Abstract
The aim of the study was to synthesize nanoparticles (NPs) with chitosan (CS), and poly (lactic acid) (PLA) as a carrier for the drug piceatannol (PIC). The synthesized nanoparticles form the composite of polymeric-drug nanoparticles (CS/PLA-PIC NPs) by dropping method. The preliminary and stability studies were determined for the polymers drug-loading capacity and encapsulation efficiencies. The in vitro drug release study showed that NPs provided a continuous release of the entrapped PIC. The NPs found to be a good scavenger for DPPH, SOD and NO radicals. MTT and LDH assays revealed higher cytotoxic efficacy of CS/PLA–PIC NPs in HepG2, A549 and MCF7 cells compared to CS-PLA NPs and PIC. Dual staining results showed the early/late-stages of apoptotic and necrotic cells. Furthermore, cells treated with CS/PLA-PIC NPs showed fragmenting DNA and also demonstrated for apoptotic cells percentage by flow cytometry. These results suggested that upon CS/PLA–PIC NPs exposure leads to decrease in cancer cell viability due to apoptosis.
Introduction
The existences of the bioactive phyto-compounds have produced synergistic health benefits which lead to the development of novel treatments in the global struggle against cancer and cancer-related disorders. In current years, nanotechnology is being implemented and assessed in different ways to improve areas of cancer therapeutics and management [Citation1]. Therefore, it is essential to identify the potential delivery mechanism and explore more efficient cancer therapeutic strategies. A range of experiments has accounted that, in-taking of red wine in a limited amount which is high in antioxidant polyphenols with probable defensive behaviour, is associated with the lesser occurrence of numerous human diseases like carcinoma of the esophagus, prostate cancer, leukaemia, non-Hodgkin’s lymphoma and gastric cardia adenocarcinoma [Citation2–4]. The well-known plant polyphenols and their by-products are commercially available as a major source of resveratrol and piceatannol [Citation5].
Piceatannol (PIC), a polyphenol occurs in nature which is present in red wine. It is one of the major dietary stilbenes, a class of organic compounds possessing important health benefits that contains pterostilbene and resveratrol [Citation6]. It has been proved that PIC helps in preventing and treating cancer [Citation7]. Reports stated that PIC persuades an intrinsic approach for programmed cell death and also to treat cancers like bladder, prostate, melanoma and colorectal [Citation8–12]. PIC proves as an anti-inflammatory, antioxidant, anti-leukemic [Citation13] and as a drug for Epstein–Barr virus and also act as a recovery agent of cardiovascular diseases [Citation14], atherosclerosis and inflammatory bowel diseases and gives protection against beta-amyloid stimulated oxidative stress and cell death [Citation15]. As mentioned in our previous article, there was a hasty glucuronidation and deficit of PIC bioavailability when injected in rats, hence the encapsulation of polymeric nanoparticles overcomes bioavailability against the target cells [Citation16]. Polymeric nanoparticles that are originated from the biodegradable and biocompatible polymers are good candidates for drug carrier to deliver drugs because they are predictable to be adsorbed in an intact form in the gastrointestinal tract after oral administration.
Chitosan (CS) is the second generous linear polysaccharide and a cationic polyelectrolyte present in nature. CS has depicted convenient biocompatibility and increasing membrane permeability characteristics both in vitro and in vivo and can be degraded by lysozyme in serum. On account of its mucoadhesive and permeability, CS has the potential of serving as an adsorption enhancer across intestinal epithelium and it was an illustrated biopharmaceutical fact. In our previous report [Citation16], we have discussed the reason of combining CS with synthetic polymer. Though CS has many beneficial properties, the drawback is that a reactive hydroxyl and amine groups which undergo potential modifications tend to results in graft reaction. When introduced in vivo it has been digested by the enzymes such as lysozyme and chitosanase into oligomers and promote to N-glucosamine [Citation17]. To overwhelm this drawback and to improve the polymer stability, synthetic polymer was combined with CS to form novel drug delivery systems.
Biodegradable synthetic polymers that are extensively used including poly (lactic acid) (PLA), poly (glycolic acid) (PGA) and their copolymers. PLA is a hydrophobic crystalline structured compound which is insoluble in water, but it is utterly soluble in organic liquids like ethyl acetate, dichloromethane or chloroform. Comparing to other synthetic polymers such as polystyrene, polypropylene, PLA also has a high mechanical confrontation that forms porous nano and micro-structures [Citation18]. PLA is being broadly used as drug delivery vehicle, in tissue engineering and surgical sutures. Apart from the beneficial characteristics of PLA, it also has a drawback in lack of biological activity on the cells [Citation19]. PLA forms an intact mould when combined with CS which will help for the targeted drug delivery. Overall, this study involves the in vitro apoptotic activity of the novel drug PIC encapsulated with polymeric nanoparticles (CS-PLA NPs).
