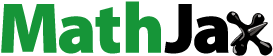
Abstract
The global burden of cancer have encouraged oncologists to develop novel strategies for treatment. Present study was proposed to develop Arginyl-glycyl-aspartic acid (RGD)-containing nanostructured lipid carriers (NLC) as a delivery system for improving the anticancer capability of epigallocatechin gallate (EGCG) on breast cancer cell line by attaching to integrin superfamily on cancer cells. For this purpose, RGD-containing EGCG-loaded NLC were prepared by hot homogenization technique and characterized by different techniques. Then, cytotoxic and apoptotic effects of prepared nanoparticles and their uptake into cells was evaluated. As results, the nanoparticles with particle size of 85 nm, zeta potential of −21 mV, encapsulation of 83% were prepared. Cytotoxicity and apoptosis experiments demonstrated that EGCG-loaded NLC-RGD possessed greatest apoptotic activity. Furthermore, it has been shown that, EGCG-loaded NLC-RGD causes cell cycle arresting more effective than EGCG. Therefore, loading EGCG into NLC-RGD make it more effective in both targeting and accumulation into tumour cells, which results from specialized uptake mechanism by adhesion to αvβ3 integrin. The results strengthen our hope that loading EGCG into RGD-containing NLC could possibly overcome the therapeutic limitations of EGCG and make it more effective in cancer therapy.
Graphical Abstract
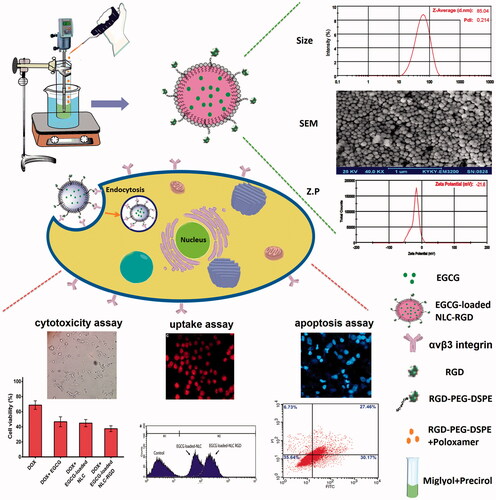
Introduction
The global burden of cancer and chemotherapy limitations such as drug resistance have encouraged oncologists to develop novel strategies for cancer prevention and treatment [Citation1,Citation2]. Using natural antitumor agents which could overcome chemotherapy limitations is one of such strategies [Citation3]. One of these natural agents is epigallocatechin gallate (EGCG) which comprises majority of the antioxidants in green tea [Citation4], and its anti-proliferative [Citation5], anti-angiogenic [Citation6] and apoptotic [Citation7,Citation8] properties have been demonstrated in a wide range of cell cultures and pre-clinical studies. Furthermore, EGCG interacts with P-glycoprotein (P-gp) and hinders the binding and efflux of drugs by P-gp [Citation9]. In spite of EGCGs benefits, poor cell penetration, efflux mechanisms and fast rate of glucuronide conjugation have limited its therapeutic potential [Citation10,Citation11]. Therefore, employing a nanoparticulate delivery system may enhance EGCGs bioavailability [Citation12]. In recent years, researchers have focused on nanoparticles as diagnosis and drug delivery systems [Citation13,Citation14]. Among various effective drug delivery systems, nanostructured lipid carriers (NLC) have concerned growing attention as a potential drug delivery carrier due to their high drug-loading capacity, physical stability, protection of encapsulated bioactive compound from degradation, easy preparation and low toxicity [Citation15]. NLCs are Lipid-based colloidal systems characterized by a solid lipid core containing a combination of solid and liquid lipids, and having a mean particle size in the nanometer range [Citation16]. In order to extend the capacity of a drug delivery system, nanoparticles that actively participate in the tumour targeting process is underway [Citation17]. As a consequence, enhanced permeability and retention (EPR) mediated passive and peptide mediated active tumour targeting have received noteworthy attention in the last years [Citation18]. Arginyl-glycyl-aspartic acid (RGD) is a motif present in several adhesion molecules, and is recognized by a certain set of integrins, especially αvβ3 integrin which overexpressed in certain cell types [Citation19]. Integrins are a group of cell adhesion molecules which directly interact with extracellular matrix (ECM) components when regulating cell migration and proliferation [Citation20]. Integrins also have very vital roles in cancer development and some subtypes of them, have been described to be extremely overexpressed on various cancer cells [Citation21]. It is also proposed that the RGD-containing nanoparticles can be penetrated into cells by integrin-mediated endocytosis [Citation22]. The aim of the present study was to prepare and optimize RGD-containing EGCG-loaded NLC (EGCG-loaded NLC-RGD) to increase EGCGs bioavailability and explore its ability in enhancing the cytotoxic and apoptotic effects of doxorubicin (DOX) on human breast cancer cell line, as a most repeatedly diagnosed cancer in human [Citation23].
