Abstract
Earlier studies have clarified that NADH and NADPH, re-energized repeatedly by red blood cell (RBC) glycolysis, can be used in extracellular chemical reactions, where electron energies are extracted by electron mediators, such as methylene blue (MB). The electron mediators, which are reduced by NAD(P)H, permeate both the membranes of RBC and phospholipid bilayer of liposomes encapsulating haemoglobin (Hb-vesicles, HbV) and reduce autoxidized ferric methemoglobin (metHb) in HbV to ferrous Hb. Moreover, in vitro screening study clarified some other potential electron mediators with comparable capacity to reduce metHb. Given this background, eight of these compounds: MB, 1,9-dimethyl MB, azure A, azure B (AB), azure, toluidine blue, brilliant cresyl blue and toluylene blue, were evaluated in both in vitro and in vivo studies in this work. Compared with MB as a reference, in vitro experiments demonstrated that most compounds caused effective metHb reduction of HbV in the presence of RBC. However, in vivo experiments of bolus injection of autoxidized HbV to rats (10 mL HbV/kg body weight) followed by injection of the dye (1.53 mL/kg body weight, 2.6 mM) led to some differences from in vitro results. Effective metHb reduction was found for the combination of AB. To evaluate AB effectiveness further, a haemorrhagic shock and resuscitation model was used, where the rats were resuscitated with HbV. When the level of metHb increased to 50%, a dye solution was injected. Again, AB caused sufficient reduction of metHb. Through these in vivo experiments, this study clarified that AB is a suitable electron mediator to prolong the functional lifetime of HbV.
Graphical Abstract
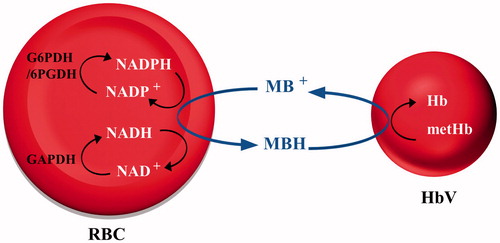
Introduction
The present blood donation system cannot always sufficiently supply healthcare facilities with enough safe blood for transfusion. Donated blood has a short shelf-life. Moreover, its use entails various complicated, time-consuming and expensive blood tests, creating persistent conditions of blood supply shortage. Alternative blood substitutes such as haemoglobin-based oxygen carriers (HBOCs) are believed to have the capacity to ease blood supply shortages and alleviate many blood transfusion-related issues [Citation1–6]. Actually, HBOCs can be produced in a way that eliminates viral infections such as HIV and hepatitis. Furthermore, these oxygen carriers can be modified to be ubiquitously applicable for all blood types. Attributes such as longer shelf-life can be achieved [Citation7,Citation8]. Haemoglobin-vesicles (HbVs) are a type of HBOC in which a purified and concentrated Hb solution is encapsulated with phospholipid bilayer membranes (liposomes). Earlier in vivo studies have clarified the oxygen carrying capacity, safety and efficacy of HbV as a transfusion alternative [Citation9,Citation10]. One remaining issue of HbV is autoxidation of the encapsulated ferrous HbO2 to form ferric methemoglobin (metHb) that does not bind oxygen. MetHb formation shortens the functional lifetime of HbV after injection into the bloodstream.
In red blood cells (RBCs), ferric metHb is mainly reduced by NADH-cytochrome b5 reductase via cytochrome b5, NADPH metHb reductase and NADPH-flavin reductase. According to a simulation study of metHb reduction, the NADPH-flavin pathway is used under normal physiological conditions where the oxidative stress is low and metHb levels are low. Conversely, the NADH-cytochrome b5 pathway plays a major role when oxidative stress is high [Citation11]. These electron-energy-rich molecules are re-energized repeatedly during the glycolysis of RBCs. Glucose, the main energy source for the cells, is metabolized through glycolysis and the hexose monophosphate shunt (HMP), also known as the pentose phosphate pathway [Citation12,Citation13]. In the presence of metHb, the electron-energy-rich molecule NADH produced in the Embden–Meyerhof pathway can be a resource to reduce metHb by NADP-cytochrome b5 to its functional form. The HMP shunt, the only source for NADPH, is generated by reduction of NADP+ [Citation11,Citation14].
