Abstract
Recently, green metal nanoparticles have received global attention owing to their economical synthesis, biocompatible nature, widespread biomedical and environmental applications. Current study demonstrates a sustainable approach for the green synthesis of silver nanoparticles (P-AgNPs) and gold nanoparticles (P-AuNPs) from P. serrulata fresh fruit extract. The silver and gold nanoparticles were synthesized in a very rapid, efficient and facile manner, within 50 min and 30 s at 80 °C, respectively. The nanoparticles were characterized by using visual observation, UV–Vis, FE-TEM, EDX, elemental mapping, FT-IR, XRD and DLS, which confirmed the formation of monodispersed, crystalline and stable nanoparticles. Further, we explored these nanoparticles for anti-inflammatory activity through inhibition of downstream NF-κB activation in macrophages (RAW264.7). We demonstrated that the nanoparticles reduced expression of inflammatory mediators such as nitric oxide (NO), prostaglandin E2 (PEG2), inducible nitric oxide synthase (iNOS) and cyclooxygenase-2 (COX-2) was attenuated in lipopolysaccharide (LPS)-induced RAW264.7 cells. Furthermore, nanoparticles significantly suppressed LPS-induced activation of NF-κB signalling pathway via p38 MAPK in RAW 264.7 cells. To the best of our knowledge, this is the first report on the efficient green synthesis of P-AgNPs and P-AuNPs using P. serrulata fresh fruit extract and its in vitro anti-inflammatory effects. Collectively, our results suggest that P. serrulata fresh fruit extract is a green resource for the eco-friendly synthesis of P-AgNPs and P-AuNPs, which further can be utilized as a novel therapeutic agent for prevention and cure of inflammation due to their biocompatible nature.
Introduction
Biogenesis of nanoparticles using environmental friendly and economical resources is an unindustrialized part of bionanotechnology. To overcome the limitations of physiochemical synthesis, the anticipation of “green” synthesis with implementation of sustainable and eco-friendly processes for the development of green nanoparticles is essential and undeveloped part of bio-nanotechnology [Citation1,Citation2]. This is due to the fact that the production of metal nanoparticles by traditional physio-chemical approaches often requires use of toxic reducing agents, organic solvents and results in hazardous environmental waste by-products [Citation3]. Thus, it is necessary to explore “green” approaches for the nanoparticles synthesis which additionally advantageous for the applicability of nanoparticles in human health care and industrial products. Several biogenic approaches are available for the nanoparticles synthesis, which employs various natural resources like plant extracts, microorganisms, algae etc. [Citation4,Citation5]. For instance, silver and gold nanoparticles synthesized from microorganisms, like Bhargavaea indica, Brevibacterium frigoritolerans, Sporosarcina koreensis, Weissella oryzae, Pseudomonas deceptionensis, Lactobacillius kimchicus, etc., has been reported [Citation4–13]. However, due to the requirement of expensive culture medium and maintenance of highly aseptic conditions, the microbial mediated synthesis of nanoparticles is not industrially feasible [Citation14]. Therefore, exploration of the plant systems as the potential bio-nano-factories has gained heightened interest in the biological nanoparticles synthesis. Studies suggest that plant systems are sustainable, energy efficient, requires low production cost, moreover the synthesized nanoparticles are estimated to be biocompatible in nature for pharmaceutical and other biomedical applications [Citation15,Citation16]. Further, these green methodologies offer advantage of the well-defined size and morphology, easy scale-up process and production of large quantities of nanoparticles with high yield and free from any surface contamination [Citation16]. Hence, exploration into plant systems has been considered to be a potential bioreactor for synthesis of metal nanoparticles without using toxic chemicals.
On biomedical platform, metal nanoparticles are used in various medical applications including in vitro diagnostics, photo-imaging, photo-thermal therapy, delivery of drug; gene and various biomolecules [Citation15,Citation16]. The significant interest for emerging metal nanoproducts is due to their size and shape dependent unique and tunable properties. Especially, silver and gold nanoparticles are of high significance interest because of their potential applications in fields of medical nano-engineering, agriculture, crop protection, food packaging, developing biosensors and therapeutic agents for chronic disease diagnostics and treatment [Citation17]. Silver at the nanostructure level have gained considerable attention due to their antimicrobial, anticoagulant, biofilm inhibition, anticancer, anti-ageing and anti-inflammatory efficacies which make them an ideal candidate on medical platform [Citation18,Citation19]. Importantly, silver nanoparticles possess broad spectrum of antimicrobial activity against human and animal pathogens, as it affects the permeability of microbial cell membranes which make them a suitable choice for antimicrobial coating in various medical appliances and implants. Further, silver nanoparticles are applied in electronics, bio-sensing, clothing, food industry, paints, sunscreens and cosmetics [Citation20]. Therefore, it is widely being used as antimicrobial agents in commercial medical and consumer products. Instead, gold nanoparticles found to exhibit unique physicochemical properties including surface plasmon resonance, optical property and ability to allowing surface modifications, further applied in biomedical applications for photo-thermal therapy, contrast agents, radiosensitizers, etc. [Citation21,Citation22]. Recently, gold nanoparticles are emerging as promising agents for cancer diagnosis and therapy and are being investigated as drug carriers. Gold nanoparticles have been found to be active against filariasis and malaria vectors, plasmodial pathogens and cancer cells [Citation23]. With their large surface area, they can simultaneously incorporate therapeutic and imaging agents, which can be used in the treatment of cancer, microbial infection and many other diseases. Prominently, compared to the native metal nanoparticles, biosynthesized metal nanoparticles are highly valuable in therapeutics due to their biocompatible nature, stability and surface properties [Citation4]. Thus, considering these advantages current study focus on green synthesis of silver and gold nanoparticles from P. serrulata fresh fruit extract.
