Abstract
This study developed a novel bioactive chitosan nanoparticle loaded calcium alginate hydrogel to regulate inflammation and neovascularization for accelerated wound healing in vivo. It was found that chitosan nanoparticles loaded calcium alginate hydrogel exhibited remarkable antibacterial activity. Through the modulation of generation of ROS, it promoted the synthesis and secretion of IL-6 in vascular endothelial cell (VEC), suggesting its potential proinflammatory activation. Further, it promoted VEC invasion, metastasis and neovascularization to accelerate wound healing.
Introduction
Natural (acute) wound healing is a coordinated process that includes several largely overlapping phases that involve an inflammatory response and associated cellular migration, proliferation, matrix deposition and tissue remodelling [Citation1,Citation2]. When the skin injury was initially occurred, haemostasis was immediately induced by the assembly of a fibrin clot, and initiated the invasion and recruitment of inflammatory and other cells for achieving debridement and anti-infection effect [Citation3–5]. Vascular endothelial cells (VECs) played an important role on the formation of capillary vessels and brought an important effect in the final healing of wound. The rapid growth of VECs formed new blood vessels in the granulation tissue and supplied nutrients to the facilitate the skin regeneration by enhancing proliferation and migration of fibroblasts and keratinocytes for re-establishing the normal cellular and extracellular matrix (ECM) composition of skin [Citation6–10]. Therefore, designing therapeutic treatment on the regulation of inflammation and neovascularization showed a specific multi-target profile and had been regarded as a possible strategy to improve the wound healing effect.
Reactive oxygen species (ROS) played important roles in vascularization and it was reported that some substance showed suppression of ROS mediated VEGF signalling and had an anti-angiogenic effect on pathologic retinal neovascularization [Citation11–15]. In addition, ROS are generated after tissue injury through initiating acute inflammation and some results indicated that ROS induced ERK activation and led to the activation of pro-inflammatory cytokine responding to mechanical injury in the acute phase [Citation16].
Compared with the traditional transdermal drug delivery systems, nanotechnology-driven therapeutic interventions showed the potential aspects on facilitating the wound healing process and ultimately repairing the injured tissue [Citation17–21]. Especially, it was reported that a large amount of ROS were produced via internalization of some nanoparticles, indicating the possibility that NPs may exhibit intrinsic properties beneficial for wound treatment via NPs mediated ROS production [Citation22–25]. Based on the primary hypothesis of our study, we prepared a novel bioactive chitosan nanoparticles loaded calcium alginate hydrogel (CS NPs loaded CaAlg hydrogel) with a specific multi-target profile on the regulation of anti-bacteria, inflammation and neovascularization. Moreover, its preliminary possibility for accelerated wound healing in vivo was further investigated ().
Figure 1. The primary hypothesis of this study. Chitosan nanoparticle loaded calcium alginate hydrogel exhibited antibacterial activity and triggered the generation of ROS, thus enhancing acute inflammatory response which was conducive to debridement and anti-infection effect. In the stage of skin tissue regeneration, it promoted proliferation and migration of VEC, and led to rapid healing.
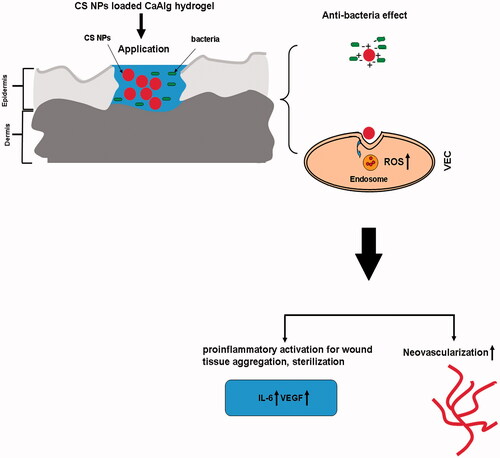
Materials and methods
Materials
The polymer chitosan (MW, 100–150 kDa; degree of deacetylation, 85%) was acquired from Haixin Biological Product Co., Ltd (Ningbo, PR China); alginic acid with molecular weight of approximately 160 kDa was purchased from Qingdao Huanghai Biological Pharmaceutical Co., Ltd (Qingdao, PR China); tripolyphosphate (TPP) and calcium chloride were purchased from Sigma-Aldrich (St. Louis, MO); Dulbecco’s modified Eagle’s medium (DMEM) and foetal bovine serum (FBS) were purchased from Gibco Technologies (Logan, UT); all other chemicals purchased were of analytical grade and were obtained from a variety of vendors. Human umbilical vein endothelial cells (HUVECs) were obtained from the Cell Line Bank of the Chinese Academy of Sciences (Shanghai, China).