Materials and methods
Material
EGCG, Poloxamer 407, 3–(4, 5-dimethylthiazol-2-yl)-2, 5-diphenyltetrazolium bromide (MTT), RPMI 1640 and foetal bovine serum (FBS) were purchased from Sigma Aldrich Company. Glyceryl palmito stearate (Precirol® ATO 5) and DSPE-PEG (2000) amine were supplied from Gattefosse (France) and Avanti Polar Lipids (Alabaster, AL), respectively. MDA-MB-231 breast cancer cell line was obtained from national cell bank of Iran (Pasteur institute, Iran). Annexin V-FITC apoptosis detection kit was prepared from Oncogene Research Products, (San Diego, CA).
Preparation of EGCG-loaded NLC-RGD
EGCG-loaded NLC-RGD was prepared by hot homogenization method. For this purpose, DSPE-PEG (2000) Amine was dissolved in DMSO and RGD was added and stirred for 24 h. After finishing point of the reaction, the solution was dialyzed sequentially against Milli-Q water (membrane tubing, molecular weight cut-off 1000 Da). After dialysis, product was lyophilized, and RGD-PEG-DSPE (yield 67.3%) was achieved.
The lipid phase, consisting of Precirol and Miglyol were heated up to 70 °C in a boiling water bath. EGCG was dissolved in ethanol and injected into the molten lipid phase. Subsequently, RGD-PEG-DSPE was dissolved in aqueous phase containing Poloxamer 407 in double distilled water at 70 °C and added gradually into the lipid phase under homogenization at 20,000 rpm for 40 min (Heidolph, Germany). The produced hot oil/water nano-emulsion was cold down into ambient or lower temperature resulting in the lipid phase re-crystallization, and finally the EGCG-loaded NLC-RGD was formed.
Physicochemical properties characterization
In order to gain the suitable system for nanoparticles preparation, the mean particle size, polydispersity index (PDI) and zeta potential (ZP) of the EGCG-loaded NLC-RGD were determined by dynamic light scattering technique (Nano ZS, Malvern Instruments, UK). The size of the particles reported by intensity and was expressed by the Z-Average value. Finally, in order to study the morphological behaviours, nanoparticles were observed with scanning electron microscopy (SEM). Briefly, samples were dropped on the glass object (1 cm2) and allowed air dried until water completely evaporated. Dried particle was put on SEM holder then coated with gold–palladium and evaluated by SEM (KYKY-EM3200, KYKY Technology Development Ltd., China).
Drug loading and physical stability studies
Drug encapsulation efficiency (EE) and loading capacity (LC) were determined using centrifugal filter tube (Amicon® Ultra-15 with molecular weight cut-off of 100 kDa, Millipore, USA). To determine the amount of non-encapsulated EGCG (free drug), 1 ml of formulation was taken and passed through Amicon® filters by centrifugation (3K30, Sigma, Germany) at 5000 rpm for 10 min to separate the free EGCG from the encapsulated ones. The solution in the bottom of Amicon® tube was used for EGCG determination by ultraviolet-visible spectrophotometer (Ultrascope 2000®, Pharmacia Biotech, UK) at ʎmax 276 nm. The EE and LC values of formulations were calculated using the following equations:
The physical stability of NLC formulations considering drug loading (the lack of drug leakage), size and PDI (the lack of aggregation or fusion of particles) were evaluated during 2 months of storage period at 4–8 °C.