Following the green route, we utilize the fresh fruit extract of P. serrulata for green and facile synthesis of silver (P-AgNPs) and gold nanoparticles (P-AuNPs). P. serrulata tree lives for a short time and also known by the common names so-called Cherry Blossom, Cherry Tree, Japanese Cherry, Oriental Cherry, East Asian Cherry etc. The tree is well known for its ornamental usage [Citation24]. P. serrulata fruits contain amygdalin and prunasin substances which break down in water to form hydrocyanic acid. This compound in very small amount stimulates respiration, improves digestion and gives a sense of well-being [Citation25]. Importantly, P. serrulata fruits are reported for antioxidative, anticancer and antiviral properties. The P. serrulata can also be used as a dye. Recently, Yang et al. have showed that P. serrulata inhibited the expression of nitric oxide in LPS-induced RAW 264.7 cells. In the current study, we explored the new application of fresh fruit of P. serrulata for green and economical synthesis of P-AgNPs and P-AuNPs in a rapid and facile way. Further, to determine and characterize the efficacy P-AgNPs and P-AuNPs for treatment of inflammatory disease using macrophages.
Inflammation is a main defence system in human body. However, excessive inflammation could cause various diseases such as cancer, arthritis, and neurological diseases [Citation26,Citation27]. Several reports have shown that control of multiple inflammatory pathways is influenced by macrophage cells which are the essential cells in inflammation [Citation28,Citation29]. The nuclear factor-kappa B (NF-κB) pathways play a critical role in inflammation process, increasing the levels of cytokines and inflammatory mediators such as nitric oxide (NO), prostaglandin E2 (PEG2), inducible nitric oxide synthase (iNOS) and cyclooxygenase-2 (COX-2) induced by lipopolysaccharides (LPS) [Citation30–32]. Macrophage is activated by LPS, which produce various pro-inflammatory cytokines, such as NO, PGE2, tumour necrosis factor-α (TNF-α). Several studies have shown that inflammatory mediators derived NF-κB via extracellular signal-regulated Mitogen-activated protein kinase (MAPK) signalling pathway which consists of extracellular signal-regulated kinase (ERK), Jun kinase (JNK) and p38 MAPK [Citation33,Citation34]. Therefore, blockage of NF-κB transcription factor through the inhibition of MAPK may have possible to treatments for inflammatory diseases. Thus, we explored the P-AgNPs and P-AuNPs in RAW264.7 cell lines for anti-inflammatory efficacies.
Experimental details
Materials
All media were purchased from Difco, MB cell, Seoul, Korea. Analytical grade gold (III) chloride trihydrate (HAuCl4.3H2O) and silver nitrate (AgNO3) was purchased from Sigma-Aldrich Chemicals, USA. RAW264.7 cells, derived from murine macrophage cells (KCLB, Seoul, Korea) were cultured in Dulbecco's modified Eagle's medium (DMEM) medium containing 10% heat-inactivated foetal bovine serum (FBS) and 1% penicillin/streptomycin (P/S) at 37 °C, 5% CO2 humidified incubator. DMEM was purchased from Gibco-BRL (Grand Island, NY, USA). FBS, P/S, and Phosphate-buffered saline (PBS) were obtained from WelGENE Inc. (Daegu, Korea). Primary antibodies against iNOS, COX-2, NF-κB and IKKα/β were from Santa Cruz Biotechnology (Santa Cruz, CA). Antibodies against ERK, phosphor(p)-ERK, JNK, p-JNK, p38 and p-p38 were obtained from Cell Signalling (Beverly, MA).
Green synthesis of P-AgNPs and P-AuNPs
For green synthesis of nanoparticles, previously reported methodology followed [Citation1–3]. Briefly, P. serrulata fresh ripen fruit 7 g was collected, and washed thoroughly with distilled water. Then, the fruits were then added into 9 ml of water and crushed thoroughly to release complete fruit extract and its derived components. This fruit extracted water was then collected by filtration in order to completely remove the suspended materials. The aqueous extract was further purified by centrifugation at 8000 rpm for 5 min to remove any fine suspended particles, finally, obtained in liquid form and considered as stock solution. Stock solution was further diluted in water at ratio of 1:9 (aqueous fruit extract to water). This diluted volume was used to synthesize nanoparticles. For synthesis of silver and gold nanoparticles, final concentration of 1 mM filter-sterilized solution of AgNO3 and HAuCl4·3H2O were maintained respectively, in 30 ml of diluted fruit extract and kept for incubation at 80 °C. Synthesis was monitored visually for a visual colour change of the reaction mixture. After complete reduction of metal salts to nanoparticles, the nanoparticles were purified by first centrifugation at 2000 rpm for 5 min to remove undesirable big components and then nanoparticles were collected by high speed centrifugation at 20,000 rpm for 10 min. The obtained nanoparticles product was washed thoroughly by distilled water to remove unconverted metal ions or any other constituents. Finally, nanoparticles were collected in the form of a pellet by air drying, further, were used for analytical characterization and in vitro applications.