For the treatment of methemoglobinemia, where the above-described enzymes malfunction or with an accidental poisoning, 1–2 mg/kg methylene blue (MB) is usually injected [Citation15]. The water-soluble tricyclic phenothiazine reacts with metHb in the presence of NADH and NADPH in RBCs. These electron carriers reduce the thiazine dye to leucomethylene blue (MBH), which in turn reduces metHb, resulting in HbO2 formation. This knowledge has been used for reduction of metHb encapsulated in HbV. Because the reduced MBH has a neutral charge and since it has a hydrophobic character, this molecule can readily diffuse through the phospholipid bilayer of both RBCs and HbVs. MBH entering the HbV functions as an electron mediator between NADH/NADPH in RBCs and metHb compartmentalized inside the vesicles [Citation16]. Earlier studies have verified that other cationic organic dyes (phenothiazines, phenazines, phenoxazines, indamine) are also useful in such redox reactions, and are thereby able to participate in the reduction of metHb [Citation17]. The present study was undertaken to clarify whether the suggested potential electron mediators (Scheme 1) can prolong the functional lifetime of HbVs, as evaluated by in vivo studies.
Materials and methods
Preparation of haemoglobin vesicles
The HbV suspension used for this study was prepared according to earlier reported procedures [Citation16]. Outdated human blood was provided by the Japanese Red Cross Society (Tokyo, Japan), from which Hb was purified. The liposome consists of 1,2-dipalmitoyl-sn-glycero-3-phosphatidylcholine, cholesterol, 1,5-O-dihexadecyl-N-succinyl-L-glutamate (DHSG; Nippon Fine Chemical Co. Ltd., Osaka, Japan) and 1,2-distearoyl-sn-glycerol-3-phosphatidylethanolamine-N-PEG5000 (NOF Corp., Tokyo, Japan) at a molar composition of 5/4/0.9/0.03 [Citation11]. The HbV is suspended in physiological saline solution at [Hb] = 10 g/dL.
HbV suspension with an internal level of metHb at 50–70% was achieved by favouring auto-oxidation through incubation at 37 °C in a water bath. To measure the level of metHb, 10 μL HbV solution was diluted in 4 ml phosphate buffered saline (PBS, pH 7.4; Gibco Life Technologies, Paisley, UK) in a Thunberg cuvette, which was then deoxygenated by bubbling with N2 for 10 min. The metHb level was estimated from the ratio of absorptions for deoxyHb (λmax = 430 nm) and metHb (λmax = 406 nm). All absorption measurements were taken using a UV–vis spectrophotometer (V-650; Jasco Corp., Tokyo, Japan) with an integrated sphere (ISN-40).
Preparation of washed rat red blood cells
Blood volume of 10–12 ml was withdrawn from a Wistar rat under anaesthesia, using syringes with heparin (Heparine 10,000 IU/mL; Mochida Pharmaceutical Co. Ltd., Tokyo, Japan). The blood sample was then centrifuged at 2000 rpm (himac CF12RX; Hitachi Koki Co. Ltd., Tokyo, Japan) for 5 min. After blood plasma had been removed carefully, 3 ml PBS was added; the RBCs were re-suspended. This procedure was repeated three times. The RBC concentrate was adjusted to 12 g Hb/dL by the addition of PBS to simulate the original Hb concentration of fresh blood. The RBC suspension was used within 24 h.
Preparation of dye solutions
MB has been clinically approved in Japan. Therefore, the dye solutions were prepared according to the instruction manual (methylene blue intravenous injection; Daiichi Sankyo Co. Ltd., Tokyo, Japan). Dyes were prepared by solubilizing the dye powder in a mixture of water and 5% glucose (Glucose 5%; Otsuka Pharmaceutical Co. Ltd., Tokyo, Japan) with the volume ratio 1:5. The solutions were filtered with a 0.20 μm-pore-sized filter (DISMIC-25CS; Toyo Roshi Kaisha Ltd., Tokyo, Japan). The final concentration of the dye solution was 2.6 mM. Dye solutions were stored in a dark place at room temperature. In all, eight dyes were prepared (Scheme 1): MB, 1,9-dimethyl methylene blue (DMB), azure A (AA), azure B (AB), azure (AC), toluidine blue (TDB), brilliant cresyl blue (BCB), and toluylene blue (TLB). AA, AC, BCB, and TLB were purchased from MP Biomedicals, LLC. (Illkirch, France). AB was purchased from Wako Pure Chemical Industries Ltd., Japan. DMB was from Polysciences Inc. (Warrington, FL). MB was from Sigma-Aldrich (St. Louis, MO), and TDB was from Waldeck GmbH & Co. KG (Münster, Germany).
In vitro reduction of encapsulated MetHb
An 80-min assay was used to examine the metHb-reducing potential of the different dyes. RBC suspension, HbV containing 50–70% metHb, 2.6 mM dye solution and 5% glucose solution were mixed at the volume ratio of 40:20:2:5. Glucose is necessary for the RBCs to provide electron energies generated in the glycolysis. Addition of glucose will, therefore, provide comparable conditions for all the experiments conducted. The amount of glucose and washed RBCs were sufficient to reduce metHb immediately after mixing and cancel the different initial metHb levels.