MTT cell viability assay
In vitro cytotoxicity of nanoparticles with human breast cancer MDA-MB-231 cells were investigated by MTT assay. In brief, MDA-MB-231 cell line was cultured in RPMI-1640 supplemented with 10% FBS at 37 °C in humidified atmosphere with 5% CO2. Then, cells were seeded into a 96-well plate at 104 cells/well followed by 24-h incubation to allow cells attach to the surface of the wells. Subsequently, the medium was replaced with 200 µl fresh mediums containing various concentrations of EGCG, DOX, EGCG-loaded NLC and EGCG-loaded NLC-RGD. After 48 h, medium of all wells were removed and 200 μl of fresh medium containing MTT was added to each well and incubated for 4 h at 37 °C. Finally, the formazan crystals were dissolved in 200 µl of DMSO and the absorbance was measured at 570 nm using a microplate reader (Biotek, ELX 800).
Haemolysis assay
Haemolytic activity of nanoparticles (NPs) was assessed according to Wang et al. method [Citation24]. The fresh blood was collected and centrifuged at 1000 rpm for 10 min to remove the plasma. The resulted precipitation was washed several times with 5 ml of cold PBS by centrifugation. Next, 0.1 ml of the diluted RBC suspensions were added separately to 900 μL of each samples (EGCG-loaded NLC-RGD diluted in PBS) and followed by gentle shaking and incubating for 2 h at room temperature. PBS and water was used as the negative and positive control respectively. Finally, absorbance of the haemoglobin in supernatants was determined by UV-vis spectrophotometer at 541 nm. The percentage of haemolysis was calculated as
where Atest, Aneg, Apos are the absorbance values of the test sample, negative control (PBS) and positive control (water), respectively.
Cell uptake study
To confirm the uptake of NLC into the cells, rhodamine-B (0.01%w/w with regarding to the weight of the lipids) was used as a fluorescence probe to produce fluorescent NLCs for in vitro uptake studies. Unloaded rhodamin-B was removed by filtration using centrifugal filter devices. Then, the cultured cells in 6-well plates were treated with fluorescent NLC and NLC-RGD for 2 h. Thereafter, the cells were washed five times with PBS and analyzed by FACS caliber flow cytometer (Becton Dickinson Immunocytometry Systems, San Jose, CA) and fluorescence microscope (Olympus microscope Bh2-RFCA, Japan). All the experimental steps were performed in dark to avoid fluorescent dequenching.
Apoptosis assay
In order to detect necrosis, early and late apoptosis, FITC-labelled annexin V assay was carried out. In brief, MDA-MB-231 cells were treated with 1 µM of DOX and 10 µM of EGCG-loaded NPs for 48 h, and then, cells were detached, washed and were incubated with 200 μl of binding buffer containing 5 µl FITC-labelled Annexin V in the dark for 14 min at 37 °C. Cells were washed again with 500 µl binding buffer and were treated by 200 µl binding buffer with 5 µl propidium iodide (PI). Finally, the samples were analysed by Becton Dickinson FACS Calibur System (San Jose, CA).
Cell-cycle arrest analysis
Cell-cycle arrest was assessed by PI staining of cells. Cells incubated for 24 h with DOX + EGCG loaded NPs. Then, cells were collected and fixed in 1.5 ml aqueous ethanol (70%) at 4 °C overnight. Cells were then stained with PI in the presence of ribonuclease A for 30 min in dark. Finally, the cell population was analysed by Becton Dickinson FACS Calibur System (San Jose, CA).