Characterization of nanoparticles
UV–vis spectrophotometer (UV–Vis) (Ultrospec 2100 Pro, Amersham, Biosciences) was used to confirm reduction of metal ions by scanning synthesis mixture in range of 300–700 nm. Partially purified nanoparticles products were further characterized by using field emission transmission electron microscopy (FE-TEM), elemental mapping and energy dispersive X-ray spectroscopy (EDX) with a JEM-2100 F (JEOL) instrument operated at 200 kV. Samples for FE-TEM was prepared by placing a drop of collected nanoparticles on carbon coated copper grid and subsequently drying in an oven at 60 °C before transferring it to the microscope. X-ray diffraction (XRD) analyses were performed on X-ray diffractometer, D8 Advance, (Bruker), Germany, operated at 40 kV, 40 mA, with CuKα radiation, at a scanning rate of 6°/min, step size 0.02, over the 2θ range of 20–80°. For XRD analysis, nanoparticles sample preparation was done by air drying purified nanoparticles, and obtained in powder form [Citation5]. Fourier Transform-Infrared (FT-IR) measurements, air-dried nanoparticles powder samples were scanned on a PerkinElmer Spectrum One FT-IR spectrometer over the range of 4000–450 cm−1 at a resolution of 4 cm−1 [Citation5,Citation6]. FT-IR analysis was done to study the interactions between functional groups present as a source of reducing, catalytic and capping agents on surface of synthesized nanoparticles [Citation13]. Spectra recorded were plotted as transmittance (%) versus wavenumber (cm−1). Particle size distribution of nanoparticles was studied using dynamic light scattering (DLS) (Photal, Otsuka Electronics, Japan). Hydrodynamic diameter and polydispersity index (PDI) were analyzed at 25 °C. As a reference, a dispersive medium of pure water with a refractive index of 1.3328, viscosity of 0.8878 and dielectric constant of 78.3 was used.
Nanoparticles stability was detected by measuring absorbance of purified P-AgNPs and P-AuNPs solution, kept for different time interval at room temperature. Additionally, effect of change in pH on nanoparticles solution was studied, to address the particles stability. For pH stability, sodium hydroxide (NaOH) solution was added in range of 4–10 pH and then absorbance has been measured by UV–vis.
Anti-inflammatory action of nanoparticles
Cell culture
Murine macrophage cell line RAW 264.7 was purchased from Korean Cell Line Bank (Seoul, South Korea), respectively. RAW 264.7 was cultured in DMEM medium (GIBCO-BRL, Rockville, MD) supplemented with 10% heat-inactivated foetal bovine serum (FBS) and 1% penicillin/streptomycin (P/S) (WelGENE Inc., Daegu, Korea). Cells were incubated at 37 °C in a humidified atmosphere containing 5% CO2 and 95% air.
MTT assay
Cell viability was determined by MTT assay as described previously [Citation21,Citation35]. To determine the toxic effects of P-AgNPs and P-AuNPs on cell viability, cells were treated with various concentration (0–200 μg/ml) for 24 h. After 1-day treatment, cell viability was measured by MTT assay. 10 μl MTT solutions (5 mg/mL) added to each well, and the plates were incubated for an additional 3–4 h, and the formazan formed was dissolved in DMSO; then absorbance of each well was recorded on a synergy 2 multi-mode microplate reader at 570 nm (BioteK, Winooski, VT). Optical density of formazan formed in control (untreated) cells was taken as 100%.
Measurement of NO production
Nitrite production was determined by Griess methods as described before [Citation21,Citation35,Citation36]. To determine levels of nitrite (NO) in the cultured cells, RAW 264.7 cells were seeded as mentioned in the cell viability assay, and pre-treated of 1 μg/ml LPS with various concentrations of P-AgNPs and P-AuNPs or L-NMMA for 24 h. Next, we used Griess reagents to determine nitrite levels in cultured cells’ suspended media. Briefly, an equal volume (100 μl) of supernatants cultured cells were mixed with Griess reagent; then absorbance was read at 540 nm against a standard sodium nitrite curve.
Enzyme-linked immunosorbent assay (ELISA)
Level of PGE2 productions were measured an enzyme-linked immunosorbent assay (ELISA) kit (R&D Systems, Minneapolis, MN) according to the manufacturer's instructions. Absorbance was measured at 450 nm, with subtraction measured at 540 nm. Concentration of PGE2 concentration in each well was then calculated from its respective standard curves.
Reverse transcription polymerase chain reaction (RT-PCR)
PCR reactions and conditions were as described previously [Citation35–37]. RAW264.7 cells were stimulated with LPS (1 μg/ml) in the presence or absence of P-AgNPs and P-AuNPs. Total RNA was isolated by harvesting whole cell lysate using TRIzol LS reagents (Invitrogen, Carlsbad, CA) according to the protocol supplied by the manufacturer. Total RNA (2 µg) was used to synthesize 1st stranded cDNA using thermo scientific cDNA synthesis kit (Onebio, Lithuania EU), according to the manufacturer’s instructions. The following primers used for RT-PCR are listed in .
Table 1. The primer sequences of the tested genes.
Immunoblotting analysis
Immunoblotting as described previously [Citation36,Citation37]. Proteins were extracted from cells in ice-cold using PRO-PREPTM Protein Extraction Solution (iNtRON Biotechnology, Seongnam-Si, Korea) according to the provided protocol (Sungnam, Kyungki-Do, Korea). Thirty micrograms of total protein per lane was separated by 8% sodium dodecyl sulphate (SDS)-polyacrylamide gel electrophoresis (PAGE). Proteins were then transferred to a nitrocellulose membranes (Millipore), after 1.5 h incubation the membrane was blocked with 5% skim milk, and probed with the respective primary antibodies. After five times washing (5 min/wash), the membranes were incubated with the horseradish peroxidase-conjugated secondary antibodies for 2 h and blot was detected using an enhanced chemiluminescence system (Amersham Biosciences Inc., Piscataway, NJ) and exposed to X-ray film (Fuji Photo Film Co. Ltd).
