systems gives a new archetype in drugs delivery, used for drug targeting at secondary and tertiary levels [Citation1,Citation2]. Development of lipid-based drug delivery systems has also attracted the attention of researchers since last decade. Solid lipid nanoparticles (SLNs) socialize the advantages of both polymeric nanoparticles and emulsions while overcoming disadvantages of these two approaches [Citation3]. In the field of pharmaceutical nanotechnology, SLNs are leading due to numerous potential uses in pharmaceutical drug delivery systems [Citation4]. SLNs are being extensively used to enhance bioavailability of Biopharmaceutical Classification System (BCS) class II category and targeted drug delivery [Citation5]. Owing to their unique size dependent properties, SLNs offer distinctive opportunity for the development of novel ways to treat the diseases [Citation6]. On the other hand, SLNs as oral dosage form, potentially enhance the lymphatic transport, thus reducing hepatic first pass metabolism and boosted oral bioavailability [Citation7].
Niclosamide (NCS) is an oral anthelminthic drug having chemical name 5-chloro-N-(2-chloro-4-nitrophenyl)-2-hydroxybenzamide [Citation8]. NCS is yellowish or yellowish-white, fine crystals, practically insoluble in water and slightly soluble in ethanol [Citation9]. NCS is used for five decades against tapeworm infections including Taenia saginata, Diphyllobothrium latum, Taenia solium and Hymenolepis nana infections [Citation10].
NCS acts by inhibiting oxidative phosphorylation in mitochondria and anaerobic ATP production [Citation11]. Modern studies show that NCS is very effective against cancerous cell [Citation12]. It has shown anti-proliferative activity but the exact mechanism against cancer cells is unknown [Citation13]. Besides, NCS gave striking anti-tumour activity in animal models [Citation8].
Niclosamide belongs to BCS-II drugs having poor water solubility, i.e. 0.23 μg/ml [Citation14]. One of the modern approaches to enhance NCS solubility is to modify it chemically and several water-soluble NCS derivatives have been created [Citation15]. But chemical modification may affect safety and efficacy of NCS. The current study is based on fabrication of NCS loaded SLNs, i.e. size reduction to nano-scale, resulting in increased aqueous solubility with improved oral bioavailability.
Materials and methods
Materials
Niclosamide was a generous gift from Shaigan Pharmaceuticals Pvt. Ltd (Rawalpindi, Pakistan), and stearic acid (SA), Tween-80 and Poly Ethylene Glycol-400 were purchased from Acros Organics Thermo Fisher Scientific (Mullica Hill, NJ). Dialysis bags were purchased from Spectrum Lab (Brockville, Canada). Other chemicals used in this study were of analytical grade.
Methods
Preparation of unloaded SLNs
Micro-emulsion technique was optimized to fabricate unloaded SLNs through four variables, i.e. different concentrations of SA, Tween-80, Polyethylene Glycol (PEG) and stirring time. Twelve different SLN formulations (UFM-1 to UFM-12) were prepared (). Stearic acid was melted 5 °C above its melting point, Tween-80 was dissolved in deionized water and heated up to 75 °C with continuous magnetic stirring at 1200 rpm. Both oily phase and aqueous phase were mixed and continued stirring for different time intervals to obtain micro emulsion followed by addition of cold water (1:25) under magnetic stirring to get SLNs dispersion. The SLNs dispersion was centrifuged at 30,000 rpm for 10 min at room temperature [Citation16]. Zeta-size and polydispersity index were measured by zeta-sizer Nano (ZS-90, Malvern Instruments, Malvern, UK) [Citation17].
Table 1. Unloaded SLNs formulations.
Preparation of Niclosamide loaded SLNs
Using the optimized conditions of UFM-11, five different formulations (NFM-1 to NFM-5) of NCS loaded SLNs were prepared on basis of lipid drug ratio (). NCS was dissolved in melted SA, the rest of protocol followed was same as used for unloaded SLNs. Schematic diagram for fabrication of NCS-SLN is shown in .
Table 2. Different NCS loaded SLN formulations.