Preparation and identification of chitosan nanoparticles loaded calcium alginate hydrogel
According to our previous reports [Citation26], chitosan NPs were prepared by ionic crosslinking method. Certain amount of sodium alginate was dissolved in distilled water followed by the respective addition of CS NPs under stirring until NPs were fully dispersed in solution. When calcium chloride used as the best Ca2+ sources for the ionic crosslinking of alginate was added into the solution, homogeneous alginate hydrogel cross-linked with Ca(2+) cations was formed and CS nanoparticles were loaded into the matrix of calcium alginate hydrogel. By comparison, chitosan conjugated calcium alginate hydrogel (CS-CaAlg hydrogel) was prepared by the addition of calcium chloride into the mixture of chitosan and sodium alginate. The preparation of chitosan nanoparticles loaded calcium alginate hydrogel is shown in . The characterization of CS NPs loaded CaAlg hydrogel was investigated by affinity-1 infrared spectroscopy (Shimadzu, Kyoto, Japan). Scanning electron microscopy (S-4800, Hitachi, Tokyo, Japan) was used to observe the morphology of CS NPs loaded CaAlg hydrogel. Zetasizer (Nano ZS90; Malvern Instruments, Malvern, UK) was used to determine the size, morphology and zeta potential of CS NPs. Rheological studies of CS-CaAlg hydrogel and CS NPs loaded CaAlg hydrogel were conducted using rotational rheometer (Anton Paar MCR302, Graz, Austria). Swelling capacity of gels was also determined by calculating the difference between the initial weight of the gel (W0) and the weight of absorbent gel (WS) as follows: swelling ratio (SR) (%) = 100 × (WS – W0)/W0.
Figure 2. Schematic representation of CS NPs loaded CaAlg hydrogel preparation (A). SEM images of CS NPs loaded CaAlg hydrogel (B). FT-IR spectra of pure CS NPs, CaAlg hydrogel, simple mixture of pure CS NPs and CaAlg hydrogel, and CS NPs loaded CaAlg hydrogel (C). DLS analysis of the obtained CS NPs (D). Rheological flow curves of CS-CaAlg hydrogel and CS loaded NPs (E). In vitro swelling profile of CS NPs loaded CaAlg hydrogel and CS-CaAlg hydrogel in phosphate-buffered saline (pH 7.4 and pH 8.0 at 37 °C) for 24 h. Data are expressed as the mean ± standard deviation (n = 3) (F).
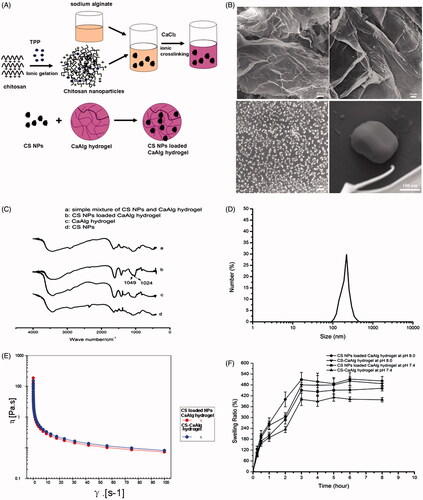
Antibacterial tests
Different amount of CS NPs loaded CaAlg hydrogel and CS-CaAlg hydrogel was placed into a tube and incubated with 5 mL of bacterial suspension containing 1 × 106 CFU of Escherichia coli and Staphylococcus aureus for continuous shaking for 24 h at 37 °C. Sterilized cotton swab was used to dip into bacteria suspension treated with both gels and slowly scratched on the LB-agar growth plates for incubation at 37 °C. The optical density (OD) was measured at 600 nm for determining the minimum bactericidal concentrations (MBCs) by using a microplate reader (Tecan, infinite M200, Männedorf, Switzerland). In addition, the OD at 260 nm was also evaluated for checking the leakage of DNA and RNA from bacteria.
ROS production measurement
According to our previous reports [Citation27], CS NPs loaded CaAlg hydrogel and CS-CaAlg hydrogel were co-incubated with HUVECs for 12 h at 37 °C with the treatment of 2,7-dichlorofluorescein diacetate (DCFH-DA, Sigma, St. Louis, MO, USA) with cells for about 30 min. Generation of ROS was detected by observing the intracellular DCF fluorescence using confocal laser scanning microscopy (FluoView FV10i, Olympus, Tokyo, Japan), and also quantified using microplate reader (Synery-2, Biotek, Winooski, VT, USA).
MTT assay
One hundred microlitres suspension of HUVECs at the logarithmic growth phase was added to each well in the 96-well plate and adjusted the cell concentration to 1 × 105 cells/well at 37 °C in a humidified atmosphere of 5% CO2, 95% air. One hundred microlitres of extracts of CS NPs loaded CaAlg hydrogel and CS-CaAlg hydrogel were added into each hole for incubating with cells at 37 °C in a humidified atmosphere of 5% CO2, 95% air. At different time interval, 20% MTT with concentration of 5 mg/mL was added to each well and continued to incubate for 4 h. One hundred and fifty microlitres of DMSO was added to each well, and the absorbance was measured at 490 nm.
Angiogenesis and confocal microscopy
The ECM gel solution was thawed out at 4 °C and 200 μL of mixture of ECM gel solution and serum free medium (v/v, 1:1) was added into each well of a 24-well plate. After incubation for 30 min at 37 °C, the ECM solution formed a gel. HUVECs (80 μL per well, about 1 × 105 cells/well) were cultured in gel loaded-bottom 24-well tissue culture plates followed by addition of the extracts of CS-CaAlg hydrogel and CS NPs loaded CaAlg hydrogel for continuous incubation for 8 h. The tube formation of HUVECs was observed and analysed using a confocal microscope (TCS SP8; Leica Microsystems, Wetzlar, Germany) and imaged at 100× magnification.