Statistical analysis
All data expressed as means ± standard deviations (SD) were either calculated from at least three independent experiments. Student’s t-test and two-way analysis of variance (ANOVA) were used to determine statistical significance of differences between treated and untreated (control) groups. Significant differences two treatment groups with p < .05, p < .01 and p < .001, respectively [Citation36,Citation37].
Results and discussion
Green route of nanoparticles synthesis is always found to be an eco-friendly approach for developing biocompatible nanoparticles. In the current work, we demonstrated this eco-friendly approach by using fresh fruit extract of P. serrulata for green synthesis of P-AgNPs and P-AuNPs. Further, these synthesized nanoparticles were purified and characterized by several analytical techniques and applied for anti-inflammatory efficacy in RAW264.7 cells. shows the schematic illustration of P-AgNPs and P-AuNPs synthesis from fresh fruit extract of P. serrulata and its detailed action mechanism for anti-inflammatory efficacy on RAW264.7 cell lines.
Figure 1. Schematic illustration of green synthesis of P-AgNPs and P-AuNPs from P. serrulata fresh fruits extract and its applications on inflammatory cells with outlined detailed mechanism.
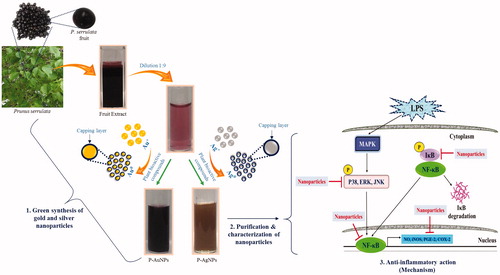
Green synthesis of P-AgNPs and P-AuNPs from P. serrulata fresh fruit extract
Initially, synthesis of nanoparticles was confirmed by visual observation with appearance of colour change in reaction mixture. For P-AgNPs, colour transformed from ruby red to brown within 50 min, which resembles synthesis of silver nanoparticles, as particles cause surface plasmon resonance due to which deep brown colour within 50 min at 80 °C (. For P-AuNPs, reaction mixture colour turns from light ruby red to deep purple colour within 30 s at 80 °C. Gold nanoparticles exhibit ruby red to dark purple colour in aqueous solutions due to excitation of surface plasmon vibrations by gold nanoparticles (. Thus, appearance of deep purple colour in reaction mixture indicated the formation of gold nanoparticles [Citation23]. The methodology for nanoparticles synthesis was green, simpler and more cost-effective. Exact mechanism behind the synthesis of nanoparticles remains to elucidate, however, reports proposed that, plat components, for instance, proteins released by the fruit extract are liable for reduction reaction [Citation38]. Thus, first confirmatory visual observation that indicated the synthesis of P-AgNPs and P-AuNPs in reaction mixture has been observed. Afterwards, nanoparticles were purified by several water wash and centrifugation cycles, and finally, air dried and collected in pellet form. These air dried samples further used for characterization.
Characterization of P-AgNPs and P-AuNPs
For characterization of nanoparticles, reaction mixture was monitored by UV–Vis spectral analysis. Reaction mixture after incubation period was scanned in the range of 300–800 nm. It has been reported that, UV–Vis absorption spectrum shows highest absorbance in the range of 400–500 nm for silver nanoparticles and 500–600 nm for gold nanoparticles [Citation6,Citation7]. In our results, the UV–Vis absorption spectrum, showed a strong peak at about 440 nm and 558 nm, which is attributed to the surface plasmon resonance band of silver and gold nanoparticles (), respectively. By using FE-TEM, various studies characterized different shape and size of nanoparticles synthesized by biological resources [Citation8,Citation9]. In the present study, FE-TEM results revealed spherical shape for silver nanoparticles () and hexagonal shape for gold nanoparticles (). Nanoparticles synthesised using P. serrulata were quite monodisperse in nature, which avoid the polydispersity problem associated with biogenic synthesis. It has been reported that numerous plant metabolites, for instance, proteins, polyphenols, terpenoids, phenolic acids, alkaloids and sugars play an important role in bioreduction of metal ions, yielding nanoparticles. Next, elemental mapping results of nanoparticles indicated the maximum distribution of silver and gold elements in their respective electron micrograph image of corresponding nanoproducts, which further suggested that silver and gold elements were predominant in distributions (). EDX spectrum of nanoparticles showed highest peak at 3 keV for P-AgNPs and 2.15 keV for P-AuNPs () [Citation6]. Other metal ions group also appeared in EDX spectrum which corresponds to TEM grid utilized for study. EDX results were quite similar with previous reports of characterization of silver and gold nanoparticles. X-ray diffraction pattern of nanoparticles, exhibited intense peaks in whole spectrum of 2θ value ranging from 20 to 80 and this pattern was similar to the Braggs's reflection of silver and gold nanocrystals (). Similar values reflected form SAED (selected area electron diffraction) pattern of purified single nanoparticle as well, which further confirm crystalline nature of nanoparticles.
Figure 3. FE-TEM image of spherical shape P-AgNPs (A, B) and hexagonal shape P-AuNPs (C, D). Elemental mapping of P-AgNPs, electron micrograph image and respective silver elements distribution (E, F). Elemental mapping of P-AuNPs electron micrograph image and respective gold elements distribution (G, H). EDX spectrum of P-AgNPs (I) and P-AuNPs (J), respectively.
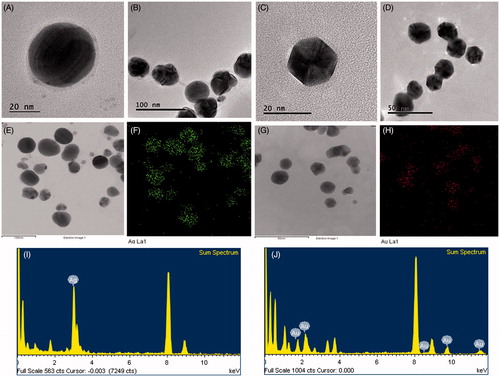
Figure 4. XRD spectrum and SEAD pattern of P-AgNPs (A), and P-AuNPs (B), showing crystalline nature of nanoparticles.