To imitate physiological conditions the RBC, HbV suspension and glucose solution were mixed and incubated in a water bath at 37 °C for 5 min. The dye solution was then added to the mixture, which was incubated for additional 5 min. Thereafter, the first sample was collected in heparinized hematocrit (Hct) glass capillaries (Hirshmann Laborgerate GmbH & Co., Germany). As the experiment continued, one sample was collected every 15 min. HbVs were separated from RBCs by centrifuging (micro hematocrit centrifuge 3220; Kubota Corp., Tokyo, Japan) the capillaries at 12,000 rpm for 5 min. The obtained upper HbV layer was diluted with 4 ml PBS in a Thunberg cuvette and was bubbled with N2 for 10 min to ascertain the level of metHb as described above.
In vivo reduction of encapsulated MetHb in topload infusion model
Experimental procedures on animals were approved by the ethical committee of animal experiments, Nara Medical University. In all, 36 male Wistar rats with body weight of 334 ± 21 g were used. The rat was anaesthetized using a vaporizer (MK-A110; Muromachi Kikai, Co. Ltd.) with an anaesthetic gas scavenging system (MK-T100E; Muromachi Kikai Co. Ltd.), providing the animal with inhalation of 1.0% isoflurane (Pfizer Inc., Tokyo, Japan) mixed with air at a flow rate of 1 ml/min. Spontaneous breathing was maintained throughout the experiment, enduring for approximately 6 h. To the left femoral artery, a polyethylene catheter (SP-31 tubing, I.D. 0.5 mm, O.D. 0.8 mm; Natsume Seisakusho Co., Ltd., Tokyo, Japan) containing saline with a concentration of 40 IU/mL heparin was cannulated.
Reports of earlier studies have described the systemic whole blood volume as 56 ml/kg body weight. As a safety measure, the HbV suspension was filtrated in advance using a 5 μm filter (Minisart; Sartorius Stedim Biotech GmbH, Goettingen, Germany). The rat was injected with HbV at the dose rate of 10 ml/kg body weight at a 1 ml/min flow rate. HbV comprised 50% metHb. After 5 min, 2.6 mM of the dye solution was injected at the dose rate of 1.53 ml dye/kg body weight. An earlier study confirmed that an increased dose of MB solution (2.6 mM) in the range 0.506–1.53 ml/kg body weight caused the most effective reduction of metHb [Citation16]. This dosage is below the recommended maximum dosage for MB (2 mg/kg, which is approximately 2.4 ml dye/kg rat [2.6 mM]) for injection in treatment of methemoglobinemia [Citation15]. A blood sample of about 70 μL was collected from the artery at every time point into heparinized Hct glass capillaries and was centrifuged for 1 min at 12,000 rpm. Thereafter 20 μL of the plasma phase was collected and diluted with 4 ml of PBS in a Thunberg cuvette, sealed with a rubber cap, and bubbled with N2 gas for 10 min. The level of metHb was estimated using the spectroscopic method described above.
Haemorrhagic shock and resuscitation model
To conduct the haemorrhagic shock model, 23 male Wistar rats with body weight of 312 ± 22 g were used. The rats were prepared as described in the previous section. Spontaneous breathing and anaesthesia were maintained throughout the experiment, enduring for approximately 10 h for the one-day experiment. For the two-day experiment, topload study, spontaneous breathing was maintained for 9 h on day 1 and 7 h on day 2. The rats were un-anaesthetized overnight for 15 h, and kept in a temperature-controlled clean room with 12 h dark-/light cycle. Rats were provided food and water ad libitum inside their cages.
To induce haemorrhagic shock, half of the blood volume, 28 ml/kg, was withdrawn from the femoral artery and the rat was left hypotensive for 15 min [Citation10]. An equal volume to that of the withdrawn blood was injected (28 ml/kg), with the resuscitative fluid consisting of a suspension HbV and human serum albumin (HSA) mixture (albumin 25% I.V. 5 g/20 ml BENESIS) at a mixing ratio of 1.4 ml HSA to 8.6 ml HbV.
In the one-day experiment, a dye was injected 6 h after resuscitation. The dye injection amounts used to investigate the efficiency were 0.756, 1.0 and 2.4 ml/kg [2.6 mM]. Blood lactate levels were monitored electrochemically with Lactate Pro (Arkray Inc., Kyoto, Japan). In the two-day experiment, a dye solution of electron mediator (1.53 and 2.4 ml/kg) was injected 8 h after resuscitation with HbV. A second injection was done 15 h later. Blood samples were collected from the artery with regular intervals in heparinized Hct glass capillaries for metHb measurement. All rats survived the invasive surgical intervention, haemorrhagic shock, and resuscitation until the planned killing of the rats.