Scratch wound healing assay
HUVEC was cultured in DMEM supplemented with 10% FBS and seeded into 24-well tissue culture plate until they reach 70–80% confluence as a monolayer. A new 200 μL pipette tip was used to gently and slowly scratch a straight line to the monolayer across the centre of the well. After scratching, the wells were washed twice with medium to remove the detached cells followed by replenishing the wells with fresh medium. Extracts of CS NPs loaded CaAlg hydrogel and CS-CaAlg hydrogel containing the different concentration of CS were cultured in a tissue culture incubator at 37 °C. At different time interval, the cells were washed twice with PBS, and the gap distance can be observed and quantitatively evaluated under the microscope.
Transwell cell migration assay
The invasion of HUVEC was detected by transwell method. Extracts of CS NPs loaded CaAlg hydrogel and CS-CaAlg hydrogel containing the different concentration of CS were placed into the transwell insert precoated with basement membrane matrigel and incubated with HUVEC for 24 h in the 37 °C, 5% CO2 incubator. At different time interval, insert was removed from the chamber and chamber was turned upside-down and gently submerged in PBS several times to remove unattached cells. Cells were fixed by submerging insert in 4% paraformaldehyde for 20 min and stained with 1% crystal violet for 30 min (or overnight). Cells were removed from unmigrated (top) side by gently scrapping this side with a wet Q-tip/cotton swab. Invasiveness was determined by counting cells on the lower surface of the filter.
Western blot assay
According to the protocol [Citation27], extracts of CS NPs loaded CaAlg hydrogel and CS-CaAlg hydrogel containing concentration of CS at 10 and 50 μg/mL as well as the combination of NAC and CS NPs loaded CaAlg hydrogel were incubated with HUVECs for 48 h followed by washing twice with ice-cold PBS. Finally, the level of the targeted proteins was photographed and analysed by UVP gel analysis system.
Experimental wound model and wounding procedure
Six- to eight-week-old female SD mice were purchased from Beijing Vital River Laboratory Animal Technology Company. All procedures were performed by the guidelines of National Institutes of Health Guide for the Care and Use of Laboratory Animals and were approved by the Animal Care and Use Committee of Jinzhou Medical University. Using a dermatological pencil, a circle with a diameter of 2 cm was drawn on the back skin, and the skin was cut using a scalpel and scissors. Wound in all animals was treated with PBS, CS NPs loaded CaAlg hydrogel and CS-CaAlg hydrogel separately twice daily for consecutive days for two weeks. Wound area reducing curves were determined by calculating the ratio of the initial area and the wound area at the different time. The mice were sacrificed, then the area around the scar or residual wound were isolated and the wound specimens were prepared as paraffin-embedded sections for histopathological analysis.
Results and discussion
Physicochemical characterization of hydrogels
In this study, we employed a Ca(2+) cations as the crosslinker to fabricate a hydrogel system by dispersing CS nanoparticles into alginate aqueous solution, and the solution was formed into a hydrogel using CaCl2. We observed that CS NPs we prepared were monodisperse spheres, and further analysis revealed that the diameters of NPs were about 208.4 ± 15.7 nm and positive zeta potential about 24.2 ± 3.9 mV (). In addition, the polydispersity index of NPs was above 0.019, indicating the homogenous monodispersion and good stability in media. The investigation of the hydrogel microstructure was performed by scanning electron microscopy (SEM). CS NPs loaded CaAlg hydrogel showed tight stack vertically and had a low porosity, whereas it showed a considerable amount of CS nanoparticles (diameter around 200 nm) at the surface of hydrogel (). The possible interaction between CS NPs and CaAlg hydrogel was investigated via FTIR spectroscopic analysis of pure CS NPs, CaAlg hydrogel, simple mixture of pure CS NPs and CaAlg hydrogel, and CS NPs loaded CaAlg hydrogel. It is observed in that compared with the spectra of t simple mixture of pure CS NPs and CaAlg hydrogel, the appearance of two intense and sharp peaks at 1049 and 1024 cm−1 in the spectra of CS NPs loaded CaAlg hydrogel indicated CS NPs with the functional groups such as –NH and –OH as hydrogen bonding donors formed hydrogen bond at the surface of CaAlg hydrogel with the functional groups such as carboxylic group as hydrogen bonding acceptors. Therefore, the carbon–oxygen electron cloud density on the carboxyl group was increased, and stretching vibration from –C–O was strengthened and appeared at 1049 and 1024 cm−1. A flow curve shown in is presented by measuring the viscosity of developed gels from 0.01 to 100 s–1 of shear rate. The results showed that CS NPs loaded CaAlg hydrogel could be easily spread and located in wound site. With the initial minutely increase of shear rate, viscosity of both gels decreased rapidly. When the shear rate was increased gradually, the gel viscosity decreased slowly, indicating that the gels showed the shear thinning property and the viscosity of all the prepared gels decreased with increase in shear rate. The results in showed that both gels depended on the variation of time required to control the absorbing amount of water. The swelling process of CS NPs loaded CaAlg hydrogel and CS-CaAlg hydrogel showed a similar swelling pattern. The SR of both gels was increased with the enhancement of pH of medium. It further indicated that when pH was increased, the anionic carboxyl group was highly ionized and tend to charge repulsion and hydration, thus enhancing hydrogel swelling.