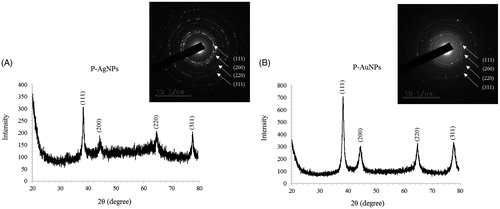
Previously, FT-IR spectroscopy demonstrated that plant metabolites such as sugars, terpenoids, polyphenols, alkaloids, phenolic acids and proteins play an important role in reduction of metal ions into nanoparticles and in supporting their subsequent stability (). FT-IR analysis was conducted to identify organic compounds responsible for bio-reduction of metal ions into metal nanoparticles and protein interactions excreted by plant extract (). Results revealed intense band at 3746.77, 3454.12, 2922.06, 2851.64, 1620.09, 1541.42, 1297.69, 1015.02 and 688.67 cm−1 for silver nanoparticles and at 3829.94, 3790.83, 3744.67, 3407.95, 2917.90, 2851.64, 2353.45, 2317.49, 1631.14, 1541.10, 1260.36, 1047.40, 820.35, 667.10 and 599.56 for gold nanoparticles. Bands in the range of 3,500–3,000 cm−1 represent –N–H and OH–O stretching. In the case of P-AgNPs, FT-IR peaks indicated that proteins and other aromatic organic compounds from P. serrulata fresh fruit extract played major role in synthesis and stabilization of nanoparticles [Citation24,Citation38]. For P-AuNPs, peaks at 2917.90, 2851.64 cm−1 could be attributed to –CH2 asymmetric stretching. Band at 1631.14 cm−1 could be equated to an amide 1 bond of the polypeptides. Peaks ranging from 1650 to 1450 cm−1 indicate the presence of an aromatic ring and elongation of aromatic C = C bonds. Bands between 1100 and 1010 cm−1 in the spectrum were assigned to C–O and C–H chemical bonds. Deformation vibration of C–C bonds in phenolic groups creates peaks between 1500 and 1400 cm−1. Following it, in P-AuNPs, obtained peaks suggested that phenolic compounds from P. serrulata fresh fruit extract could be mainly responsible for synthesis of gold nanoparticles [Citation24,Citation38]. Proteins and amino acid residues are reported to be responsible for providing stability to synthesized nanoparticles. Following, phenolic compounds from P. serrulata fresh fruit extract are predicted to form a coating around nanoparticles, thereby contributing to their stability. Previously, studies have reported that gold nanoparticles from plant extracts contain strong characteristic peaks of primary amines and carbonyl stretch in the amide linkages which suggest that free amino groups due to amino acid residues and surface-bound proteins are incumbent for the formation of capping layers on the nanoparticles and preventing their agglomeration [Citation24,Citation38]. Further, nanoparticles from plant extract possess characteristic binding affinity to thiols, amines and amides, which are present in amino acid residues. Thus, the active groups surround the P-AgNPs and P-AuNPs from P. serrulata fresh fruit extract play major role in providing stability, additionally, it may help in binding of some active drug or biomolecules due to free surface groups, which further, could be helpful for drug or biomolecules delivery. Similar characteristic peaks of FT-IR spectra were also observed in silver and gold nanoparticles synthesized from Siberian ginseng in previously published reports.
Figure 5. FT-IR profile of P-AgNPs and plant (fruit) extract (A), P-AuNPs and plant (fruit) extract (B), respectively.
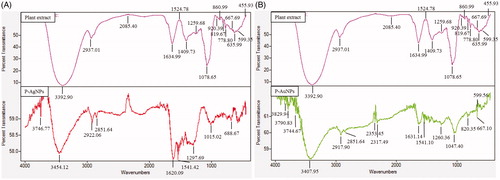
Particle size analysis results have well demonstrated the distribution of P-AgNPs and P-AuNPs according to intensity, number and volume of nanoparticles. Average particles size observed for P-AgNPs was 66 nm with PDI 0.275 and for P-AuNPs was 65 nm with PDI 0.287, respectively ()). Stability of P-AgNPs and P-AuNPs was determined by keeping purified nanoparticles solution for different time intervals up to a month at room temperature. There was no observable variation in UV–vis spectrum of nanoparticles solution even after one month, which revealed nanoparticles stability in aqueous system. In addition, effect of pH change in range of 4–10 on nanoparticles stability was studied. Nanoparticles solution was observed before and after addition of NaOH. In results, no major change in wavelength was observed, which further supports P-AgNPs and P-AuNPs stability.
Inhibition of LPS-induced inflammatory response byP-AgNPs and P-AuNPs on RAW264.7 cells
Green synthesis of nanoparticles using natural plants extract shows diverse advantages among which various phytochemicals present in the plant plays crucial role in providing biocompatibility and stability to nanoparticles [Citation39]. LPS is gram-negative bacteria and triggers macrophages to involve in inflammatory activities. Many research groups report evidence that LPS induced Raw264.7 macrophage was used as in vitro model of inflammation to assess potential anti-inflammatory agents. In order to examine the inhibitory effect of P-AgNPs and P-AuNPs for treatment of inflammation, we used LPS-induced RAW264.7 cells line as in vitro model. The objective of this study was to determine if our sample can safely reduce NF-κB activation in anti-inflammatory disease. MTT assay is used to determine the effects of P-AgNPs and P-AuNPs on cell viability of RAW 264.7 cells. As shown in , P-AgNPs nanoparticles had no cytotoxic effect at concentrations ranging from 1 to 25 μg/ml. In addition, P-AuNPs did not exhibit cytotoxic effect at the investigated concentrations up to 100 μg/ml on RAW264.7 cells. Therefore, subsequent experiments were performed using concentrations of 50 µg/ml or lower.