DAPI (4′, 6-diamidino-2-phenylindole dihydrochloride) staining
MDA-MB-231 cells in six-well plates were treated with EGCG, DOX and EGCG-loaded NPs for 48 h and then fixed with 4% paraformaldehyde and permeabilized in 0.1% (w/v) Triton X-100, washed with PBS and treated by DAPI for 30 min in the dark. Nuclear morphology was evaluated under a fluorescence microscope to identify cells suffering apoptosis.
Statistical analyses
Experiments for each sample were performed three times, and all results were presented as the means ± standard deviation (SD) that was calculated by Microsoft® Excel 2013. Mean values were compared using Student’s t-test to compare mean differences between two groups and ANOVA was used to multiple comparisons. A value of p < .05 was considered to be statistically significant.
Results
Preparation and characterization of EGCG-loadedNLC-RGD
RGD containing EGCG-loaded NLCs were successfully prepared through the hot homogenizing method using diverse percent of Percirol, Miglyol and Poloxamer. Regarding to nanoscale particle size, and narrow polydispersity and higher LC, the best formulation was composed of 10 mg Miglyol, 100 mg Precirol as a core material for the NLCs and 50 mg Poloxamer as aqueous phase surfactants and stabilizer of particulate system. The DLS graph () of optimized formulation displayed that the mean size of NPs are 85 nm with a polydispersity index (PDI) of 0.21 that proposes a relatively narrow size distribution. Generally, PDI <0.5 confirms the monodispersed particles [Citation25]. Scanning electron microscope () imaging confirmed these results. Average zeta potential value of nanoparticles was −21.6 mV ().
Drug loading and physical stability
The average value of EE and LC for optimum formulation were 83.03 ± 2.51% and 75.48 ± 2.28 mg/g, respectively. EGCG-loaded NLC-RGD displayed a good stability for 2 months when stored at 4–8 °C (). During this time, there was no noteworthy change in clarity and phase separation. The size, PDI and EE of samples were 92 nm, 0.27 and 78.12 ± 3.21, respectively, which showed that characteristic of nanoparticles had no significant alteration compared with fresh formulae (p < .05).
Cell viability assay
The cytotoxicity of EGCG, EGCG-loaded NLC, EGCG-loaded NLC-RGD and the synergistic effects of combinatorial treatment of DOX and EGCG-loaded NPs were evaluated by MTT assay. Data analysis of the cytotoxicity assay revealed that IC50s of DOX, EGCG, EGCG-loaded NLC, EGCG-loaded NLC-RGD on MDA-MB-231 cells are 3.0, 49.3, 45.2 and 38.2 μM, respectively (). As revealed in , combination treatment of the cells with [10 μM EGCG+ 1 μM DOX], [10 μM EGCG-loaded NLC+ 1 μM DOX] and [10 μM EGCG-loaded NLC-RGD+ 1 μM DOX] results in 46.61%, 44.85%, 37.48% cell viability, which shows that [EGCG-loaded NLC-RGD + DOX] has 9.13% and 7.37% more cytotoxicity in comparison to [EGCG + DOX] and [EGCG-loaded NLC + DOX] respectively. No apparent cytotoxicity of blank NLC and NLC-RGD was considered which indicating the biocompatibility of the nanocarriers.
Figure 2. Cytotoxic effects of EGCG, EGCG-loaded NLC, EGCG-loaded NLC-RGD (a), DOX (b) and synergistic effects of combinatorial treatment of 1 µM DOX and 10 µM of EGCG and EGCG-loaded NPs for 48 h on MDA-MB-231 cells (c). The figure illustrates that EGCG-loaded NLC-RGD are more anti-proliferative when compared with EGCG-loaded NLC (p > .05). Data are presented as mean ± standard deviation (n = 3).