Antibacterial activities of CS NPs loaded CaAlg hydrogel
The results in show that compared to the control and CS-CaAlg hydrogel, CS NPs loaded CaAlg hydrogel showed the highest anti-bacteria activity. It is demonstrated in that with the increasing addition of CS NPs, it could kill two more kinds of bacteria, which may be part of the reason for the remarkable antibacterial activities. It indicated that positive charged CS NPs released from gel adhered to the negative charged surface of E. coli and S. aureus cells with a high probability. A strong interaction with both Gram-positive and Gram-negative bacteria by electro-static interaction might be a key reason that explains the effective antibacterial activity of CS NPs loaded CaAlg hydrogel. The MBCs quantitatively evaluated the antibacterial activities and were measured by determining the OD600. As shown in , the resulting gel exhibited remarkable antibacterial activities at very low dosages, with an MBC of 100 μg/mL against E. coli and against S. aureus. As shown in , the amount of DNA and RNA released from the bacteria was significantly higher in the CS NPs loaded CaAlg hydrogel group than the CS-CaAlg hydrogel group; CS NPs loaded CaAlg hydrogel group also exhibited an increased release of bacterial DNA and RNA with the increasing addition of CS NPs. The results above revealed that CS NPs loaded CaAlg hydrogel group killed G– bacteria (E. coli) and G + bacteria (S. aureus) by changing the membrane permeability, while the disruption in the cell wall was the primary mechanism of action on bacteria.
Figure 3. Photographs of culture plates of E. coli and S. aureus after exposure to CS NPs loaded CaAlg hydrogel containing different concentration of CS NPs (A). Photographs of the culture plates of E. coli and S. aureus after exposure to CaAlg hydrogel as well as CS-CaAlg hydrogel and CS NPs loaded CaAlg hydrogel with the same concentration of CS at 50 μg/mL (B). OD600 nm of bacterial suspension treated CS NPs loaded CaAlg hydrogel with different concentrations of CS NPs. These data represent three separate experiments and are presented as the mean values ± SD. *p < .05 versus OD600 nm of E. coli treated with control group, #p < .05 versus OD600 nm of S. aureus treated with control group. (C). Relative release of 260 nm absorbing materials from CS-CaAlg hydrogel and CS NPs loaded CaAlg hydrogel treated E. coli and S. aureus. These data represent three separate experiments and are presented as the mean values ± SD. *p < .05 CS-CaAlg hydrogel group versus control group, #p < .05 CS NPs loaded CaAlg hydrogel versus control group (D).
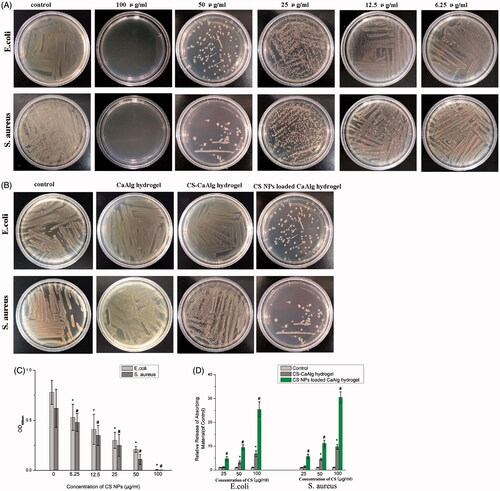
ROS determination
The results showed that compared with control group and CS-CaAlg hydrogel group, CS NPs loaded CaAlg hydrogel induced the massive generation of ROS (). With the increasing amount of CS at the concentration from 20 to 100 μg/mL, green fluorescence intensity also continued to rise gradually and the productions of intracellular ROS were increased from 112.3% to 124.5% in HUVECs treated with CS-CaAlg hydrogel. On the contrast, when HUVECs were treated with CS NPs loaded CaAlg hydrogel with the different concentration of CS, green fluorescence intensity was significantly enhanced and the productions of intracellular ROS were increased from 125.3% to 189.8%. Addition of 10 mM oxygen free radical scavenger acetylcysteine (NAC) inhibited the production of oxygen free radical, thus the intensity of intracellular green fluorescence was weakened and amount of ROS was significantly reduced to 110.0% in cells. It indicated that CS NPs loaded CaAlg hydrogel enhanced the uptake of CS NPs and increased ROS production.
Figure 4. ROS generation in cells treated with extracts of CS NPs loaded CaAlg hydrogel and CS-CaAlg hydrogel containing low concentration of CS at 20 μg/mL and high concentration at 100 μg/mL as well as the combination of NAC and CS NPs loaded CaAlg hydrogel in HUVEC cells. *p < .05, versus ROS levels of untreated cells at 24 h, the scale bar is 100 μm and applies to all figure parts.