Materials and methods
Materials
Chitosan (Mw = 90 kDa), poly (lactic acid) (Mw= 60 kDa), piceatannol (Mw= 244.24), DPPH, MTT, LDH assay kit, RNAse A, proteinase K, acridine orange, ethidium bromide, RPMI-1640 medium were purchased from Sigma Aldrich, USA. Apoptosis detection kit was purchased from Biolegend, USA. All other chemicals used were of analytical reagent grade and without further purification. Ultra-pure water was used for the preparation of all solutions. A549, HepG2, and MCF7 cell lines were purchased from National Centre for Cell Science, Pune, India. The cell line was grown in Dulbecco’s Modified Eagle’s Medium supplemented with 2 mM L-glutamine, 100 U/ml penicillin, 100 g/ml streptomycin and 10% foetal bovine serum (FBS). Cells were cultured in 75-cm2 cell culture flasks at 37 °C in a 5% CO2 atmosphere.
Preparation of CS/PLA-PIC NPs by dropping method
CS–PLA nanoparticles were prepared by mixing CS and negatively charged PLA by dropping method which was previously described in our article using the drug anthraquinone [Citation20] with slight modification. Briefly, 2 ml of 0.02% CS solution was dissolved in 1% (w/v) acetic acid solution, and it was added drop wise into 10 ml of 0.02% PLA aqueous solution which was dissolved in acetone and stirred under hot water bath for 1 h at 50 °C, to obtain an opalescent suspension. The suspension obtained was filtered by a filter paper; the filtered suspension was incubated in a buffer solution of pH 4.5 for 24 h using a dialysis membrane for further analysis. 10 mg of PIC was added to 10 ml of CS-PLA mixture and incubated for 48 h. These nanoparticles were separated by centrifugation at 15,000 rpm for 30 min. The obtained suspension was freeze-dried and used for further analysis. The synthesized nanoparticles were determined for FTIR, SEM, zeta sizer and zeta potential which was reported in our previous study [Citation16].
Entrapment and drug loading efficiencies
A known quantity (2 mg) of a lyophilized sample which contained 2 mg of CS-PLA NPs and 10, 15, 20 µg of PIC was taken in 10 ml of ethanol; the solution was sonicated thoroughly using a probe sonicator (Frontline 30 W) for 5 min. The resulting solution was centrifuged at 20,000 rpm for 15 min and the supernatant was collected. Amount of drug within the supernatant was quantified spectrophotometrically at an absorption maximum of 524 nm, which corresponds to the peak absorption of PIC (UV-1700 Spectrophotometer, SHIMADZU, Japan). Entrapment efficiency was calculated based on the ratio of the amount of drug present in the NPs to the amount of drug used in the loading process. Loading efficiency (LE) of the drug-loaded system was also calculated with respect to the yield of the nanoparticles obtained after centrifugation.
Stability study
The stability of drug-loaded nanoparticles was dogged in the terms of its drug content with slight modification in the protocol [Citation21]. The firmness of nanoparticles was evaluated in PBS (pH-7). The prepared nanoparticles were incubated at 4 °C for a period of 2 months. At particular time interval, the suspension was centrifuged at 20,000 rpm for 15 min, the supernatant was collected to evaluate the amount of drug. Drug loading, and encapsulation efficiency was detected by UV spectrophotometer (UV-1700 Spectrophotometer, SHIMADZU, Japan) at 524 nm.
In vitro drug release study
In vitro drug release profile of PIC from drug-loaded CS-PLA nanoparticles was done by direct dispersion method. In vitro drug release studies were done for a period of 6 days. A known quantity of CS-PLA NPs (2 mg) and different concentration of PIC were taken in 30 ml 10 mM PBS and dispersed in 3 sets of tubes. The tubes were then incubated in a shaker water bath at 37 °C. At definite time intervals, one set of the tube was taken out and centrifuged at 1200 rpm for 3 min to pelletize the released drug, leaving the entrapped drug within nanoparticles which stay in the supernatant. The pellets were dissolved in 4 ml ethanol and an amount of drug released was quantified spectrophotometrically (UV-1700 Spectrophotometer, SHIMADZU, Japan) at a wavelength of 524 nm. The in vitro drug release was calculated.
Antioxidant assays
DPPH Scavenging Activity
DPPH was carried out with different concentrations (5, 10, 20, 40 and 80 µg/ml) of CS-PLA NPs, PIC, and CS/PLA-PIC NPs. The absorbance at 590 nm was measured against a blank (ethanol) using UV-1700 Spectrophotometer, SHIMADZU, Japan. Quercitin was used as positive control. The experiment was done in triplicates.
Superoxide dismutase (SOD) radical scavenging activity
SOD was carried out with different concentrations (5, 10, 20, 40 and 80 µg/ml) of CS-PLA NPs, PIC and CS/PLA-PIC NPs. Absorbance was measured at 590 nm on UV-1700 Spectrophotometer, SHIMADZU, Japan. Quercitin was used as positive control. The experiment was done in triplicates and calculated [Citation22].