Statistical analysis
For statistical analyses, software (Prism 6 GraphPad, San Diego, CA) was used to calculate significant differences between the different electron mediators at each used time point. Calculations were performed using a two-way ANOVA (fixed effects) with repeated measures for which significance was determined by p < .05, n = 3. In vitro and in vivo data are presented as mean values for each electron mediator, with their respective standard deviations at different time points.
Results
In vitro reduction of encapsulated MetHb
The efficacies of the dyes as electron mediators from NAD(P)H in RBC to metHb in HbV were initially evaluated and compared using an in vitro experiment. This was achieved by incubating each dye with a mixture of RBC, partially oxidized HbV, and glucose solution for 80 min at 37 °C (). All dyes proved to be effective for metHb reduction. Using MB as a reference, the reduction mediated by AA, AB, and BCB caused lower metHb levels with a consistently significant difference (p < .05, n = 3) enduring between 5 and 65 min of the assay. However, despite non-significant improvement compared to MB of the other dyes, all dyes were regarded as potential candidates worth to scrutinize in the reduction reaction of encapsulated metHb in the following in vivo experiments.
Figure 1. Reduction of metHb encapsulated in HbVs studied in vitro over 80 min. RBC suspension, HbV containing 50% metHb, 2.6 mM dye solution, and 5% glucose solution were mixed at the volume ratio of 40:20:2:5 and were incubated at 37 °C. All dyes showed metHb reduction to a level below 25% metHb. The level was steady over the time course of 80 min. The dashed lines represent 25% metHb. Plotted data are mean ± standard deviation (n = 3). Each dye is displayed separately with the other dyes in the background for comparison. *p < .05; #p < .01; ‡p < .0001 vs. MB.
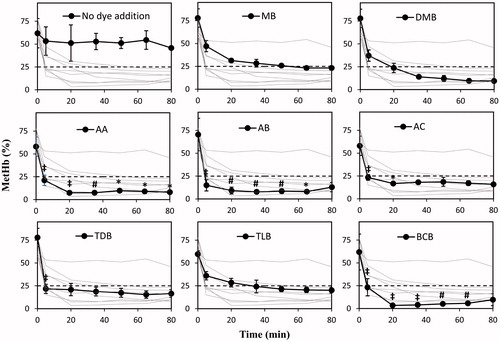
In vivo reduction of encapsulated MetHb
Anesthetized healthy male Wistar rats received HbV with about 50% metHb level; then they received a dye solution. An earlier study confirmed that the reduction efficiency of MB increased with a larger injection amount in the range of 0.42–1.27 mg/kg body weight [Citation16]. Assuming that the other dyes show similar profiles, the injection amount was fixed to 1.27 mg MB/kg body weight in this study, which is equal to approximately 4 μmol/kg body weight. Dye solutions were prepared in 2.6 mM concentrations. Thereby, the injection amount for each dye was calculated to 1.53 ml/kg body weight. Injection of all dyes except DMB showed promising reduction of ferric metHb of HbV (), decreasing the percentage from approximately 50% metHb to less than 25% within 1 h. The experiment continued for 6 h while the drop displayed in the profiles sustained for a maximum time of 1 h. Thereafter, regeneration of metHb is visualized by the growing profiles. Especially, significantly lower metHb levels were found for AB and AC than those of MB, especially at the latter time points.
Figure 2. In vivo reduction of encapsulated metHb in HbV. Wistar rats received injection of 10 ml HbV/kg with an initial metHb level around 50%, followed by 1.53 ml dye/kg at a concentration of 2.6 mM. Plotted values are mean ± standard deviation (n = 3). Each dye is displayed separately with the other dyes in the background for comparison. *p < .05; #p < .01; ‡p < .0001 vs. MB.
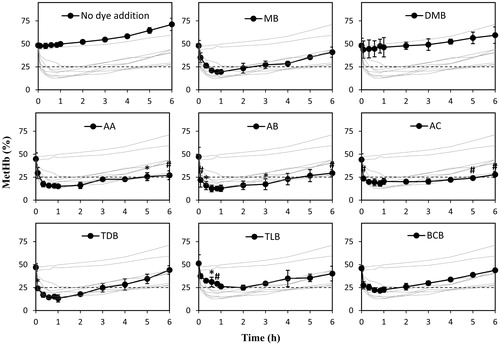
After the experiments rats were killed by incision of abdomen and exsanguination, it was observed that the bladder in all rats was darkly discoloured. Excretion of the dyes through urination was noticed in all trials, except in rats that had been injected with DMB.