Lyophilization
The NCS-SLNs were lyophilized by means of freeze dryer (Heto PowerDry LL1500-Thermo Electron Corporation, Beverly, MA). Fructose solution (5%) was used as cryoprotectant [Citation18]. After overnight storage at −20 °C, SLNs were transferred to freeze dryer (−75 °C) and lyophilized for 48 h [Citation19].
Entrapment efficiency (EE) and drug loading capacity (DLC)
After preparation, NCS-SLNs samples were centrifuged [Citation2]. The supernatant was analysed for un-entrapped drug by Nano Drop Spectrophotometer (Thermo Scientific 2000c/2000 UV-VIS Spectrophotometer, Waltham, MA) and percent EE was calculated by using equation:
The DLC was also calculated by using equation:
Characterization of NCS loaded SLNs
Photon correlation spectroscopy
Zeta-size, PDI and zeta-potential (ζ) were recorded by photon correlation spectroscopy using a Zeta-sizer Nano ZS-90, Malvern Instruments (Malvern, UK) at 90° scattering angle and 25 °C [Citation20].
Scanning electron microscopy
For morphological studies, scanning electron microscopy (SEM) was performed at magnification of 30,000× and acceleration voltage of 20 kV using Scanning Electron Microscope JSM-910, JEOL (Tokyo, Japan) [Citation21,Citation22].
Differential scanning calorimetry
Thermal properties of the unprocessed NCS (drug) and processed NCS (NFM-3) were studied by differential scanning calorimeter (Perkin Elmer, Waltham, MA). Samples were heated from 40 °C to 300 °C at heating rate of 10 °C/min [Citation23].
Powder X-ray diffraction (P-XRD)
Powder X-ray diffraction (JEOL, Tokyo, Japan) technique was applied to analyse crystalline nature of NCS (drug) and NFM-3 [Citation24]. P-XRD analysis was carried out by CuKa radiation and scanned up to 80°, step size 2Ø-0.05° and step time 1.0 s, divergence slit 1°, receiving slit 0.2 mm and scattering slit 1°.
Fourier transform infrared (FT-IR) radiation measurements
Fourier transform Infrared spectrometer (IR Prestige 21, Shimadzu, Kyoto, Japan) study was conducted to check the compatibility among formulation’s components. Spectra of pure NCS and NFM-3 were taken in the range of 2000–700 cm−1. For compatibility, the peaks and patterns shaped by the pure NCS were compared with the NFM-3 formulation [Citation25,Citation26]. The spectra were recorded at room temperature.
Stability study
The freshly prepared NFM-3 formulation was divided into two parts and stored under refrigerated (5 ± 3 °C) and room (25 ± 2 °C) temperature for 90 days [Citation27]. Average particle size and PDI were measured for both temperatures at different time intervals. Statistically data were analysed by using the two-tailed t-test (p < .05).
In vitro study
In vitro release studies were performed using dialysis bag method [Citation28]. Dialysis bags of molecular weight cut off 12–14 kDa (Spectrum Lab, Brockville, Canada) was soaked in deionized water for about 12 h before use. NCS-SLNs dispersion (1 ml) from each formulation was transferred to dialysis bag and both ends of dialysis bag were fixed with thread. Each sample was separately placed into 250 ml phosphate-buffer solution (pH 7.4) at 50 rpm and samples were taken for analysis after specific interval of time (1–12 h) while same volume of phosphate-buffer solution was replaced. Amount of drug release was determined by using UV Spectrophotometer (λmax 332 nm) against blank phosphate buffer solution [Citation29]. Data from in vitro release studies were fitted into numerous kinetic models to find release rate and mechanism followed [Citation30].
In vivo pharmacokinetics
Oral administration
Rabbits (2 ± 0.3 kg) were kept fasted for 12 h before oral administration but given access to water. Two groups of rabbits were made each having six animals. NFM-3 was administered to Group-I while marketed drug (Mesan®) to Group-II (100 mg kg−1). Blood samples (0.5 ml) were collected from marginal ear vein at various time intervals (0–24 h). Blood was heparinized and plasma was separated by centrifugation which was stored at −20 °C till analysis.