Nitric-oxide (NO) radical scavenging activity
NO was carried out with different concentrations (5, 10, 20, 40 and 80 µg/ml) of CS-PLA NPs, PIC and CS/PLA-PIC NPs. The absorbance was measured at 546 nm on UV-1700 Spectrophotometer, SHIMADZU, Japan. Quercitin was used as positive control. The experiment was done in triplicates and calculated [Citation23].
Cytotoxicity assays
MTT assay
A549, HepG2 and MCF7 cells were cultured and seeded into 96-well plates approximately as 5 × 105 cells in each well and incubated for 48 h. Cells were treated with different concentrations of synthesized CS-PLA NPs, PIC and CS/PLA-PIC NPs (1, 5, 10, 20 and 40 µg/ml). After treatment, the plates were incubated for 24 h to perform cytotoxic analysis using MTT assay. MTT (3–(4,5-dimethylthiazol-2-yl)-2,5-diphenyltetrazolium bromide, a yellow tetrazole) was prepared at a concentration of 5 µg/ml and 10 ml of MTT was added to each well and incubated for 4 h. Purple colour formazone crystals that formed were then dissolved in 100 ml of dimethyl sulphoxide (DMSO). These crystals were observed at 570 nm on UV-visible spectrometer Shimadzu, Japan. Results were given as mean ± standard deviation of three independent experiments.
LDH assay
One millilitre of cells (A549, HepG2 and MCF7) at a density of 5 × 105 cells/ml (Dulbecco’s modified Eagle’s medium containing 10% FBS) were seeded in each well of 12-well plates and grown for 48 h before nanoparticles exposure. The cells were washed and dosed with different concentrations of synthesized CS-PLA NPs, PIC, and CS/PLA-PIC NPs (1, 5, 10, 20 and 40 µg/ml). After 24-h exposure, the 12-well plates were shaken briefly to homogenize the released LDH in the cell culture medium and the medium was transferred to 1.5 ml microcentrifuge tubes and was centrifuged at 12,000 x g at 4 °C for 15 min to remove any cell debris. 100 μl of each concentration of CS-PLA NPs, PIC and CS/PLA-PIC NPs was added to the substrate solution and the absorbance was measured at 570 nm on UV-visible spectrometer Shimadzu, Japan. The experiment was repeated in triplicates.
Cell death assays
DNA fragmentation
A549, HepG2 and MCF7 cells (5 × 105 cells/ml) were seeded in 6-well microplates and treated with IC30 and IC50 of CS/PLA-PIC NPs. After 24 h of the treatment, the culture medium was removed, and the cells were harvested by scraping with 1 ml of PBS and lysed with 500 μl of lysis buffer, 20 mM Tris-HCl (pH 8), 5 mM EDTA, 400 mM NaCl, 1% SDS, and 10 mg/ml proteinase K for 1 h at 55 °C. Fragmented DNA was extracted with phenol/chloroform/isoamyl alcohol (25:24 : 1 v/v/v), precipitated with ethanol, and suspended in Tris-EDTA buffer (TE, pH 8) containing 20 μg/ml RNase A. For quantitative analyses of DNA content, an equal amount of DNA was loaded and run on a 1% agarose gel containing 1 μg/ml ethidium bromide at 70 V, and the DNA fragments were visualized by exposing the gel to ultraviolet light, followed by photography. Control was maintained only with 24 h gown cell cultures.
Analysis of apoptotic cell death by fluorescence method
A549, HepG2 and MCF7 cells (5 × 105 cells/ml) were added to IC30, IC50 and IC90 concentrations of CS/PLA-PIC NPs. When cells had sloughed off, suspensions (25 μl) were transferred to glass slides. Dual fluorescent staining solution containing 100 μg/ml acridine orange (AO) and ethidium bromide (EB) dyes was added to each suspension and then covered with a coverslip. The morphology of apoptotic cells was examined and 500 cells were counted within 20 min using an inverted fluorescent microscope (Ti-Eclipse). This experiment was repeated thrice.
Annexin V/propidium iodide staining for apoptotic cells
A549, HepG2 and MCF7 cells were stained with annexin-V and propidium iodide for determining the apoptotic activity for CS/PLA-PIC NPs using fluorescence-activated cell sorting (FACS) analysis. Cells were seeded at 2.5–5.0 × 105 cells per 60 mm plate and incubated until 80% confluence. Cells were treated with the IC50 concentration of CS/PLA-PIC NPs. Cells that were incubated with complete growth culture medium served as control. After 24 h incubation, cells were harvested by quick trypsinization to minimize potentially high annexin-V background levels in adherent cells, washed twice with cold PBS and stained with annexin-V/fluorescein 5(6)- isothiocyanate (FITC), and propidium iodide (PI) in binding buffer. Stained cells were placed on ice and protected from light until reading via flow cytometry. Processed single cell suspensions were analysed on a FACS Calibur flow cytometer (BD, USA), with the laser excitation wavelength set at 488 nm. The green signal from FITC/annexin-V was measured at 525 nm and the red signal from PI was measured at 620 nm.