Figure 7. Effects of P-AgNPs (A) and P-AuNPs (B) on cell viability respectively. For cell viability assay, RAW264.7 cells were incubated for 24 h with various concentrations of P-AgNPs (1–100 μg/ml) and P-AuNPs (1–200 μg/ml).
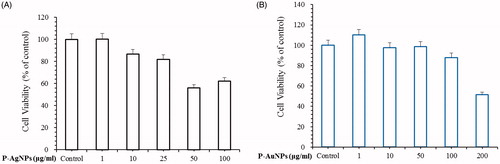
Number of studies have suggested that the inhibition and down regulation of iNOS and COX-2 reduces the development of several diseases, including inflammatory bowel syndrome, atherosclerosis, cancer and allergic encephalomyelitis. Furthermore, majority of inflammatory diseases are cause by the up-regulation of PGE2 and NO production. Several studies have revealed that these cytokines are critically involved in inflammation, different auto-immune disorders and tumour production. Due to their unique role in development of inflammation, down regulation of iNOS, COX-2 and NF-κB is an important therapy for proper treatment and prevention of inflammatory diseases. As inflammatory process progressed, various inflammatory mediators and growth factors are released. Previous studies evidence that nitrite and COX-2 is a basic inflammatory component in development of inflammatory process [Citation37,Citation39]. In order to examine inhibitory activity of P-AgNPs and P-AuNPs on inflammatory mediators, we performed nitrite oxide and PGE2 assay as a result of NO and PGE2 production. Our observations confirmed that P-AgNPs and P-AuNPs inhibited the LPS-induced production of NO and PGE2. Nitrite analysis exhibited that the treatment of both samples can inhibit nitrite activity compare with LPS-induced control group. P-AgNPs showed approximately 60% inhibition at all concentration of nanoparticles. Furthermore, P-AuNPs showed that the inhibition level of nitrite was as N-Methylarginine (L-NMMA) which is an inhibitor of nitric oxide synthase (). The PGE2 production analysis also demonstrated that the treatment of both samples showed a decreasing effect on the level of PGE2 in LPS stimulated RAW264.7 cells (). Moreover, our results show that both samples reduced the production of LPS-mediated inflammatory genes and proteins iNOS and COX-2 in RAW264.7 cells (). Therefore, it suggested that P-AgNPs and P-AuNPs have anti-inflammatory effects by inhibiting secretion of pro-inflammatory mediators in RAW264.7 cells stimulated by LPS.
Figure 8. Effects of P-AgNPs and P-AuNPs on the production of NO (A) and PGE2 (B). Macrophages RAW264.7 cells were pre-treated both samples for 1 h, and then stimulated with LPS (1 μg/mL) for 24 h. The concentrations of Nitrite and PGE2 were determined as described in the materials and methods. Data shown represent the mean values of three experiments ± SD. ***p < .001as compared to the group treated with LPS.
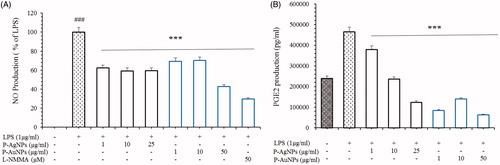
Figure 9. Effects of P-AgNPs and P-AuNPs on iNOS and COX-2 activation. RAW264.7 cells were pre-treated with the indicated concentrations of both samples for 1 h, and then stimulated with LPS (1 μg/mL) for 24 h. Subsequently, total RNAs were extracted and the mRNA expression levels iNOS and COX-2 were determined by RT-PCR analysis and compared with those of β-actin (A, B and C). Total protein levels of iNOS and COX-2 in whole lysates from LPS-activated RAW264.7cells were determined by western blot analysis (D). The data shown are representative of mean values of three independent experiments ± SD. ***p < .001 as compared to the group treated with LPS and ###p < .001 as compared to the LPS-treated cells.
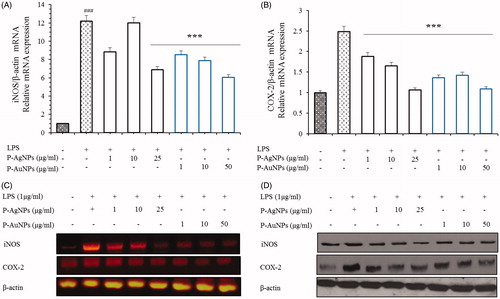
Sample inhibited NF-κB activation induced byLPS-stimulated RAW264.7 cells
Inflammatory pathways like those of NF-κB and p38 mitogen-activated protein kinase (MAPK) are activated to increase the production of inflammatory mediators and cytokines. These activated NF-κB and MAPK signals have capability to accelerate inflammation [Citation40,Citation41]. MAPK signalling pathway is an important intracellular mechanism and is known to play a central role in the phosphorylation of transcription factors including NF-κB by producing high levels of numerous pro-inflammatory factors [Citation40–42]. Therefore, we examined whether samples suppressed LPS-mediated induction of protein levels through NF-κB signalling via the p38 MAPK pathway. NF-κB pathways known to play a role in various inflammatory diseases have thus been attractive targets for the development of anti-inflammatory drugs. As shown in , co-treatment with P-AgNPs and P-AuNPs dose-dependently decreased the expression of p-IκB, NF-κB p-38, p-JNK and p-ERK proteins. Thus, the present study showed that both sample suppressed the production of inflammatory mediators and pro-inflammatory cytokines in LPS-induced RAW264.7 cells via inhibition of NF-κB activation. These findings suggest that both samples can be utilized as an effective agent in inflammatory diseases.