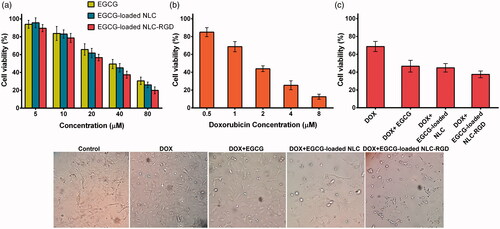
Haemocompatibility assay
Haemolytic test was performed to evaluate haemocompatibilty of synthesized NPs. As shown in , there is no considerable (less than 5%) haemolytic activity for EGCG-loaded NLC-RGD up to 45 µg/ml. It was reported that up to 5% haemolysis is accepted for nanoparticles [Citation24]. Optical microscopic images of RBCs confirmed these results (). The morphology of RBCs incubated with NPs at concentration of 500–600 µg/ml was the same as negative control but after enhancing the concentration to 600 µg/ml some of the cells exhibit some degree of deformation and membrane disruption.
Figure 3. Haemolysis assay of RBSs exposed to different concentration of NLC-RGD. (a) There is no considerable (less than 5%) haemolytic activity for EGCG-loaded NLC-RGD up to 45 µg/ml. Error bars represent the standard deviation of three measurements (n = 3). (b) Photographs of each treated sample as well as positive and negative controls. (c) Microscopic images of red blood cells treated by NLC-RGD.
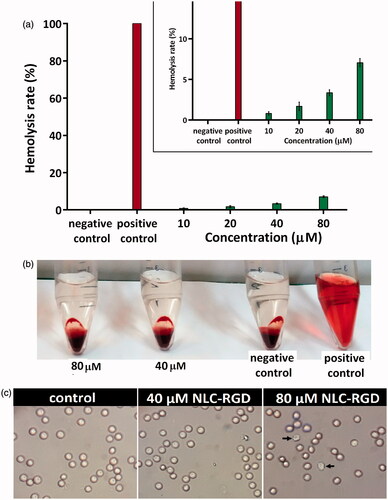
Uptake of nanoparticles into cells
In order to examine the impact of RGD in internalization of NLC inside MDA-MB-231 cells, the in vitro cellular uptake of rhodamin-loaded nanoparticles were analysed by FACS and fluorescence microscopy. As shown in , higher cellular uptake into MDA-MB-231 cells was detected for EGCG-loaded NLC-RGD with respect to EGCG-loaded NLC. The red fluorescence strength in the cells, which treated with EGCG-loaded NLC-RGD (), was higher and displayed more red dye in cytoplasm than cells which treated by EGCG-loaded NLC ().
Synergistic efficacy of DOX and EGCG-loaded NPs in apoptosis induction
To compare the percentage of apoptosis and necrosis induction by DOX + EGCG-loaded NLC with DOX + EGCG-loaded NLC-RGD, flow cytometry analysis Annexin V/PI double staining was performed. The fraction of viable cells (bottom left), early apoptotic cells (bottom right), late apoptotic cells (top left) and necrotic cells (top right) are presented in a quadrants in . Treating cells with DOX + EGCG-loaded NLC and DOX + EGCG-loaded NLC-RGD, induced apoptosis in about 49% and 58% of cells respectively. These results showed the delivery capability of NLC-RGD into tumour is more precise than NLC.
Figure 5. (a) The percentage of apoptosis and necrosis in MDA-MB-231 cells induced by combinatorial treatment of DOX and EGCG-loaded NPs. (b) Effects of combinatorial treatment of DOX and EGCG-loaded NPs on cell-cycle distribution of MDA-MB-231 cells. (c) Fluorescent images of DAPI stained cancerous cells following a 48 h exposure by DOX and EGCG-loaded NPs.
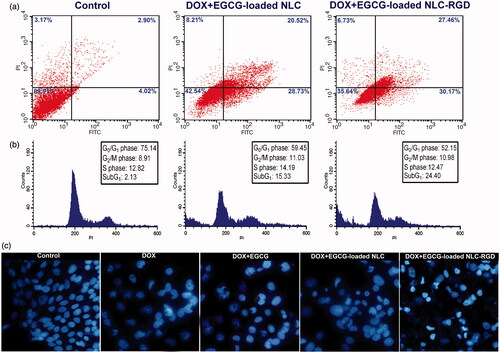
Effect of EGCG loaded NLC-RGD on cell cycle arrest
Effect of EGCG-loaded NLC-RGD on the cell cycle was examined by PI staining. As shown in , exposure of cells to DOX + EGCG-loaded NLC-RGD resulted in increase in the proportion of apoptotic cells as reflected by the sub-G1 peak (apoptotic cells). The values of sub-G1 of DOX + EGCG-loaded NLC treatment group increased from 15.33% to 24.44% when loaded into NLC-RGD. As displaying in , percentage of cells was decreased at G0/G1 and S phase after treatment with DOX + EGCG-loaded NLC-RGD. These results established that EGCG-loaded NLC-RGD causes cell-cycle arresting more effective than EGCG-loaded NLC on cancerous cells.