Determination of NCS in plasma
Plasma was quantified for NCS by using HPLC technique [Citation28]. Acetonitrile:potassium dihydrogen phosphate (40:60, v/v) were used as mobile phase. Reversed phase column (Supelco C18, 25 cm in length, 4.6 mm width and 5 µm particle size) protected with a pre-column (Supelco C18) was used. The column was kept at 37 °C, flow rate 1 ml/min and retention time 4.5 min. Plasma samples were mixed with two volumes of acetonitrile and placed at −20 °C for 10 min followed by centrifugation to precipitate protein. The supernatant (20 µl) was injected for the detection of NCS concentration using UV detector (λmax 290 nm) [Citation31]. The concentration was quantified from area of chromatographic peak by using calibration curve.
Analysis of HPLC data
Pharmacokinetic limits (parameters) were determined based on non-compartmental model. Area under the curve from 0 to t (AUC0→t) was calculated from concentration–time curve by trapezoidal rule [Citation32]. Peak plasma concentration (Cmax) and peak plasma concentration time (Tmax) were obtained from the individual plasma concentration–time curve. Area under the total plasma concentration–time curve from time 0 to 24 h was determined by equation:
where Ct is the NCS concentration observed after 24 h and Ke is the apparent elimination rate constant. Relative bioavailability (Fr) at 24 hour for the equal dose was determined by equation:
Data of pharmacokinetic parameters were statistically analysed by one-way analysis of variance and t-test (p < .05).
Results
Zeta-sizer study
Unloaded SLNs were optimized based on different variable factors. Significant changes were observed by changing these variables. Optimized unloaded formulation (UFM-11) gave average particle size 205.5 ± 2.9 nm and PDI 0.388 ± 0.008 (). Optimized NCS loaded formulation (NFM-3) gave Average particle size 204.2 ± 3.2 nm, PDI 0.328 ± 0.02, () and zeta potential −33.16 ± 2 mV (). NFM-3 formulation gave zeta potential −33.16 mV ±2.
Entrapment efficiency and drug loading capacity
Maximum EE (89.1 ± 0.03%) and DLC (2.87 ± 0.05) were observed for NFM-3 having drug lipid ratio (15:1) ().
Morphological studies
SEM image of NFM-3 formulation clearly showed that SLNs were of spherical shape and below 210 nm having smooth surface. SLNs were mostly dispersed but some aggregations were also present ().
Thermal analysis
DSC thermograms of unprocessed NCS (free drug), and processed NCS NFM-3 formulation were recorded separately. Endothermic peak was observed for unprocessed NCS at 229 °C while processed NCS (NFM-3 formulation) showed endothermic peak at 188.5 °C ().
Powder X-ray diffraction
Powder X-ray diffraction pattern showed sharp peaks both for NCS (pure drug) having larger peak counts at highest compared to optimized NFM-3 formulation (). The diffused peaks in P-XRD pattern of optimized NFM-3 formulation revealed its amorphous nature. Reduced average particle size, increased surface area and strong contact between Tween-80 and drug (NCS) could be considered for conversion to amorphous state.
Fourier transform infrared radiation measurements
Major peaks of NCS appeared at wave numbers 1572, 1515, 1613, 1285, 1650 and 1218 cm–1 [Citation33] ().
Stability study
No effective change was observed in size and PDI of NFM-3 formulation stored at refrigerated temperature. However, significant increase in size and PDI was observed for sample kept at room temperature (). Statistical data were analysed by two tailed t-test which showed p-value .03 for zeta-size and 0.05 for PDI.
Table 3. Observation during stability study of NCS-SLNs (NFM-3).
In vitro release of NCS from SLNS
During in vitro study, cumulative percent drug release from NFM-1 to NFM-5 formulations were 100%, 100%, 97.31%, 57.35% and 55.15%, respectively (). Kinetic study of drug release is very valuable in finding important parameters [Citation34]. Kinetic models study showed that NCS-SLNs followed zero-order kinetics ().
Table 4. R2 values from different kinetic models.