Results and discussion
Drug loading and encapsulation efficiencies
The preliminary drug loading and encapsulation efficiencies studies were evaluated. The minimum drug-loading capacity of the synthesized drug was 23.6% and encapsulation efficiency was 78.4%, whereas the maximum drug-loading capacity was 35.8% and encapsulation efficiency was 91.2%. When the stability tests were carried out after 60 days of storage of CS/PLA-PIC NPs at 4 °C, the drug loading capacity decreased from 35.8 to 34.2% and drug encapsulation efficiency decreased from 91.2 to 90.1% (). The narrow difference in drug loading and encapsulation efficiency between 1st day and 6th day shows that the synthesized drug was stable. The slight decrease in value might be due to shrinkage of particles or saturation/degradation of the polymers when it is stored for a long time. The drug release is influenced by the amount of the drug loaded. It shows that greater the drug loaded quicker the drug will be released. From this result, it can be seen that the drug-loading methods have a significant effect on drug loading and encapsulation efficiency of the nanoparticles complex.
Table 1. Determination of drug and polymers.
In vitro drug release study
In vitro drug release was carried out for 6 days at pH 7. The results were showed that 18–20% of the PIC was released from CS-PLA NPs from day 2 (). The sustained release was 90, 78.3 and 59.1% in 2:20, 2:15 and 2:10 formulations over 6 days. The drug release which was plotted against time, it showed a linear relationship. The synthesized polymeric nanoparticles have attained sustained release for long period, which added the value for the patient in reducing the treating incidence. In general, the drug release of nanoparticles made from the polymers is controlled by the erosion of the polymer back bones in nanoparticles. However, the drug release of nanoparticles made from the slowly erodible polymers is controlled by both of polymer erosion and drug diffusion.
Antioxidant assays
DPPH activity
In the DPPH free radical scavenging activity, three formulations (CS-PLA NPs, PIC, and CS/PLA-PIC NPs) of the concentrations 5 to 80 µg/ml were evaluated for their free radical scavenging activity with quercitin as a standard compound. The scavenging effect increased with the increasing concentrations of the tested compounds. The DPPH activity of CS-PLA NPs ranged from 5 to 53.6% whereas PIC and CS/PLA- PIC NPs was 18% to 64.5% and 30.3% to 84.6%, respectively. Quercetin showed moderate to high scavenging abilities from 25.4% to 79.56% (). Thus, the antioxidant activity of CS/PLA-PIC NPs was found to be a good scavenger for DPPH radicals. CS/PLA-PIC NPs recognized as potent antioxidant activity and also a scavenger of DPPH. Free radical scavenging activity of CS/PLA-PIC NPs was established based on their ability to reduce the stable free radical DPPH to the yellow-colored diphenylpicrylhydrazine. CS/PLA-PIC NPs was more efficient in free radical scavenging activity than the standard antioxidant quercitin. The CS/PLA-PIC NPs showed noticeable scavenging activity and exhibited a stronger concentration-dependent inhibition of deoxyribose oxidation.
Superoxide dismutase activity
Riboflavin/light/NBT (Nitro blue tetrazolium) reduction test was used to measure in-vitro super oxide radical scavenging activity. The super oxide radical reduces NBT to a blue colored formazan that can be measured at 560 nm. PIC is electron donor due to the presence of electron donating substituents groups like –OH, -CL and –CH3 at different positions of PIC. The SOD activity of CS-PLA NPs ranged from 2 – 43.76% whereas PIC and CS/PLA- PIC NPs was 14% to 58.6% and 17.3% to 76.5%, respectively (). Quercetin showed moderate to high scavenging abilities from 25.4% to 79.56%. Even though superoxide is a moderately weak oxidant, it festers to form strapping reactive oxidative species, such as single O2 and hydroxyl radicals, which initiate lipid peroxidation.
Nitric oxide scavenging activity
This activity possesses the reductive potential of three combinations (CS-PLA NPs, PIC and CS/PLA-PIC NPs) and quercitin exhibited dose-dependent activity. Nitric oxide scavenging effect of CS/PLA-PIC was 88.25% at the concentration of 80 µg/ml, whereas scavenging effect of quercitin was 86.5% at the same concentration. The activity of PIC (5 to 80 µg/ml) was found to be 19.2% to 63.9% higher than CS-PLA NPs (7.2% to 56.8%). Results are graphically represented (). From the graph, it was observed that as concentration increases, the percentage of scavenging is also increasing linearly for all three formulations and quercitin (standard preparation). From results, it was confirmed that the CS/PLA-PIC NPs showed potent antioxidant activity than CS-PLA NPs and PIC. CS/PLA-PIC NPs showed good antioxidant activity than quercitin. Free radicals such as nitrite and peroxy-nitrite anions were generated when oxygen reacts with the excess NO. CS/PLA-PIC NPs compete with oxygen to react with NO and thus inhibit the generation of the nitrite and peroxy-nitrite anions. The activities of DPPH, nitric oxide and SOD were scavenged in a concentration dependent manner. From results, it confirmed that the CS/PLA-PIC NPs showed potent antioxidant activity than CS-PLA NPs and PIC. CS/PLA-PIC NPs showed good antioxidant activity than quercitin.