Single injection of electron mediator following resuscitation from haemorrhagic shock
A haemorrhagic shock and resuscitation model was constructed to elucidate the electron mediating capacities of MB, AB, and AC in acute situations. Haemorrhagic shock was induced by withdrawal of 50% of the total blood volume in Wistar rats. The rat was resuscitated with HbV-albumin suspension 15 min after withdrawal; 6 h later, the electron mediator (2.6 mM) was injected at the dose rate of either 1.53 ml/kg () or 1.0 ml/kg (). Consequently, the metHb drops were visible. As shows, AB showed significantly lower metHb levels (p < .05, n = 3) than those of MB in the first 45 min after dye injection.
Figure 3. Reduction of metHb by injection of the dye solutions (MB, AB or AC) after resuscitation with HbV from haemorrhagic shock. The dye concentration is 2.6 mM. The injection volume is 1.53 ml/kg. Plotted values are mean ± standard deviation (n = 3). *p < .05; #p < .01 vs. MB.
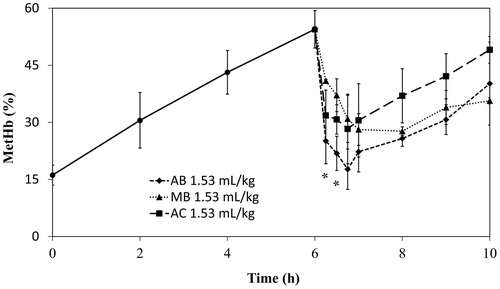
Figure 4. Reduction of metHb by injection of dye solutions (MB or AB) after resuscitation with HbV from haemorrhagic shock. The injection volumes of AB and MB were lower: 1.0 ml/kg [2.6 mM]. Plots show data as mean ± standard deviation (n = 3). *p < .05 vs. MB.
![Figure 4. Reduction of metHb by injection of dye solutions (MB or AB) after resuscitation with HbV from haemorrhagic shock. The injection volumes of AB and MB were lower: 1.0 ml/kg [2.6 mM]. Plots show data as mean ± standard deviation (n = 3). *p < .05 vs. MB.](/cms/asset/db5e8932-f419-4de2-873d-78e2c1861810/ianb_a_1397003_f0004_b.jpg)
The efficacy of metHb reduction did not change as much with the injection volume for AB ( and ), although a greater injection volume of MB seemed to improve reduction of metHb in HbV. The metHb level obtained with both concentrations of AB was significantly lower than the results obtained with MB from the injection of the dye and 45 min into the reduction. The reduction obtained with AB was better, however, the reoxidation of AB occurred more rapidly than that of MB, resulting in rapid elevation of metHb.
Withdrawal of half of the blood volume induced haemorrhagic shock, resulting in a rapid increase of the lactate level to nearly 10 mM because of anaerobic metabolism in anoxia. Nevertheless, the level returned to around 1.5 mM after resuscitation. It stabilized until injection of dye at time point 6 h, after which a slight decrease of lactate concentration was observed, indicating the improved oxygen supply and aerobic metabolism ().
Repeated injection of electron mediators
Experiments were conducted to investigate whether a repeated reduction is mediated or not, by repeated injection of AB or MB (2.4 and 1.53 ml/kg). Both substances could mediate the reduction of metHb repeatedly after the second injection ( and ). All rats survived the 30 h experiment until planned death.
Discussion
The main findings of this study are that, among several cationic organic dyes tested, AB a derivate from MB, showed significant reduction of metHb encapsulated by phospholipid bilayer membranes, HbVs. Results demonstrated that AB might be used in combination with HbVs to prolong their functional lifetime. The electron pathway of MB for the reduction of metHb compartmentalized inside the vesicle has been clarified by earlier studies [Citation11]. In the present investigation, eight dyes were evaluated: MB, DMB, TDB, AA, AB, AC, TLB and BCB. Because of similarity in molecular structure and physiochemical properties, such as reaction rates with NADH/NADPH, incorporation rate into HbVs and RBC, reoxidation rate and redox potential, the electron pathway is presumably similar to that of MB, as shown in [Citation17]. Because MB has been approved by the FDA as an antidote for methemoglobinemia [Citation18], potential substances have been compared with MB to evaluate their efficacy. Results from both in vitro and in vivo studies support results described in an earlier report, which clarified that several different cationic organic dyes can contribute to similar electron-mediating properties as MB and thereby can reduce metHb [Citation17].
Table 1. Summary of the chemical properties of eight dye molecules as an electron mediator from RBC to metHb in HbV.
Despite these similarities among organic dyes, the electron mediating properties vary in vivo. This variation is attributable to differences in the in vivo biodistribution and metabolism of the substances. Differences observed by comparing the results obtained from in vitro () and in vivo () studies indicate that physiological conditions are difficult to re-create. Therefore, in vivo studies must be conducted to elucidate the metHb reduction.