In vivo pharmacokinetic study
After oral dose, plasma concentration–time curves of NFM-3 formulation and marketed drug, i.e. marketed drug in rabbits are shown (). It was observed that at all-time points, plasma concentrations of NCS were significantly more in rabbits with NFM-3 formulation than for those treated with marketed drug.
Peak plasma concentration (Cmax) for marketed drug and NFM-3 formulation was 1.84 ± 0.3 μg ml−1 and 3.97 ± 0.3 μg ml−1, respectively. AUC0→24 for marketed drug was 1.51 μg h ml−1 whereas for NFM-3 was 16.74 μg h ml−1. NFM-3 formulation showed 2.15-fold increase in Cmax and 11.08-fold increase in AUC0→24 compared to marketed drug ().
Table 5. Pharmacokinetic parameters of Niclosamide loaded SLNs (NFM-3) and marketed drug.
Discussion
The process and experimental conditions both for unloaded SLNs and NCS loaded SLNs were optimized using micro-emulsion technique. The unloaded SLNs formulation (UFM-11; particle size, 205.5 nm ± 2.2 and PDI 0.388 ± 0.03) with formulation components including steric acid (1000 mg), PEG (400 mg), Tween-80 (1600 mg) and stirring time (15 min) was found the suitable formulation for loading of drug and further studies. As drug is loaded, the amount of free lipid decreased and hence size reduced [Citation35]. In addition, PDI was reduced and controlled by increasing stirring time [Citation36] ().
The optimized NFM-3 formulation produced NCS-SLNs with particle size 204.2 nm ±3.2, PDI 0.328 ± 0.02, zeta potential −33.16 mV ± 2, EE 84.4 ± 0.02% and DLC 5.27 ± 0.03%.
The PDI <0.5 and zeta potential in the range of ±30 demonstrate that the produced nano-dispersion would be stable in nature [Citation37]. For our prepared NCS-SLNs, both these values are within the range, which exhibit electrostatic stabilization and consequently there would not be an aggregation in NCS-SLNs which can potentially lead to prevent Ostawald ripening and particle growth [Citation38]. Moreover, concentration of the chosen excipients including steric acid (1.0 g), Tween-80 (1.6 g) and PEG (0.4 g) was found the effective combination to demonstrate maximum encapsulation of the drug with higher loading efficiency [Citation39]. It has been previously reported that in lipid and polymer based nanoparticles, the binding energy of the APIs with the lipids and polymers plays a key role to effectively encapsulate the drug in the lipid and polymer layers [Citation40]. In this case, it might be attributed to the high binding energy of the NCS with steric acid, Tween-80 and PEG which results in maximum drug loading.
Scanning electron microscopy further confirmed the size and morphology of NCS loaded nanoparticles. shows that the nanoparticles are spherical in shape with homogeneous distribution which exhibit amorphous nature of the produced nanoparticles. The FT-IR studies confirmed compatibility of the NCS with the formulation components, because there was no any observed considerable variation in FT-IR spectra of NCS free drug and NFM-3 formulation, revealed no interaction between NCS and SA.
Additionally, the DSC studies also confirmed the amorphous nature of the NCS loaded SLNs, because the sharp melting point peak for the unprocessed NCS which appeared on 229 °C disappeared for NCS-loaded SLNs. There was observed a tiny peak at 188.5 °C for processed NCS, which is because of reduced size of the NCS, enlarged surface area and closed contact between hydrophilic polymer (Tween-80) and drug (NCS) which could be considered for the change of NCS from crystalline to amorphous state. Also this shows the dispersion of the NCS in lipid layer where the melting point of the NCS shifted to lower melting point with disappearance of the peaks of other components. The shifting of the melting point peak of active pharmaceutical ingredients (API) in SLNs using steric acid and Tween-80 has previously been reported [Citation41].
P-XRD studies also confirmed the amorphous nature of the NCS loaded nanoparticles (). The disappearance and reduction in intensities of the XRD peaks are indicative for amorphous nature of the particles [Citation37,Citation42]. Some of the sharp peaks disappeared in diffractogram of the processed NCS compared to unprocessed NCS.