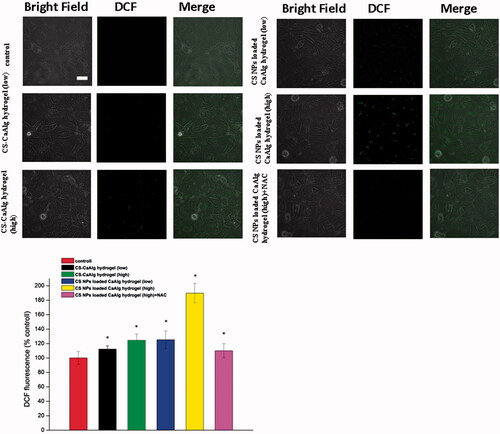
MTT assay and angiogenesis analysis
The MTT results shown in demonstrated that CS-CaAlg hydrogel and CS NPs loaded CaAlg hydrogel at a certain concentration of CS (ranging from 5 to 100 μg/mL) did not show cytotoxicity and the promotion on the proliferation of HUVECs induced by CS-CaAlg hydrogel and CS NPs loaded CaAlg hydrogel was not obvious, and the viability ratios in cells treated with CS NPs loaded CaAlg hydrogel at a certain concentration of CS at 10 and 100 μg/mL were 121.3% and 113.4% at 24 h, respectively.
Figure 5. Tube formation in HUVECs treated with extracts of CS NPs loaded CaAlg hydrogel and CS-CaAlg hydrogel containing low concentration of CS at 20 μg/mL and high concentration at 100 μg/mL as well as the combination of NAC and CS NPs loaded CaAlg hydrogel in HUVECs (A). HUVEs viability cultured with CS NPs loaded CaAlg hydrogel (B) and CS-CaAlg hydrogel (C) containing different concentrations of CS after 24 h. Data are presented as means ± SD (n = 3).
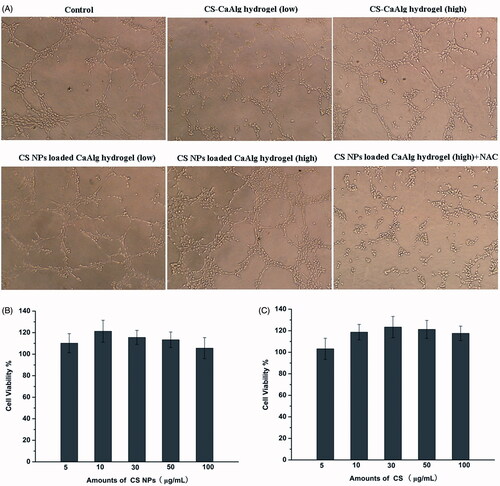
Angiogenesis played a normal and vital process in wound healing process and capillary-like tube formation of HUVEC formed new blood vessels from preexisting vessels and facilitated the formation of granulation tissue. The results in show that compared with control and CS-CaAlg hydrogel, tube formation in the presence of CS NPs loaded CaAlg hydrogel was significantly accelerated and the branch numbers of connected cells in randomly selected fields depended on a dose to significant increase. On the contrary, treatment with the combination of CS NPs loaded CaAlg hydrogel and NAC as oxygen free radical scavenger inhibited tube formation of HUVEC on Matrigel. These results suggested that CS NPs loaded CaAlg hydrogel promoted in vitro angiogenesis by enhancing tube formation. Although CS NPs loaded CaAlg hydrogel did not show the obvious proliferation of HUVEC cell, blood vessel recruitment may be mainly by cell migration rather than proliferation of HUVECs. Angiogenesis induced by CS NPs loaded CaAlg hydrogel was primarily regulated by the generation of ROS.
Cell migration and invasion assay
As shown in , compared with the control group, the migration distance of cells treated with the extracting solutions of CS-CaAlg hydrogel and CS NPs loaded CaAlg hydrogel was higher than that of the control group and showed the dose dependent pattern. Especially, CS NPs loaded CaAlg hydrogel induced the higher cell migration and migration rates in contrast with cells treated with CS-CaAlg hydrogel. It indicated that CS NPs were loaded into the matrix of hydrogel and further released, finally internalized into the HUVEC, thus accelerating the migrating rate of cells. With the increasing amount of CS at the concentration of 20 and 100 μg/mL, migrated distance also continued to rise gradually from 8 to 34 μm in cells treated with CS-CaAlg hydrogel. On the contrast, when cells were treated with CS NPs loaded CaAlg hydrogel with CS NPs at the concentration of 20 and 100 μg/mL, migrated distance was significantly enhanced from 40 to 84 μm. Interestingly, with the addition of NAC as an inhibitor of oxygen free radical, the degrees of cell invasion and migration were significantly reduced in contrast with cells treated with non-NAC. It suggested that NPs-mediated ROS generation could be associated with the induction of cell migration. The cell invasion assay results also confirmed that compared with cells treated with CS-CaAlg hydrogel, the number of CS NPs loaded CaAlg hydrogel treated cells on the underside of the membrane was significantly increased, indicating that cells secreted more protease to damage the ECM, therefore enhancing the invading force of cells. In the same way, the addition of NAC effectively prohibited cell invasion and migration. It also proved that CS NPs loaded CaAlg hydrogel promoted cell invasion and metastasis via the massive production of ROS.