Cytotoxicity assay
The foremost challenge in this study is to demonstrate the cytotoxicity of CS/PLA-PIC NPs under in vitro conditions in HepG2, A549 and MCF7 cells using cytotoxicity markers, including mitochondrial function (MTT reduction) and membrane integrity (LDH leakage). These are the basic markers for the estimation of toxicity for a wide range of nanoparticles in different kinds of cell lines, at which 50% of the cells die in a given time frame (IC50) [Citation24]. To examine the toxic effects of synthesized CS-PLA NPs and CS/PLA-PIC NPs were incubated with different concentrations (1, 5, 10, 20 and 40 µg/ml) with HepG2, A549 and MCF7 cells for 24 h and the cell viability were determined. The CS/PLA-PIC NPs showed specific toxicity on cancer cells. IC 50 concentration of CS/PLA-PIC NPs against HepG2 (), MCF7 () and, A549 () cells are 5, 10 and 10 µg/ml. Results of MTT assays clearly revealed that CS/PLA-PIC NPs exerted slightly better cytotoxic effect than CS-PLA NPs in a dose-dependent manner towards HepG2, A549 and MCF7 cells.
Figure 3. (A) MTT assay of CS-PLA NPs, PIC and CS/PLA-PIC NPs with (a) HepG2. (b) A549 (c) MCF7 cell lines. (B) LDH activity of CS-PLA NPs, PIC and CS/PLA-PIC NPs with (a) HepG2. (b) A549 (c) MCF7 cell lines.
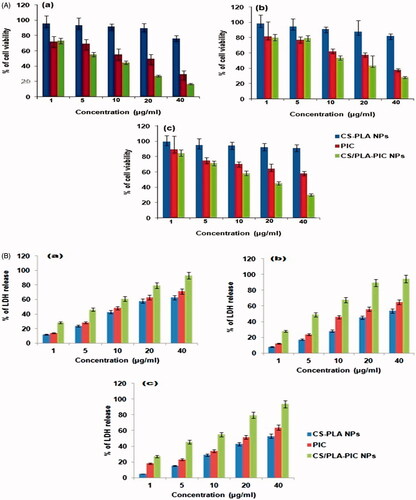
The extent of HepG2 (), A549 () and MCF7 () cell membrane rupture was revealed by LDH levels in cell medium. The LDH levels in the cell culture after exposure for a period of 24 h to CS/PLA NPs was 58.1% whereas it’s increased to 73.5% when exposed to CS-PLA-PIC NPs. Both these assays demonstrated that the CS/PLA-PIC NPs exert significant cytotoxicity to HepG2, A549 and MCF7 cells in dose-dependent manner in the concentration range of 1–40 µg/ml.
It is apparent that the rate of glycolysis increased by cancer cells wherein there is a conversion of pyruvate into lactate which was catalyzed by LDH, which consumes NADH and regenerates NAD. This, in turn, makes the cell more vulnerable to programmed cell death (apoptosis) and decreasing the cell viability indicating that cell death is the primary cause for the cell number reduction that is evident by the significant increase in the LDH activity and promoting cell membrane damage. The noticeable cytotoxicity is due to the smallest size that can interact readily with biomolecules either on the surface of or within cells. In addition, Lin et al established in his study that upon treatment with 15 and 46 nm of amorphous silica nanoparticles at 50 µg/ml for 48 h against A549 cell line, the cell viability was decreased significantly for both the sizes [Citation25]. Thus, with nanoparticles toxicity, it is often expected that smaller the size, stronger the exerted cytotoxicity [Citation26]. Results of cell viability assays clearly revealed that CS/PLA-PIC NPs exerted better cytotoxic effect than CS-PLA NPs and PIC in a dose- dependent manner towards cancer cells.
DNA fragmentation
DNA fragmentation is broadly considered as a characteristic feature of apoptosis [Citation27]. Shrunken of cells and DNA fragmentation are two factors which confirms that apoptosis has been induced. This scenario is evidenced by performing agarose gel electrophoresis of DNA extracted from cultured HepG2, A549 and MCF7 cells treated IC 30 and IC 50 of CS/PLA-PIC nanoparticles for 24 h. clearly indicates that the DNA “laddering” pattern in HepG2, A549 and MCF7 cells treated with CS/PLA-PIC nanoparticles is one of the reasons for cell death. Necrotic degeneration was prominent in cells and it was confirmed by degradative smearing of DNA when incubated for 24 h with IC30 concentration (lane 1) and the fragmented DNA increased greatly in cells treated with IC 50 concentrations (lane 2) for HepG2 and A549. There was no differentiation in the fragments of DNA at IC30 and IC50 concentrations in MCF7-treated cells.
Figure 4. Analysis of DNA isolated from HepG2, A549 and MCF cells incubated with IC30 and IC50 concentration of CS/PLA-PIC NPs for 24 h; cells without treatment (lane C), HepG2 cells treated with 20 µg/ml (lane 1), and 40 µg/ml (lane2), A549 cells treated with 40 µg/ml (lane 1), and 60 µg/ml (lane 2) and MCF7 cells treated with 40 µg/ml(lane 1), and 60 µg/ml(lane 2).