By increasing the quantity of NCS, EE% decreases while DLC% increases gradually. No significant change was observed in size and PDI of NFM-3 formulation at refrigerated temperature (5 ± 3 °C) after 90 days. Additionally, there was some observed growth in the samples stored at room temperature for the initial 30 days which is because of the amorphous nature of the NCS-SLNs followed by stabilization for rest of the period. This might be attributed to the dissolution of the small particles while depositing onto the surface of the large particles which is common in amorphous particles [Citation37,Citation42]. Kinetic study is very important in finding valuable parameters such as bioavailability [Citation34]. The release of NCS from SLNs was best-fitted in Peppa’s fitting kinetics and the possible mechanisms for the drug release might follow relaxation from lipids, i.e. Super Case-II transport [Citation43].
The interesting results obtained from pharmacokinetic study revealed that NCS absorption was significantly enhanced by taking up SLNs as drug delivery system. The small particle size of SLNs may have bio-adhesion with gastrointestinal wall or go through the inter-villar spaces therefore rising their residence duration in the gastrointestinal tract [Citation44,Citation45]. This increase in sticking with GIT will result in boosted bioavailability. Tween-80 may have paid to increase in affinity between SA and intestine which leads to enhance permeability [Citation46,Citation47].
Statistical analysis of in vivo pharmacokinetic parameters confirmed that SLNs improved the bioavailability of NCS significantly compared to marketed drug.
SLNs as drug delivery system open angles to formulate low water soluble drugs. SLNs are not only responsible for improvement of oral absorption but can correspondingly be formulated for parenteral administration, which need additional studies [Citation48].
So, it is concluded that NCS in form of SLNs is a promising Nano-medicine with stable and sustained release behaviour. BCS-II drugs like NCS can be successfully loaded in lipid such as SA. In vitro and in vivo release studies of NCS confirmed that this system is suitable to improve oral delivery of NCS with increased aqueous solubility, permeability and finally bioavailability.
Acknowledgements
The authors wish to thank research support provided by National Institute for Biotechnology and Genetic Engineering Faisalabad, Shaigan Pharmaceuticals (Pvt) Ltd Rawalpindi and Ferozsons Labs Nowshera, Pakistan.
Disclosure statement
The authors declare that the research was conducted without financial or commercial relationships that could be construed as a potential conflict of interest.
References
- Mahon E, Salvati A, Bombelli FB, et al. Designing the nanoparticle–biomolecule interface for “targeting and therapeutic delivery”. J Control Release. 2012;161:164–174.
- Ahad A, Al-Saleh AA, Al-Mohizea AM, et al. Formulation and characterization of novel soft lipid-based nanoparticles for enhanced transdermal delivery of eprosartan mesylate. Saudi Pharm J. 2017;25:1040–1046.
- Triplett IIMD, Rathman JF. Optimization of β-carotene loaded solid lipid nanoparticles preparation using a high shear homogenization technique. J Nanopart Res. 2009;11:601–614.
- Fulekar M. Nanotechnology: importance and applications. New Delhi: IK International Pvt Ltd; 2010.
- Natarajan J, Baskaran M, Humtsoe LC, et al. Enhanced brain targeting efficacy of Olanzapine through solid lipid nanoparticles. Artif Cells Nanomed Biotechnol. 2017;45:364–371.
- Patidar A, Thakur DS, Kumar P, et al. A review on novel lipid based nanocarriers. Int J Pharm Pharm Sci. 2010;2:30–35.
- Ebrahimi HA, Javadzadeh Y, Hamidi M, et al. Repaglinide-loaded solid lipid nanoparticles: effect of using different surfactants/stabilizers on physicochemical properties of nanoparticles. DARU J Pharm Sci. 2015;23:1.
- Pan J-X, Ding K, Wang C-Y. Niclosamide, an old antihelminthic agent, demonstrates antitumor activity by blocking multiple signaling pathways of cancer stem cells. Chin J Cancer. 2012;31:178.
- Al‐Hadiya BM. Niclosamide: comprehensive profile. Prof Drug Subst Excipients Relat Methodol. 2005;32:67–96.