Figure 6. CS NPs loaded CaAlg hydrogel promoted migration and invasion of HUEVCs. Image (A) and quantity (C) of migration and image (B) and quantity (D) of invasion taken from HUEVC cells incubated for 24 h with extracts of CS NPs loaded CaAlg hydrogel and CS-CaAlg hydrogel containing low concentration of CS at 20 μg/mL and high concentration at 100 μg/mL as well as the combination of NAC and CS NPs loaded CaAlg hydrogel (magnification, 100×). The above assays were quantified. Results were expressed as mean ± SD (n = 3). *p < .05, versus untreated cells at 24 h.
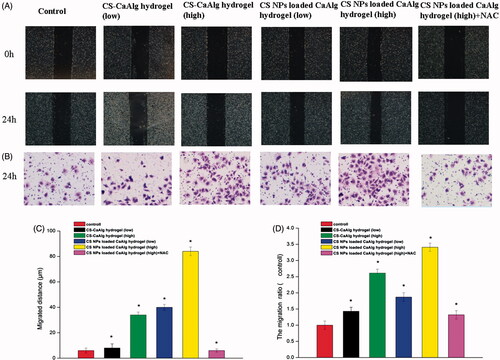
Western blot assay
Interleukin 6 (IL-6) acts as both a pro-inflammatory cytokine and an anti-inflammatory myokine [Citation28]. The results in show that compared with CS-CaAlg hydrogel, CS NPs loaded CaAlg hydrogel showed the higher potential on promoting the acute synthesis and secretion of IL-6 in HUVEC within the initial 12 h of wound injury period in a dose-dependent manner, suggesting that it had potential acute proinflammatory activation and was conducive to wound tissue aggregation, sterilization. After using ROS scavenger, IL-6 inflammasome signal activation was significantly suppressed, indicating the activation of IL-6 mediated inflammasome was partially ROS dependent. Within 48 h, the expression level of IL-6 in HUEVCs treated with CS NPs loaded CaAlg hydrogel was significantly decreased, suggesting that lower expression of IL-6 attenuated the pain, inhibited the prolonged inflammatory response, and improved the pathologic condition induced by wound injury. CS NPs loaded CaAlg hydrogel activated a ROS-dependent oxidative stress response and secretion of proinflammatory cytokines in HUVEC in the initial period of wound healing process.
Figure 7. Expression levels of IL-6 protein in HUVEC treated with CS NPs loaded CaAlg hydrogel containing low concentration of CS at 20 μg/mL, middle concentration of CS at 50 μg/mL and high concentration at 100 μg/mL for 12 h (A). Western blot analyses of the expression levels of IL-6, VEGF, MMP-2 and Bcl-2 in HUVEC following treatment with CS NPs loaded CaAlg hydrogel for 48 h (B).
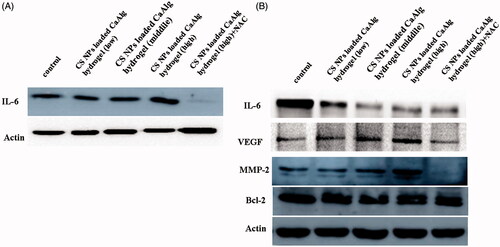
To further justify the effect of CS NPs loaded CaAlg hydrogel on angiogenesis in wound healing process, we detected the expression of VEGF, MMP-2 and bcl-2 as the primary neovascularization-relevant proteins via western blot analysis. The results showed that CS NPs loaded CaAlg hydrogel and CS-CaAlg hydrogel had no significant difference on bcl-2 as anti-apoptotic protein in HUVEC, illustrating that compared with the control groups, both gels did not induce the cell apoptosis. In order to further evaluate invasion and metastasis of cells treated with gels, the expressions of proteins of the matrix metalloproteinase (MMP) family were investigated by western blot experiment. It demonstrated that CS NPs loaded CaAlg hydrogel induced the highest expression of mmp-2 in HUVEC in contrast with control and CS-CaAlg hydrogel. The expressions of mmp-2 were significantly increased with the increasing amount of added CS NPs in gels. Combination of NAC and CS NPs loaded CaAlg hydrogel down-regulated the expression levels of mmp-2, suggesting that CS NPs loaded CaAlg hydrogel facilitated the secretion of mmp-2 as main migration-relevant proteins and accelerated the breakdown of ECM in physiological processes. The results also confirmed that there was a possibility that NPs promoted the invasion and metastasis of cells via NPs mediated ROS production. The inhibition of ROS with NAC significantly reduced the degree of cell invasion and migration. Finally, CS NPs loaded CaAlg hydrogel induced the highest expression of VEGF in HUVEC in contrast with control and CS-CaAlg hydrogel. Combination of NAC and CS NPs down-regulated the expression levels of VEGF by reducing ROS. It revealed that increased intracellular ROS induced the activation of VEGF on the cell membrane to initiate the phosphorylation of the redox-sensitive signalling transduction molecules such as P38 and ERK, leading to proliferation, migration and adhesion of VECs and thus promoting cell angiogenesis effects.