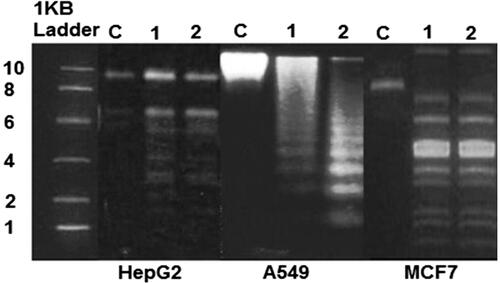
CS/PLA-PIC NPs treated cells was accompanied by a reduction in the percentage of cells in G0/G1 phase and an increase in the percentage of G2/M phase cells, indicating cell cycle arrest at G2/M [Citation28,Citation29]. The ROS can act as signal molecules promoting cell cycle progression and can induce oxidative DNA damage [Citation30].
Earlier studies by Gurunathan et al demonstrated that DNA ladder was formed in both cancer and non-cancer cell lines when they are treated with silver nanoparticle [Citation31]. Cell division could be affected due to the deposition of metal particles inside the nucleus. Dose-dependent DNA damage in DNA, aberrations in chromosomes, chromosome segregation errors and formation of sister chromatic exchanges were revealed in genotoxic studies of titanium dioxide (TiO2). Treatment with AgNPs induced the production of micronuclei (MN). Mroz et al hypothesized that nanoparticles and ROS induce DNA damage, activating p53 (apoptotic gene), and proteins related to DNA repair and mimicking irradiation related carcinogenesis [Citation32].
AO/EB staining
A549, HepG2 and MCF7 cells were labelled with AO/EB for 24 h after exposing to IC30, IC50 and IC90 concentrations of CS/PLA-PIC nanoparticles. Dual staining was examined under a fluorescent microscope. No significant apoptosis was detected in the control group (). Early-stage apoptotic cells, marked by crescent-shaped or granular yellow-green AO nuclear staining, were detected in the experimental group (). Staining was localized asymmetrically within the cells. By increasing the dose concentrations and treatment time, the early-stage of apoptotic cells were increased. Late-stage apoptotic cells, with concentrated and asymmetrically localized orange nuclear EB staining, were also detected (). Necrotic cells increased in volume and size in MCF cells when exposed to IC30 and IC50 which showed uneven orange-red fluorescence at their periphery. The cells appeared to be in the process of disintegration in IC90 concentration ().
Figure 5. Cells stained with AO and EB of CS/PLA-PIC treated HepG2, A549, and MCF cells at 24 h. Cells without treatment (control) HepG2 cells treated with (a) 20 µg/ml, (b) 40 µg/ml and (c) 60 µg/ml. A549 cells treated with (a) 40 µg/ml, (b) 60 µg/ml and (c) 80 µg/ml. MCF7 cells treated with (a) 40 µg/ml, (b) 60 µg/ml and (c) 80 µg/ml.
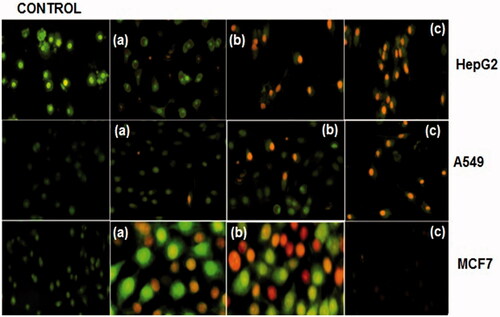
Dual AO/EB staining is a qualitative and quantitative method which detects the phenotypic changes in apoptotic cells and also differentiates between normal cells, early and late apoptotic cells and necrotic cells [Citation33]. This study also proves that AO was entered into intact membranes of normal and early apoptotic cells which provide fluorescence green when bound to DNA, whereas EB penetrates into the damaged membrane of late apoptotic and dead cells which provides orange-red fluorescence [Citation34]. Additionally, double staining is also capable to identify minor DNA injuries [Citation35]. Consequently, this study concludes that AO/EB staining differentiated normal, early apoptotic, late apoptotic cells and dead cells, a nuclear morphology of the cancer cells exposed to CS/PLA-PIC NPs with varying concentrations.