DAPI staining
To examine synergistic effect of DOX and EGCG-loaded NPs on nucleus morphology, a simple factor for the determination of healthy and apoptotic cells, DAPI staining was carried out. The obtained results confirmed the results of flow cytometry. Fluorescent images of DAPI stained cells showed that DOX + EGCG-loaded NLC-RGD induce nucleus condensation or chromatin degradation more noticeably than DOX + EGCG-loaded NLC and DOX + EGCG at the equal concentration and time treatment (). Untreated cells as a negative control showed no sign of fragmentation and segmentation after DAPI staining ().
Discussion
This study identified a RGD-containing NLC formulation for improved tumour accumulation of EGCG and compared apoptotic effects of EGCG-loaded NLC-RGD with free EGCG and EGCG-loaded NLC. RGD is a common integrin-binding tripeptide which has been widely used as a targeting molecule for anticancer therapies [Citation17]. The characterization of prepared nanoparticles (size, ZP, EE, LC) approves the appropriateness of the preparation process. The size of nanoparticles used in a drug delivery system should be large enough (>30 nm) to prevent their elimination by renal excretion, but small enough (up to 200 nm) to escape rapidly taken up by the mononuclear phagocyte system (MPS) cells [Citation26]. Zeta potential is an important factor for predicting the stability of nanoparticles. High zeta potential value implies high electric surface charge on nanoparticles which can lead to strong repulsive forces among nanoparticles to prevent it from aggregation [Citation27]. In contrast, low value of the zeta potential is indicated to display better permeability across cell membranes [Citation28]. The zeta potential of selected formulation in this study was -21.6 mV, therefore the system showed reasonable stability and drug release pattern, either even it is not too high to afraid from cell membrane.
In this study, MDA-MB-231 cell line was used to evaluate the efficacy of EGCG-loaded NLC-RGD in the treatment of breast cancer in vitro. The MDA-MB-231 is a highly expressed αvβ3 integrin human breast cancer cell line which do not express any of human epidermal growth factor receptor 2 (HER2), oestrogen receptor (ER) and progesterone receptor (PgR) [Citation29,Citation30]. The αvβ3 integrin receptor is specifically overexpressed in some tumour cells and has been implicated in the promotion of tumour growth, neovascularization, angiogenesis and the initiation, progression and metastasis of solid tumours [Citation31,Citation32]. It allows tumour cell adhere to blood vessels by interacting with αIIbβ3 expressed on platelets via fibrinogen leading to metastasis of breast cancer to bone marrow [Citation31]. Therefore, targeting drug via NLC-RGD not only enhance delivery of drug to the cell, also can potentially hinder cell adhesion and invasion by inhibition αvβ3 integrin receptor. The results () showed that the uptake of RGD-containing NPs by cells is marginally greater than that of the NPs, which suggested RGD have a high binding affinity for cells and enhance endocytosis. Data of MTT assay confirmed that EGCG-loaded NLC-RGD has more cytotoxicity than EGCG-loaded NLC because of more accumulation of EGCG in cells. For a cancer treatment to be curative, the delivery system must competently internalize the tumour tissue to reach all of the viable cells [Citation33,Citation34] and our findings indicated that RGD could intensify the uptake and penetration of NPs into integrin positives tumour cells. The results also showed that EGCG-loaded NLC-RGD beside Dox possessed the greatest synergistic apoptotic activity, which may benefit from its increased uptake by tumours and cause escape from the influence of P-glycoprotein pumps. Another possible mechanism through which EGCG increase the apoptotic effect of DOX, is downregulation of P-glycoprotein, a pump which efflux the drugs [Citation35]. In in-vivo systems and clinical