Results of the in vitro investigation conducted in this study showed that most dyes caused moderate reduction of metHb. However, DMB produced an unexpectedly low effect in vivo (). Examination of the chemical properties of the dye DMB reveals higher reaction rates with both NADH and NADPH, than those of MB. Furthermore, the re-oxidation rate was lower. The incorporation in both HbVs and RBCs was higher. Consequently, DMB possesses desirable properties for reducing MetHb in our system. However, the unexpected tendency of DMB obtained from the in vivo study might be attributable to an interaction with glycosaminoglycans (GAGs), which are sulphated polysaccharides found in animal tissues, such as arterial walls, heart valves, lung and liver [Citation19,Citation20]. Inspection of the molecular structure of the dye reveals that it has less ability of forming hydrogen bonds than the other dyes. Consequently, it is expected to have a higher tendency to become incorporated into lipophilic surfaces, which suggests that the injected DMB molecules might diffuse to the tissues and form complexes with GAG molecules. In addition, when using DMB precipitation was observed in the polyethylene tube, connected to the femoral artery, containing heparinized saline. Heparin is used as an anticoagulant in the experiments. Heparin, which is a GAG molecule [Citation19–21], most likely forms complexes with the DMB molecules thereby causing the observed precipitation in the tube. Precipitation is generally expected to reduce the efficiency of the dye because of the loss in the ability to diffuse across the membranes of both HbV and RBCs. Heparin, a polyanionic polysaccharide, may interfere not only with DMB but possibly also with other cationic dyes [Citation22]. In future studies it will be essential to examine possible interactions between heparin and the dyes.
BCB and TDB showed effective reduction in the 80-min in vitro assay. However, when tested for 6 h in vivo, the re-oxidation rate of metHb was high. BCB shows the highest rate of re-oxidation with O2 as shown in , which indicates relatively larger production of reactive oxygen species (ROS) by re-oxidation of the reduced dyes and the resulting higher level of metHb formation. During treatment of methemoglobinemia, patients are injected with a maximum 2 mg MB/kg body weight [Citation15]. For appropriation to clinical studies, the injection amount was chosen to be within this range or lower, to consider possible toxicity effects. As reported by other researchers, phenothiazines have low toxicity in mammals [Citation23,Citation24], but further investigations are necessary. For instance, MB and its metabolites have been demonstrated to bind to DNA and act as inhibitors to cell growth [Citation25]. Adverse effects such as haemolytic action, accelerated methemoglobinemia, reactions to a rapid injection, and contraindications are described in detail by Bradberry [Citation15]. Further research must be conducted to obtain more information related to dye toxicity.
From the in vivo evaluations described here, it appears that the metabolites of MB (AA, AB and AC) mediate in the best reduction of metHb. These metabolites are produced by N-demethylation of MB, where AB is the main and AA is the secondary metabolite [Citation26]. The conducted experiments caused a significantly better reduction of metHb with AB compared with MB. According to previous in vitro-based study, AB has a lower re-oxidation rate than that of MB [Citation12]. A lower re-oxidation rate is a desirable property because it induces a lower risk of harmful reactions with ROS, which in turn induces autoxidation of Hb. Reportedly, AB has pharmacological effects but these have not been well studied [Citation27]. It is generally assumed that AB possesses similar low-toxicological effects as MB, which would make it suitable for application to pharmaceutical development. Several researchers have suggested that the effect observed by MB in humans is attributable to the main metabolite AB. One study clarified that AB is a better monoamine oxidase inhibitor than MB [Citation28]. Another study clarified reduction of mortality from endotoxic shock [Citation29].
As a result of haemorrhage, an increase of lactate concentration to about 10 mM in the blood is noted (data not shown). Loss of large blood volumes induces anaerobic metabolism because of reduced tissue perfusion of oxygen, resulting in a drastic increase of lactate concentration. However, after resuscitation with the HbV-HSA suspension, the lactate level was restored extensively [Citation10]. Moreover, it is noteworthy that the lactate level tended to decrease slightly after injection of an electron mediator 6 h after resuscitation (). Restored lactate values indicate that the HbV-HSA suspension can increase the oxygen supply. The reduced lactate levels after dye injection indicate that the dye further improved the aerobic metabolism by the reduction of metHb in HbV.