Full-thickness skin wounds and quantification of healing
CS-CaAlg hydrogel and CS NPs loaded CaAlg hydrogel were applied to the wounds twice daily, and wound size was quantified from images taken on post-wounding days 1, 2, 3, 7, 9, 12 and 14. The results in show that the rate of wound closure in mice treated with CS NPs loaded CaAlg hydrogel was significantly faster than control group and CS-CaAlg hydrogel treated group. When injury wound were treated with CS NPs loaded CaAlg hydrogel, the rate of wound closure was increased to 85.6% within seven days and 98.7% within 14 days, respectively. Similarly, after being treated with PBS and CS-CaAlg hydrogel, the rates of wound closure were lower and valued at 63.6% and 69.9% within seven days, and 81.5% and 95.7% within 14 days, respectively. The results further confirmed that CS NPs loaded CaAlg hydrogel promoted wound contraction and accelerated healing of wounds.
Figure 8. Photographs of the appearance of the wounds on days 7 and 14 under the treatment of CS-CaAlg hydrogel and CS NPs loaded CaAlg hydrogel (A). Wound closure curves after wound sites were treated by CS-CaAlg hydrogel and CS NPs loaded CaAlg hydrogel (B). The results were expressed as mean ± SD (n = 5).
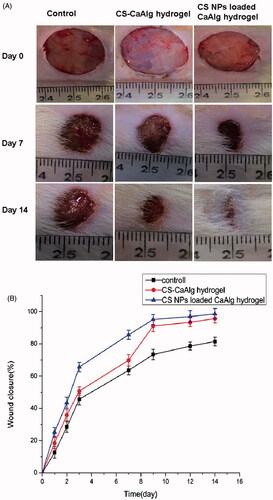
Histological observations () revealed that after treatment from 1 day to 3 days, in the control group, there were a large amount of inflammatory cells infiltrating in wound site, granulation tissue layer structure was still loose and few fibroblasts were formed and arranged impacted. Compared with CS-CaAlg hydrogel, CS NPs loaded CaAlg hydrogel did not show acute inflammatory reaction and a small amount of neutrophils and some inflammatory cells were observed in wound site. Moreover, CS NPs loaded CaAlg hydrogel exhibited significant formation of fibroblast, granulation tissue and blood vessel at the wound site. After treatment from 7 days to 14 days, in the control group, the wound was not completely closed and reepithelialization was not completed, the thinner epithelium was formed and more neutrophil infiltration was visible around the border of epithelium. The structure of granulation tissue with more neovascularization and fibrous cells still existed. In the wound site treated with CS-CaAlg hydrogel, new epidermis and a scab were obviously formed. CS-CaAlg hydrogel induced the formation of thicker epithelium containing the infiltration of a certain number of inflammatory cells, high granulation tissue content and a few regenerative blood vessels. When CS NPs loaded CaAlg hydrogel was treated in wound site, we found completely closed wound with complete reepithelialization. Fibroblasts and collagen were arranged orderly and only a very small amount of inflammation cells existed. The majority of granulation tissue and blood vessels was subsided, tending to normal skin tissue.
Figure 9. H&E staining of tissue sections treated with CS NPs loaded CaAlg hydrogel on days 1, 3, 7 and 14 (A). Number of inflammatory cells (B), blood vessels (C) and fibroblasts (D) on days 1, 3, 7 and 14. Data represent means ± SD (n = 3). #p < .05, CS-CaAlg hydrogel group versus the corresponding control group at the same day. *p < .05, CS NPs loaded CaAlg hydrogel group versus the corresponding control group at the same day.
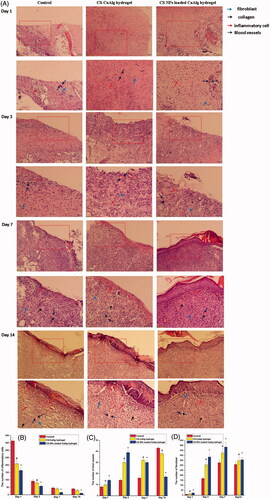
Conclusions
In this study, CS NPs loaded CaAlg hydrogel was prepared by dispersing CS nanoparticles into the matrix of homogeneous alginate hydrogels cross-linked with Ca(2+) cations. The resulting gel exhibited remarkable antibacterial activities at very low dosages, with an MBC of 100 μg/mL against E. coli and against S. aureus. In vivo studies on skin wounds in mice showed that CS NPs loaded CaAlg hydrogel promoted proliferation and migration of VEC induced by the production of ROS, thus leading to rapid skin wound healing and re-epithelialization. The hydrogel induced the enhancement on the initial inflammation reaction in skin wounds in vivo, thus was conducive to debridement and anti-infection effect. Our results suggest that the application of bioactive CS NPs loaded CaAlg hydrogel with the ability to generate the massive production of ROS was an effective way to design multifunctional hydrogel for accelerating acute wound healing effects.
Acknowledgements
English-language editing of this manuscript was provided by Journal Prep.
Disclosure statement
The authors report no conflicts of interest in this work.
Additional information
Funding
References
- Martin P. Wound healing-aiming for perfect skin regeneration. Science. 1997;276:75–81.
- Gurtner GC, Werner S, Barrandon Y, et al. Wound repair and regeneration. Nature. 2008;453:314–321.
- Grose R, Werner S. Wound-healing studies in transgenic and knockout mice. Mol Biotechnol. 2004;28:147–166.
- Springer TA. Traffic signals for lymphocyte recirculation and leukocyte emigration, the multistep paradigm. Cell. 1994;76:301–314.