- Wu Y-Q, Yang T-S, Li X, et al. Novel derivatives of niclosamide synthesis: its bioactivity and interaction with Schistosoma japonicum cercariae. Dyes Pigments. 2011;88:326–332.
- Weinbach EC, Garbus J. Mechanism of action of reagents that uncouple oxidative phosphorylation. Nature. 1969;221:1016–1018.
- Lin C-K, Bai M-Y, Hu T-M, et al. Preclinical evaluation of a nanoformulated antihelminthic, niclosamide, in ovarian cancer. Oncotarget. 2016;7:8993.
- Osada T, Chen M, Yang XY, et al. Antihelminth compound niclosamide downregulates Wnt signaling and elicits antitumor responses in tumors with activating APC mutations. Cancer Res. 2011;71:4172–4182.
- Whitesell JK. The Merck Index, CD-ROM (Macintosh): an encyclopedia of chemicals, drugs & biologicals. In: Budavari S, O'Neill M, Smith A, et al., editors. (Merck & Co., Inc.). New York: Chapman & Hall; 1997. $250.00. ISBN 0-412-75940-3. Washington, DC: ACS Publications; 1998.
- Chen H, Yang Z, Ding C, et al. Discovery of O-alkylamino-tethered niclosamide derivatives as potent and orally bioavailable anticancer agents. ACS Med Chem Lett. 2013;4:180–185.
- Rehman M, Asadullah Madni AI, Khan WS, et al. Solid and liquid lipid-based binary solid lipid nanoparticles of diacerein: in vitro evaluation of sustained release, simultaneous loading of gold nanoparticles, and potential thermoresponsive behavior. Int J Nanomed. 2015;10:2805.
- Ozturk B, Argin S, Ozilgen M, et al. Formation and stabilization of nanoemulsion-based vitamin E delivery systems using natural surfactants: quillaja saponin and lecithin. J Food Eng. 2014;142:57–63.
- Kim B-D, Na K, Choi H K. Preparation and characterization of solid lipid nanoparticles (SLN) made of cacao butter and curdlan. Eur J Pharm Sci. 2005;24:199–205.
- Abdelwahed W, Degobert G, Stainmesse S, et al. Freeze-drying of nanoparticles: formulation, process and storage considerations. Adv Drug Deliv Rev. 2006;58:1688–1713.
- Preet Kaur S, Rao R, Hussain A, et al. Preparation and characterization of rivastigmine loaded chitosan nanoparticles. J Pharm Sci Res. 2011;3:1227–1232.
- Dubes A, Parrot-Lopez H, Abdelwahed W, et al. Scanning electron microscopy and atomic force microscopy imaging of solid lipid nanoparticles derived from amphiphilic cyclodextrins. Eur J Pharm Biopharm. 2003;55:279–282.
- Zhang Y, Zhang J. Preparation of budesonide nanosuspensions for pulmonary delivery: characterization, in vitro release and in vivo lung distribution studies. Artif Cells Nanomed Biotechnol. 2016;44:285–289.
- Fang J-Y, Fang C-L, Liu C-H, et al. Lipid nanoparticles as vehicles for topical psoralen delivery: solid lipid nanoparticles (SLN) versus nanostructured lipid carriers (NLC). Eur J Pharm Biopharm. 2008;70:633–640.
- Shete AS, Yadav AV, Murthy SM. Chitosan and chitosan chlorhydrate based various approaches for enhancement of dissolution rate of carvedilol. DARU. 2012;20:93.
- Ebrahimi HA, Javadzadeh Y, Hamidi M, et al. Repaglinide-loaded solid lipid nanoparticles: effect of using different surfactants/stabilizers on physicochemical properties of nanoparticles. DARU J Pharm Sci. 2015;23:46.
- Griffiths PR, De Haseth JA. Fourier transform infrared spectrometry. Hoboken (NJ): John Wiley & Sons; 2007.
- Radomska-Soukharev A. Stability of lipid excipients in solid lipid nanoparticles. Adv Drug Deliv Rev. 2007;59:411–418.