Figure 10. Effect of P-AgNPs and P-AuNPs on LPS-induced NF-κB and MAPK phosphorylation. RAW264.7 cells cultures stimulated with LPS (1 μg/ml) in the absence or presence of P-AgNPs and P-AuNPs. Whole cell lysates were subjected to Western blot analysis using antibodies against phosphorylation of IκB, NF-κB (A) and MAPK (B) compared with those of β-actin.
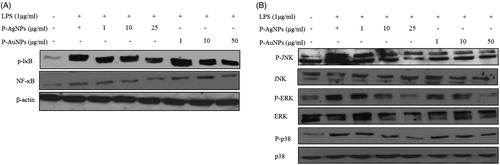
Conclusion
Green nanotechnology involves use of energy-efficient eco-friendly approaches for production of nanoparticles using biosustainable methodologies and eco-friendly substances. The present work highlights facile green synthesis of P-AgNPs and P-AuNPs in an eco-friendly manner by fresh fruit extract of P. serrulata. Synthesized nanoparticles were mono-disperse and stable in nature. Moreover, nanoparticles were found to be capable of showing anti-inflammatory effect in RAW264.7 cell lines. Thus, the study showed green route of synthesis of P-AgNPs and P-AuNPs and their applicability for anti-inflammatory efficacy in RAW264.7 cell lines based on in vitro studies.
Disclosure statement
No potential conflict of interest was reported by the authors.
Additional information
Funding
References
- Singh P, Kim YJ, Yang DC. A strategic approaches for rapid synthesis of gold and silver nanoparticles by Panax ginseng leaves. Artif Cells Nanomed Biotechnol. 2015;44:1949–1957.
- Singh P, Kim YJ, Singh H, et al. Biosynthesis of anisotropic silver nanoparticles by Bhargavaea indica and their synergistic effect with antibiotics against pathogenic microorganisms. J Nanomater. 2015;2015:10.
- Singh P, Kim YJ, Singh H, et al. Biosynthesis, characterization, and antimicrobial applications of silver nanoparticles. Int J Nanomed. 2015;10:2567–2577.
- Singh P, Kim YJ, Zhang D, et al. Biological synthesis of nanoparticles from plants and microorganisms. Trends Biotechnol. 2016;34:588–599.
- Singh P, Kim YJ, Wang C, et al. The development of a green approach for the biosynthesis of silver and gold nanoparticles by using Panax ginseng root extract, and their biological applications. Artif Cells Nanomed Biotechnol. 2016;44:1150–1157.
- Singh P, Kim YJ, Wang C, et al. Biogenic silver and gold nanoparticles synthesized using red ginseng root extract, and their applications. Artif Cells Nanomed Biotechnol. 2016;44:811–816.
- Singh P, Kim YJ, Wang C, et al. Microbial synthesis of flower-shaped gold nanoparticles. Artif Cells Nanomed Biotechnol. 2016;44:1469–1474.
- Singh P, Singh H, Kim YJ, et al. Extracellular synthesis of silver and gold nanoparticles by Sporosarcina koreensis DC4 and their biological applications. Enzyme Microb Technol. 2016;86:75–83.
- Singh P, Kim YJ, Wang C, et al. Weissella oryzae DC6-facilitated green synthesis of silver nanoparticles and their antimicrobial potential. Artif Cells Nanomed Biotechnol. 2016;44:1569–1575.
- Singh P, Kim YJ, Yang DC. A strategic approach for rapid synthesis of gold and silver nanoparticles by Panax ginseng leaves. Artif Cells Nanomed Biotechnol. 2016;44:1949–1957.
- Singh H, Du J, Yi TH. Biosynthesis of silver nanoparticles using Aeromonas sp. THG-FG1.2 and its antibacterial activity against pathogenic microbes. Artif Cells Nanomed Biotechnol. 2017;45:584–590.
- Jo JH, Singh P, Kim YJ, et al. Pseudomonas deceptionensis DC5-mediated synthesis of extracellular silver nanoparticles. Artif Cells Nanomed Biotechnol. 2016;44:1576–1581.
- Markus J, Mathiyalagan R, Kim YJ, et al. Intracellular synthesis of gold nanoparticles with antioxidant activity by probiotic Lactobacillus kimchicus DCY51(T) isolated from Korean kimchi. Enzyme Microb Technol. 2016;95:85–93.
- Velusamy Kumar GV, Jeyanthi V, Das J, et al. Bio-inspired green nanoparticles: synthesis, mechanism, and antibacterial application. Toxicol Res. 2016;32:95–102.
- Abbai R, Mathiyalagan R, Markus J, et al. Green synthesis of multifunctional silver and gold nanoparticles from the oriental herbal adaptogen: Siberian ginseng. Int J Nanomedicine. 2016;11:3131–3143.
- Makarov VV, Love AJ, Sinitsyna OV, et al. “Green” nanotechnologies: synthesis of metal nanoparticles using plants. Acta Nat. 2014;6:35–44.
- Chung IM, Park I, Seung-Hyun K, et al. Plant-mediated synthesis of silver nanoparticles: their characteristic properties and therapeutic applications. Nanoscale Res Lett. 2016;11:40.
- Castro-Aceituno V, Ahn S, Simu SY, et al. Anticancer activity of silver nanoparticles from Panax ginseng fresh leaves in human cancer cells. Biomed Pharmacother. 2016;84:158–165.