Flow cytometric analysis
Further to quantify cell apoptosis, the HepG2, A549 and MCF7 cells exposed to an IC50 concentration of CS/PLA-PIC nanoparticles were stained using Annexin-V-FITC/propidium iodide and analysed by flow cytometry. The results showed that when cells were exposed to IC 50 of CS/PLA-PIC NPs for 24 h, the proportion of Annexin-V+/PI + (necrotic cells) in HepG2, A549 and MCF cells were 7.8, 9.1 and 12.3% increased from control cells, and the number of AV+/PI- (apoptotic cells) were 57.2, 49.1 and 40.2% respectively. Results indicating that apoptosis induced in HepG2, A549, and MCF cells by CS/PLA-PIC NPs are dose-dependent and suggest that both necrosis and apoptosis contribute to the CS/PLA-PIC NPs-induced death of HepG2, A549 and MCF7 cells (). Cell cycle analysis is the method to assess homeostasis, apoptosis, or programmed cell death. Dysregulation of apoptosis is implicated in the development of most cancers. Thus, induction of apoptosis in cancer cells is an important focus in the discovery of anticancer drugs [Citation36]. Annexin-V, a calcium-dependent phospholipid-binding protein with a high affinity for phosphatidylserine, can, therefore, be used as a sensitive probe for the presence of phosphatidylserine on the cell membrane and hence as a marker of apoptosis. Propidium iodide is a non-specific DNA intercalating agent, which is excluded by the plasma membrane of living cells and thus can be used to distinguish necrotic cells from apoptotic and living cells by supravital staining without prior permeabilization. Annexin-V-FITC/PI staining exhibited distinct dose-dependent induction of apoptosis after 24 h in HepG2, A549 and MCF7 cells treated with CS/PLA-PIC NPs.
Figure 6. Flow Cytometric analysis of the cancer cell lines treated with CS/PLA-PIC NPs (right) compared with control (left).
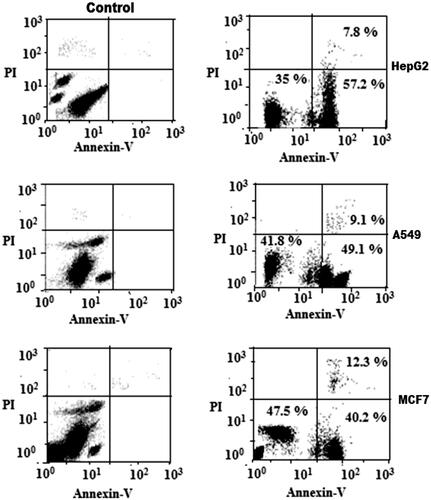
We conclude from this study that the synthesized CS/PLA-PIC NPs demonstrated strong proliferative reticence in human liver, lung and breast cancer cell line in a dose-dependent manner. It also proposed that CS/PLA-PIC NPs can possibly alter apoptotic protein expression and activate apoptosis in mitochondria-dependent pathways in cancer cells.
Disclosure statement
No potential conflict of interest was reported by the authors.
References
- Nishiyama N. Nanomedicine: nanocarriers shape up for long life. Nat Nanotechnol. 2007; 2:203–204.
- Briggs NC, Levine RS, Bobo LD, et al. Wine drinking and risk of non-Hodgkin's lymphoma among men in the United States: a population-based case-control study. Am J Epidemiol. 2002;156:454–462.
- Crew KD, Neugut AI. Epidemiology of upper gastrointestinal malignancies. Semin Oncol. 2004; 31:450–464.
- Schoonen WM, Salinas CA, Kiemeney LA, et al. Alcohol consumption and risk of prostate cancer in middle-aged men. Int J Cancer. 2005; 113:133–140.
- Harmatha J, Zidek Z, Kmoničkova E, et al. Immunobiological properties of selected natural and chemically modified phenylpropanoids. Interdisc Toxicol. 2011; 4:5–10.
- Piotrowska H, Kucinska M, Murias M. Biological activity of piceatannol: leaving the shadow of resveratrol. Mutat Res. 2012; 750:60–82.
- Jeong SO, Son Y, Lee JH, et al. Resveratrol analog piceatannol restores the palmitic acid-induced impairment of insulin signaling and production of endothelial nitric oxide via activation of anti-inflammatory and antioxidative heme oxygenase-1 in human endothelial cells. Mol Med Rep. 2015;12:937–944.
- Wieder T, Prokop A, Bagci B, et al. Piceatannol, a hydroxylated analog of the chemopreventive agent resveratrol, is a potent inducer of apoptosis in the lymphoma cell line BJAB and in primary, leukemic lymphoblasts. Leukemia. 2001; 15:1735–1742.
- Wolter F, Clausnitzer A, Akoglu B, et al. Piceatannol, A natural analog of resveratrol, inhibits progression through the S phase of the cell cycle in colorectal cancer cell lines. J Nutrit. 2002; 132:298–302.
- Larrosa M, Tomas-Barberan FA, Espin JC. The grape and wine polyphenol piceatannol is a potent inducer of apoptosis in human SK-Mel-28 melanoma cells. Eur J Nutr. 2004; 43:275–284.
- Kuo PL, Hus YL. The grape and wine constituent piceatannol inhibits proliferation of human bladder cancer cells via blocking cell cycle progression and inducing as/membrane bound Fas ligand-mediated apoptotic pathway. Mol Nutr Food Res. 2008;52:408–418.
- Lee YM, Lim d. Y, Cho HJ, et al. Piceatannol, a natural stilbene from grapes, induces G1 cell cycle arrest in androgeninsensitive DU145 human prostate cancer cells via the inhibition of CDK activity. Cancer Lett. 2009;285:166–173.