- Bhardwaj U, Burgess DJ. A novel USP apparatus 4 based release testing method for dispersed systems. Int J Pharm. 2010;388:287–294.
- Daabees H. Selective differential spectrophotometric methods for determination of niclosamide and drotaverine hydrochloride. J Anal Lett. 2000;33:639–656.
- Zur Mühlen A, Schwarz C, Mehnert W. Solid lipid nanoparticles (SLN) for controlled drug delivery-drug release and release mechanism. Eur J Pharm Biopharm. 1998;45:149–155.
- Cholifah S, Farina Kartinasari W, Indrayanto G. Simultaneous HPLC determination of levamisole hydrochloride and anhydrous niclosamide in veterinary powders, and its validation. J Liq Chromatogr Relat Technol. 2007;31:281–291.
- Chiou WL. Critical evaluation of the potential error in pharmacokinetic studies of using the linear trapezoidal rule method for the calculation of the area under the plasma level-time curve. J Pharm Biopharmaceut. 1978;6:539–546.
- Van Tonder EC, Mahlatji MD, Malan SF, et al. Preparation and physicochemical characterization of 5 niclosamide solvates and 1 hemisolvate. AAPS PharmSciTech. 2004;5:86–95.
- Barzegar-Jalali M. Kinetic analysis of drug release from nanoparticles. J Pharm Pharm Sci. 2008;11:167–177.
- Kumar PP, Gayatri P, Sunil R, et al. Atorvastatin loaded solid lipid nanoparticles: formulation, optimization, and in vitro characterization. Int Organ Sci Res J Pharm. 2012;2:23–32.
- Baharifar H, Tavoosidana G, Karimi R, et al. Optimization of self-assembled chitosan/streptokinase nanoparticles and evaluation of their cytotoxicity and thrombolytic activity. J Nanosci Nanotechnol. 2015;15:10127–10133.
- Ali HS, York P, Ali AM, et al. Hydrocortisone nanosuspensions for ophthalmic delivery: a comparative study between microfluidic nanoprecipitation and wet milling. J Control Release. 2011;149:175–181.
- Liu J, Hu W, Chen H, et al. Isotretinoin-loaded solid lipid nanoparticles with skin targeting for topical delivery. Int J Pharm. 2007;328:191–195.
- Gunay MS, Ozer AY, Erdogan S, et al. Development of nanosized, pramipexole-encapsulated liposomes and niosomes for the treatment of Parkinson’s disease. J Nanosci Nanotechnol. 2017;17:5155–5167.
- Lee J. Drug nano- and microparticles processed into solid dosage forms: physical properties. J Pharm Sci. 2003;92:2057–2068.
- Farboud ES, Nasrollahi SA, Tabbakhi Z. Novel formulation and evaluation of a Q10-loaded solid lipid nanoparticle cream: in vitro and in vivo studies. Int J Nanomed. 2011;6:611.
- Khan S, Matas Md, Zhang J, et al. Nanocrystal preparation: low-energy precipitation method revisited. Cryst Growth Des. 2013;13:2766–2777.
- Costa P, Lobo JMS. Modeling and comparison of dissolution profiles. Eur J Pharm Sci. 2001;13:123–133.
- Duchêne D, Ponchel G. Bioadhesion of solid oral dosage forms, why and how? Eur J Pharm Biopharm. 1997;44:15–23.
- Vasir JK, Tambwekar K, Garg S. Bioadhesive microspheres as a controlled drug delivery system. Int J Pharm. 2003;255:13–32.
- Song K-H, Chung S-J, Shim C-K. Enhanced intestinal absorption of salmon calcitonin (sCT) from proliposomes containing bile salts. J Control Release. 2005;106:298–308.
- Venkatesan N, Uchino K, Amagase K, et al. Gastro-intestinal patch system for the delivery of erythropoietin. J Control Release. 2006;111:19–26.
- Yang SC, Lu LF, Cai Y, et al. Body distribution in mice of intravenously injected camptothecin solid lipid nanoparticles and targeting effect on brain. J Control Release. 1999;59:299–307.