- Mayadas TN, Johnson RC, Rayburn H, et al. Leukocyte rolling and extravasation are severely compromised in P selectin-deficient mice. Cell. 1993;74:541–554.
- Barrientos S, Stojadinovic O, Golinko MS, et al. Growth factors and cytokines in wound healing. Wound Repair Regen. 2008;16:585–601.
- Chen X, Zhang M, Wang X, et al. Peptide-modified chitosan hydrogels promote skin wound healing by enhancing wound angiogenesis and inhibiting inflammation. Am J Transl Res. 2017;9:2352–2362.
- Tadokoro S, Ide S, Tokuyama R, et al. Leptin promotes wound healing in the skin. PLoS One. 2015;10:e0121242.
- Belvedere R, Bizzarro V, Parente L, et al. The pharmaceutical device Prisma® skin promotes in vitro angiogenesis through endothelial to mesenchymal transition during skin wound healing. Int J Mol Sci. 2017;18:E1614.
- Huang H, Kong D, Liu Y, et al. Sapylin promotes wound healing in mouse skin flaps. Am J Transl Res. 2017;9:3017–3026.
- Buico A, Cassino C, Ravera M, et al. Oxidative stress and total antioxidant capacity in human plasma. Redox Rep. 2009;14:125–131.
- Yoon SO, Park SJ, Yoon SY, et al. Sustained production of H(2)O(2) activates pro-matrix metalloproteinase-2 through receptor tyrosine kinases/phosphatidylinositol 3-kinase/NF-kappa B pathway. J Biol Chem. 2002;277:30271–30282.
- Park SW, Jun HO, Kwon E, et al. Antiangiogenic effect of betaine on pathologic retinal neovascularization via suppression of reactive oxygen species mediated vascular endothelial growth factor signaling. Vascul Pharmacol. 2017;90:19–26.
- Madigan MC, McEnaney RM, Shukla AJ, et al. Xanthine oxidoreductase function contributes to normal wound healing. Mol Med. 2015;21:313–322.
- Frey RS, Ushio-Fukai M, Malik AB. NADPH oxidase-dependent signaling in endothelial cells: role in physiology and pathophysiology. Antioxid Redox Signal. 2009;11:791–810.
- Hu YP, Peng YB, Zhang YF, et al. Reactive oxygen species mediated prostaglandin E2 contributes to acute response of epithelial injury. Oxid Med Cell Longev. 2017;2017:4123854.
- Hamdan S, Pastar I, Drakulich S, et al. Nanotechnology-driven therapeutic interventions in wound healing: potential uses and applications. ACS Cent Sci. 2017;3:163–175.
- Nguyen TH, Kim YH, Song HY, et al. Nano Ag loaded PVA nano-fibrous mats for skin applications. J Biomed Mater Res B Appl Biomater. 2011;96B:225–233.
- Silvestry-Rodriguez N, Sicairos-Ruelas EE, Gerba CP, et al. Silver as a disinfectant. Rev Environ Contam Toxicol. 2007;191:23–45.
- Nurhasni H, Cao JF, Choi M, et al. Nitric oxide-releasing poly(lactic-co-glycolic acid)-polyethylenimine nanoparticles for prolonged nitric oxide release; antibacterial efficacy; and in vivo wound healing activity. Int J Nanomed. 2015;10:3065–3080.
- Schairer DO, Martinez LR, Blecher K, et al. Nitric oxide nanoparticles: pre-clinical utility as a therapeutic for intramuscular abscesses. Virulence. 2012;3:62–67.
- Li T, Li F, Xiang W, et al. Selenium-containing amphiphiles reduced and stabilized gold nanoparticles: kill cancer cells via reactive oxygen species. ACS Appl Mater Interfaces. 2016;8:22106–22112.
- Latvala S, Hedberg J, Di Bucchianico S, et al. Nickel release, ROS generation and toxicity of Ni and NiO micro- and nanoparticles. PLoS One. 2016;11:e0159684.
- Wu YY, Zhang JH, Gao JH, et al. Aloe-emodin (AE) nanoparticles suppresses proliferation and induces apoptosis in human lung squamous carcinoma via ROS generation in vitro and in vivo. Biochem Biophys Res Commun. 2017;490:601–607.
- Angelé-Martínez C, Nguyen KV, Ameer FS, et al. Reactive oxygen species generation by copper(II) oxide nanoparticles determined by DNA damage assays and EPR spectroscopy. Nanotoxicology. 2017;11:278–288.
- Yu X, Yang G, Shi Y, et al. Intracellular targeted co-delivery of shMDR1 and gefitinib with chitosan nanoparticles for overcoming multidrug resistance. Int J Nanomedicine. 2015;10:7045–7056.
- Wang T, Hou J, Su C, et al. Hyaluronic acid-coated chitosan nanoparticles induce ROS-mediated tumor cell apoptosis and enhance antitumor efficiency by targeted drug delivery via CD44. J Nanobiotechnol. 2017;15:7.
- Liu L, Mu Q, Li W, et al. Isofraxidin protects mice from LPS challenge by inhibiting pro-inflammatory cytokines and alleviating histopathological changes. Immunobiology. 2015;220:406–413.