- Wei L, Lu J, Xu H, et al. Silver nanoparticles: synthesis, properties, and therapeutic applications. Drug Discov Today. 2015;20:595–601.
- Ge L, Li Q, Wang M, et al. Nanosilver particles in medical applications: synthesis, performance, and toxicity. Int J Nanomedicine. 2014;9:2399–2407.
- Ahn S, Singh P, Castro-Aceituno V, et al. Gold nanoparticles synthesized using Panax ginseng leaves suppress inflammatory-mediators production via blockade of NF-kappaB activation in macrophages. Artif Cells Nanomed Biotechnol. 2016;45:270–276.
- Dykman LA, Khlebtsov NG. Gold nanoparticles in biology and medicine: recent advances and prospects. Acta Nat. 2011;3:34–55.
- Arvizo R, Bhattacharya R, Mukherjee P. Gold nanoparticles: opportunities and challenges in nanomedicine. Expert Opin Drug Deliv. 2010;7:753–763.
- Poonam V, Raunak GK, Reddy LC, et al. Chemical constituents of the genus Prunus and their medicinal properties. Curr Med Chem. 2011;18:3758–3824.
- Yook HS, Kim KH, Park JE, et al. Antioxidative and antiviral properties of flowering cherry fruits (Prunus serrulata L. var. spontanea). Am J Chin Med. 2010;38:937–948.
- Lee HJ, Jeong HS, Kim DJ, et al. Inhibitory effect of citral on NO production by suppression of iNOS expression and NF-kappa B activation in RAW264.7 cells. Arch Pharm Res. 2008;31:342–349.
- Nam N. Naturally occurring NF-κB inhibitors. Mini Rev Med Chem. 2006;6:945–951.
- Jin M, Suh SJ, Yang JH, et al. Anti-inflammatory activity of bark of Dioscorea batatas DECNE through the inhibition of iNOS and COX-2 expressions in RAW264.7 cells via NF-κB and ERK1/2 inactivation. Food Chem Toxicol. 2010;48:3073–3079.
- Kim DH, Chung JH, Yoon JS, et al. Ginsenoside Rd inhibits the expressions of iNOS and COX-2 by suppressing NF-κB in LPS-stimulated RAW264.7 cells and mouse liver. J Ginseng Res. 2013;37:54–63.
- Hu C, Kitts DD. Luteolin and luteolin-7-O-glucoside from dandelion flower suppress iNOS and COX-2 in RAW264.7 cells. Mol Cell Biochem. 2004;265:107–113.
- Iravani S. Green synthesis of metal nanoparticles using plants. Green Chem. 2011;13:2638–2650.
- Meng Z, Yan C, Deng Q, et al. Curcumin inhibits LPS-induced inflammation in rat vascular smooth muscle cells in vitro via ROS-relative TLR4-MAPK/NF-κB pathways. Acta Pharmacol Sin. 2013;34:901–911.
- Kim J, Park SJ, Yun K, et al. Isoliquiritigenin isolated from the roots of Glycyrrhiza uralensis inhibits LPS-induced iNOS and COX-2 expression via the attenuation of NF-kappaB in RAW 264.7 macrophages. Eur J Pharmacol. 2008;584:175–184.
- Yoon HJ, Moon ME, Park HS, et al. Chitosan oligosaccharide (COS) inhibits LPS-induced inflammatory effects in RAW 264.7 macrophage cells. Biochem Biophys Res Commun. 2007;358:954–959.
- Ahn S, Siddiqi MH, Castro-Aceituno V, et al. Ginsenoside Rg5: Rk1 attenuates TNF-α/IFN-γ-induced production of thymus-and activation-regulated chemokine (TARC/CCL17) and LPS-induced NO production via downregulation of NF-κB/p38 MAPK/STAT1 signaling in human keratinocytes and macrophages. In Vitro Cell Dev Biol Anim. 2016;52:287–295.
- Sun Z, Andersson R. NF-kappaB activation and inhibition: a review. Shock. 2002;18:99–106.
- Wang T, Wu F, Jin Z, et al. Plumbagin inhibits LPS-induced inflammation through the inactivation of the nuclear factor-kappa B and mitogen activated protein kinase signaling pathways in RAW 264.7 cells. Food Chem Toxicol. 2014;11:177–183.
- Wang C, Mathiyalagan R, Kim YJ, et al. Rapid green synthesis of silver and gold nanoparticles using Dendropanax morbifera leaf extract and their anticancer activities. Int J Nanomedicine. 2016;11:3691–3701.
- Chen C, Lin M, Liang C, et al. The anti-inflammatory effects and mechanisms of eupafolin in lipopolysaccharide-induced inflammatory responses in RAW264.7 macrophages. PLoS One. 2016;11:e0158662.
- Choi RJ, Chun J, Khan S, et al. Desoxyrhapontigenin, a potent anti-inflammatory phytochemical, inhibits LPS-induced inflammatory responses via suppressing NF-κB and MAPK pathways in RAW 264.7 cells. Int Immunopharmacol. 2014;18:182–190.
- Lee TH, Jung M, Bang M, et al. Inhibitory effects of a spinasterol glycoside on lipopolysaccharide-induced production of nitric oxide and proinflammatory cytokines via down-regulating MAP kinase pathways and NF-κB activation in RAW264.7 macrophage cells. Int Immunopharmacol. 2012;13:264–270.
- Choi RJ, Shin EM, Jung HA, et al. Inhibitory effects of kaurenoic acid from Aralia continentalis on LPS-induced inflammatory response in RAW264. 7 macrophages. Phytomedicine. 2011;18:677–682.