The difference between AB and MB in molecular structure is that AB carries a monomethyl-amino group (-NH-CH3) whereas MB harbours a dimethyl-amino group (-N-(CH3)2) at the same position. The -NH-CH3 groups of both AB and leucoAB (reduced AB) can be deprotonated to form a neutral quinoneimine. MB lacks this capacity. Consequently, the formation of neutral quinoneimine might explain the effectiveness of AB. Because of the high pKa value (12.1) of AB, it is almost exclusively found in its protonated form under physiological conditions [Citation28]. The quinoneimine is more lipophilic, thereby diffusing easily through biological membranes. According to Wart et al. [Citation30], peripheral organs of a patient who had received MB showed high levels of AB in the tissues at autopsy: approximately six-fold higher than MB. The concentration of AA in the tissues was between the values of MB and AB, although the concentration of the third metabolite AC was extremely low [Citation30]. The re-oxidation slope after injection of AB ( and ) is similar to the slope before dye injection, which suggests that AB is removed quickly from the blood.
The diffusion of AB into the tissues and elimination of the dye through urine might be the reason for the shorter reduction mediated by AB in the haemorrhagic shock and resuscitation model ( and ). However, repeated injections can be done to prolong the functional lifetime of HbVs further ( and ). Overall, the main metabolite of MB, AB, seems to show promising properties. It has markedly faster metHb reduction immediately after injection. As observed in and , the dose response for AB does not change much compared to MB, thereby it might be possible to reduce the dosage.
Conclusions
We investigated the electron mediating capabilities of eight cationic organic dyes for the reduction of metHb in HbV, both in vitro and in vivo. In addition to MB, which is used clinically for the reduction of methemoglobinemia, the potentials of other biological dyes for this effect were clarified as better electron mediators to prolong the functional lifetime of HbVs in vivo. Of the eight different dyes studied, results clearly indicated that all caused effective metHb reduction of HbVs in the presence of RBCs in vitro. However, in vivo experiments showed some discrepancies in which DMB specifically gave high-reducing effect in an in vitro experiment, but lost its electron-mediating power because of complex formation with GAG molecules in vivo. The study clarified through in vivo experiments that AB is a suitable electron mediator towards prolonging the functional lifetime of HbV and implies its potential in the treatment of methemoglobinemia.
Further investigations are necessary to optimize the metHb-reducing capacity of AB by injecting smaller volumes at more frequent time intervals. Furthermore, the toxicity and safety of repeated injection of AB must be confirmed. Finally, the effect of conventional MB-based treatment for methemoglobinemia should be compared with alternatives based on, e.g. AB.
Acknowledgements
The authors acknowledge Tomoko Kure, Takashi Matsuhira and Keizo Yamamoto (Nara Medical University), and Karin Kettisen (Lund University) for their assistance with experiments and suggestions.
Disclosure statement
The authors declare the following competing financial interests: H.S. is an inventor holding some patents related to the production and utilization of Hb-vesicles.
Funding
This research was supported partly by Grants-in-Aid for Scientific Research (Kiban B, 17H02087) from the Japan Society for the Promotion of Sciences (JSPS) and by the Project Promoting Clinical Trials for Development of New Drugs and Medical Devices (16lk0201034h0002) from the Japan Agency for Medical Research and Development: AMED. Semhar Ghirmai was recipient of scholarships from the Scandinavia − Japan Sasakawa Foundation (GA17-SWE-0125).
References
- Chang TM. From artificial red blood cells, oxygen carriers, and oxygen therapeutics to artificial cells, nanomedicine, and beyond. Artif Cells Blood Substit Immobil Biotechnol. 2012;40:197–199.
- Bian YZ, Guo C, Chang TM. Temperature stability of poly-[hemoglobin-superoxide dismutase-catalase-carbonic anhydrase] in the form of a solution or in the lyophilized form during storage at −80 °C, 4 °C, 25 °C and 37 °C or pasteurization at 70 °C. Artif Cells Nanomed Biotechnol. 2016;44:41–47.
- Wollocko H, Wollocko BM, Wollocko J, et al. OxyVita®C, a next-generation haemoglobin-based oxygen carrier, with coagulation capacity (OVCCC). Modified lyophilization/spray-drying process: proteins protection. Artif Cells Nanomed Biotechnol. 2017;45:1350–1355.
- Qi D, Li Q, Wang P, et al. Haemoglobin site-specifically modified with ferulic acid to suppress the autoxidation. Artif Cells Nanomed Biotechnol. 2017;45:1077–1081.
- Terraneo L, Bianciardi P, Malavalli A, et al. Hemoglobin extravasation in the brain of rats exchange-transfused with hemoglobin-based oxygen carriers. Artif Cells Nanomed Biotechnol. 2017;45:710–716.
- Ao-ieong ESY, Williams A, Jani V, et al. Cardiac function during resuscitation from hemorrhagic shock with polymerized bovine hemoglobin-based oxygen therapeutic. Artif Cells Nanomed Biotechnol. 2017;45:686–693.
- Winslow RM. Blood substitutes. London: Academic Press; 2006.