trial, in addition to the high uptake (active targeting), NLC-RGD may benefit passive targeting which indicate that the transport of nanoparticles is mediated by Peyer’s patches (M cells) in the small intestine, and they enter the systemic circulation through lymphatic transport which enhances oral drug absorption [Citation36]. Moreover, bypassing the liver through lymphatic transport leads to a reduced first-pass metabolism and consequently enhances oral drug bioavailability [Citation37]. In accordance to this study, Coa et al. [Citation22] also reported that RGD modified liposomes was more efficaciously penetrated into tumour spheroids than liposomes in prostate cancer cells. Observations of nucleus morphology and result of apoptotic assay illustrated the high apoptotic activities of EGCG-loaded NLC-RGD in comparison with EGCG-loaded NLC, which is probably related to high endocytosis of RGD containing NPs. Results of cell cycle test confirmed that the effects of EGCG-loaded NLC-RGD on induction of apoptosis is associated with modifications in the cell cycle progression by triggering Sub-G1 arrest. Similar to this result, arresting cell cycle in sub-G1 by EGCG was reported on HCT116 cell line by Thakur et al. [Citation38]. EGCG in combination with chemotherapeutic agent such as DOX [Citation39], Paclitaxel [Citation40] and trastuzumab [Citation41] exhibits a synergistic activity in blocking the growth of cancerous cells and reducing the IC50 values of these chemotherapeutic agents. Improved targeting of EGCG will intensify the DOX effects that can lead to use of low dose of DOX in cancer treatment which in turn will cause decreasing the undesirable side effects of DOX. In accordance to this investigation, folate-mediated EGCG bovine serum albumin nanoparticles [Citation42] and chitosan-based nanoparticles [Citation43] have been prepared to improve EGCGs efficacy on cancer cells. Although chitosan is a safe biopolymer for the delivery of therapeutic agents [Citation13], In comparison to chitosan delivery system, NLC is less toxic due to the biocompatible lipids composition. Furthermore, NLC systems have less cost, aqueous solubility and easy preparation method. Additionally prepared NPs in this study are haemocompatibile and experiments showed no haemoglobin released into the plasma when nanoparticles come into contact with erythrocytes. Our results verified that NLC-RGD could be a hopeful drug delivery system in cancer treatments, but Shuhendler et al. [Citation44] showed that cyclic RGD increased clearance of nanoparticles by the mononuclear phagocytic system. However, further study is still needed to investigate the removing rate of NLC-RGD.
Conclusions
EGCG improves the apoptotic and anti-proliferative activity of DOX. Present study demonstrated that loading EGCG into NLC-RGD make it more effective in targeting and accumulation into tumor cells due to their specialized uptake mechanism by adhesion to αvβ3 integrin. We suggest that applying EGCG-loaded NLC-RGD along with chemotherapeutic drugs can be considered as a significant adjuvant to increase the efficacy of chemotherapeutics in cancer treatment.
Acknowledgements
We would like to thank to Drug Applied Research Center of Tabriz University of medical sciences for their kind assistance.
Disclosure statement
The authors report no conflicts of interest.
Additional information
Funding
References
- Iwamoto T. Clinical application of drug delivery systems in cancer chemotherapy: review of the efficacy and side effects of approved drugs. Biol Pharm Bull. 2013;36:715–718.
- Coughlin SS, Ekwueme DU. Breast cancer as a global health concern. Cancer Epidemiol. 2009;33:315–318.
- Vella N, Aiello M, Russo AE, et al. ‘Genetic profiling’and ovarian cancer therapy (review). Molecular Med Rep. 2011;4:771–777.
- Barghi S, Amiri M, Hajipour H, et al. The effect of dietary constituents on regulation of epigenetic changes in cancer. J Babol Univ Med Sci. 2017;19:63–71.