- Kukreja A, Tandon S, Mishra A, et al. Piceatannol: a potential futuristic natural stilbene as fetal haemoglobin inducer. J Clin Diagn Res. 2013; 7:3028–3031.
- Honma H, Podratz JL, Windebank AJ. Acute glucose deprivation leads to apoptosis in a cell model of acute diabetic neuropathy. J Peripher Nerv Syst. 2003;8:65–74.
- Sharifi AM, Eslami H, Larijani BJ, et al. Involvement of caspase-8, 9, and 3 in high glucose-induced apoptosis in PC12 cells. Neuroscience Lett. 2009; 459:47–51.
- Jeevitha D, Malathy BR, Santhosh B. Toxic effects of aflatoxin B1 on embryonic development of zebrafish (Danio rerio): potential activity of piceatannol encapsulated chitosan/poly (lactic acid) nanoparticles. Anticancer Agents Med Chem. 2015; 15:248–257.
- Khor E. Chitin: Fulfilling a Biomaterials Promise. Oxford, UK: Elsevier Science 2001.
- Naghieh S, Badrossamay M, Foroozmehr E, et al. Combination of PLA micro-fibers and PCL-gelatin nano-fibers for development of bone tissue engineering scaffolds. Int J Swarm Intel Evol Comput. 2017; 6:150.
- Rahul MR, Amol VJ, Douglas EH. Poly lactic acid modifications. Progress in Polymer Sci. 2010; 35:338–356.
- Jeevitha D, Amarnath K. Chitosan/PLA nanoparticles as a novel carrier for the delivery of anthraquinone: synthesis, characterization and in vitro cytotoxicity evaluation. Colloids Surf B Biointerfaces. 2013;101:126–134.
- Ramteke S, Uma Maheshwari RB, Jain NK. Clarithromycin based oral sustained release nanoparticulate drug delivery system. Indian J Pharm Sci. 2006;68:479–484.
- Lee BC, Lee SY, Lee HJ, et al. Anti-oxidative and Photo-protective effects of coumarins isolated from Fraxinus chinensis. Arch Pharm Res. 2007;30:1293–1301.
- Jain PK, Agrawal RK. Antioxidant and free radical scavenging properties of developed mono- and polyherbal formulations. Asian J Exp Sci. 2008;22:213–220.
- Mahmoudi M, Simchi A, Milani AS, et al. Cell toxicity of superparamagnetic iron oxide nanoparticles. J Colloid Interface Sci. 2009; 336:510–518.
- Lin W, Huang YW, Zhou XD, et al. In vitro toxicity of silica nanoparticles in human lung cancer cells. Toxicol Appl Pharmacol. 2006;217:252–259.
- Oberdorster G, Maynard A, Donaldson K, et al. Principles for characterizing the potential human health effects from exposure to nanomaterials: elements of a screening strategy. Particle Fiber Toxicol. 2005; 2:1–35.
- Allen RT, Hunter WJ, Agarwal DK. Morphological and biochemical characterization and analysis of apoptosis. J Pharmacol Toxicol Methods. 1997;37:215–228.
- Wyllie AH. Glucocorticoid-induced thymocyte apoptosis is associated with endogenous endonuclease activation. Nature. 1980; 284:555–556.
- Lee YS, Kim DM, Lee YH, et al. Silver nanoparticles induce apoptosis and G2/M arrest via PKCζ-dependent signaling in A549 lung cells. Arch Toxicol. 2011; 85:1529–1540.
- Alvarez-Gonzalez R, Spring H, Muller M, et al. Selective loss of poly(ADP-ribose), and the 85-kDa fragment of poly(ADP- ribose), polymerase in nucleoli during alkylation-induced apoptosis of HeLa cells. J Biol Chem. 1999;274:32122–32126.
- Gurunathan S, Han JW, Eppakayala V, et al. Cytotoxicity of biologically synthesized silver nanoparticles in MDA-MB-231 human breast cancer cells. BioMed Res Int. 2013;2013:535796. DOI:https://doi.org/10.1155/2013/535796
- Mroz RM, Schins RPF, Li H, et al. Nanoparticle-driven DNA damage mimics irradiation-related carcinogenesis pathways. Eur Res J. 2008;31:241–251.
- Biffl WL, Moore EE, Moore FA, et al. Interleukin-6 delays neutrophil apoptosis via a mechanism involving platelet-activating factor. J Trauma. 1996;40:575–578.
- Ribble D, Goldstein N, Norris DA, et al. A simple technique for quantifying apoptosis in 96-well plates. BMC Biotechnol. 2005;5:12–12.
- Gherghi IC, Girousi ST, Voulgaropoulos A, Tzimou-Tsitouridou NR. Study of interactions between DNA-ethidium bromide (EB), and DNA-acridine orange (AO), in solution, using hanging mercury drop electrode (HMDE). Talanta. 2003;61:103–112.
- Osborne BA. Apoptosis and the maintenance of homoeostasis in the immune system. Curr Opin Immunol. 1996;8:245–254.