- Chang TMS. Blood substitutes: principles, methods, products and clinical trials. Tissue Eng. 1. Basel: S. Karger AG; 1997.
- Sakai H. Overview of potential clinical application of hemoglobin vesicles (HbV) as artificial red cells, evidenced by preclinical studies of the academic research consortium. J Funct Biomater. 2017;8:E10.
- Sakai H, Seishi Y, Takeoka S, et al. Fluid resuscitation with artificial oxygen carriers in hemorrhaged rats: profiles of hemoglobin-vesicle degradation and hematopoiesis for 14 days. Shock. 2009;2:192–200.
- Kinoshita A, Nakayama Y, Kitayama T, et al. Simulation study of methemoglobin reduction in erythrocytes. Differential contributions of two pathways to tolerance to oxidative stress. FEBS J. 2007;274:1449–1458.
- Nagai M, Yubisui T, Yoneyama Y. Enzymatic reduction of hemoglobins M Milwakukee-1 and M Saskatoon by NADH-cytochrome b5 reductase and NADPH-flavin reductase purified from human erythrocytes. J Biol Chem. 1979;255:4599–4601.
- Kennett EC, Ogawa E, Agar NS, et al. Investigation of methaemoglobin reduction by extracellular NADH in mammalian erythrocytes. Int J Biochem Cell Biol. 2005;37:1438–1445.
- Yubisui T, Matsuki T, Takeshita M, et al. Characterization of the purified NADH-Flavin reductase of human erythrocytes. J Biochem. 1979;85:719–728.
- Bradberry SM. Occupational methaemoglobinaemia – mechanisms of production, features, diagnosis and management including the use of methylene blue. Toxicol Rev. 2003;22:13–27.
- Sakai H, Li B, Lim W, et al. Red blood cells donate electrons to methylene blue mediated chemical reduction of methemoglobin compartmentalized in liposomes in blood. Bioconjugate Chem. 2014;25:1301–1310.
- Kettisen K, Bülow L, Sakai H. Potential electron mediators to extract electron energies of RBC glycolysis for prolonged in vivo functional lifetime of hemoglobin vesicles. Bioconjugate Chem. 2015;26:746–754.
- U.S. Food and Drug Administration. U.S. Food and Drug Administration. [Online]; 2016 [cited 2017 May 13]. Available from: https://www.accessdata.fda.gov/drugsatfda_docs/label/2016/204630s000lbl.pdf.
- Barbucci R, Magnani A, Lamponi S, et al. Chemistry and biology of glycosaminoglycans in blood coagulation. Polym Adv Technol. 1996;7:675–685.
- Stone JE, Akhtar N, Botchway S, et al. Interaction of 1,9-dimethylmethylene blue with glycosaminoglycans. Ann Clin Biochem. 1994;31:147–152.
- Farndale WR, Buttle DJ, Barrett AJ. Improved quantitation and discrimination of sulphated glycosaminoglycans by use of dimethylmethylene blue. Biochim Biophys Acta. 1986;883:173–177.
- Jakubowska M, Adamus J, Gebicki J, et al. Pulse radiolysis and spectrophotometric studies on the binding of organic cations with heparin. Radiat Phys Chem. 2014;99:6–11.
- Warinwright M. Methylene blue derivatives – suitable photoantimicrobial blood product disinfection? Int J Antimicrob Agents. 2000;16:381–394.
- Sonobe T, Haouzi P. H2S induced coma and cardiogenic shock in the rat: effects of phenothiazinium chromophores. Clin Toxicol. (Phila). 2015;53:525–539.
- Atamna H, Krugliak M, Shalmiev G, et al. Mode of antimalarial effect of methylene blue and some of its analogues on Plasmodium falciparum in culture and their inhibition of P. vinckei petteri and P. yoelii nigeriensis in vivo. Biochem Pharmacol. 1996;51:693–700.
- Ferreira-Leitão VS, Godinho da Silva J, Bon EPS. Methylene blue and azure B oxidation by horseradish peroxidase. Appl Catalysis B. 2003;42:213–221.
- Schirmera RH, Adlera H, Pickhardt M, et al. Lest we forget you – methylene blue. Neurobiol Aging. 2011;32:2325.e7–2e16.
- Petzer A, Harvey BH, Wegener G, et al. Azure B, a metabilite of metylene blue, is a high-potency, reversible inhibitor of monoamine oxidase. Toxicol Appl Pharm. 2012;258:403–409.
- Culo F, Sabolovic D, Somogyi L, et al. Anti-tumoral and anti-inflammatory effects of biological stains. Agents Actions. 1991;34:424–428.
- Wart A, Goeppert B, Bopp C, et al. Turquoise to dark green organs at autopsy. Virchows Arch. 2009;454:341–344.