- Wang P, Heber D, Henning SM. Quercetin increased the antiproliferative activity of green tea polyphenol (-)-epigallocatechin gallate in prostate cancer cells. Nutr Cancer. 2012;64:580–587.
- Wang CC, Xu H, Man GCW, et al. Prodrug of green tea epigallocatechin-3-gallate (Pro-EGCG) as a potent anti-angiogenesis agent for endometriosis in mice. Angiogenesis. 2013;16:59–69.
- Shuntaro T, Keisuke H, Motofumi K, et al. Green tea polyphenol EGCG induces lipid-raft clustering and apoptotic cell death by activating protein kinase Cdelta and acid sphingomyelinase through a 67 kDa laminin receptor in multiple myeloma cells. Biochem J. 2012;443:525–534.
- Hajipour H, Hamishehkar H, Raeisi S, et al. Epigallocatechin-3-gallate induces apoptosis through up-regulation of bax and down-regulation of Bcl-2 in prostate cancer cell line. Int J Med Lab. 2016;3:262–269.
- Jodoin J, Demeule M, Béliveau R. Inhibition of the multidrug resistance P-glycoprotein activity by green tea polyphenols. Biochimica Et Biophysica Acta (BBA)-Molecular Cell Res. 2002;1542:149–159.
- Cai Y, Anavy ND, Chow HS. Contribution of presystemic hepatic extraction to the low oral bioavailability of green tea catechins in rats. Drug Metab Dispos. 2002;30:1246–1249.
- Manach C, Williamson G, Morand C, et al. Bioavailability and bioefficacy of polyphenols in humans. I. Review of 97 bioavailability studies. Am J Clin Nutr. 2005;81:230S–242S.
- Rocha S, Generalov R, Pereira MC, et al. Epigallocatechin gallate-loaded polysaccharide nanoparticles for prostate cancer chemoprevention. Nanomedicine. 2011;6:79–87.
- Fasihi-Ramandi M, Ghobadi-Ghadikolaee H, Ahmadi-Renani S, et al. Vibrio cholerae lipopolysaccharide loaded chitosan nanoparticle could save life by induction of specific immunoglobulin isotype. Artif Cells Nanomed Biotechnol 2017 [Feb 28]; [6 p.]. DOI:https://doi.org/10.1080/21691401.2017.1290646
- Bagdeli S, Rezayan AH, Taheri RA, et al. FRET-based immunoassay using CdTe and AuNPs for the detection of OmpW antigen of Vibrio cholerae. J Lumin. 2017;192:932–939.
- Tamjidi F, Shahedi M, Varshosaz J, et al. Nanostructured lipid carriers (NLC): a potential delivery system for bioactive food molecules. Innova Food Sci Emerg Technol. 2013;19:29–43.
- Doktorovova S, Souto EB. Nanostructured lipid carrier-based hydrogel formulations for drug delivery: a comprehensive review. Expert Opin Drug Deliv 2009; 6:165–176.
- Pearce TR, Shroff K, Kokkoli E. Peptide targeted lipid nanoparticles for anticancer drug delivery. Adv Mater Weinheim. 2012;24:3803–3822.
- Kunjachan S, Pola R, Gremse F, et al. Passive versus active tumor targeting using RGD-and NGR-modified polymeric nanomedicines. Nano Lett. 2014;14:972–981.
- Van de Walle GR, Peters ST, VanderVen BC, et al. Equine herpesvirus 1 entry via endocytosis is facilitated by αV integrins and an RSD motif in glycoprotein D. J Virol. 2008;82:11859–11868.
- Desgrosellier JS, Cheresh DA. Integrins in cancer: biological implications and therapeutic opportunities. Nat Rev Cancer. 2010;10:9–22.
- Marelli UK, Rechenmacher F, Sobahi TRA, et al. Tumor targeting via integrin ligands. Front Oncol. 2013;3:222.
- Cao Y, Zhou Y, Zhuang Q, et al. Anti-tumor effect of RGD modified PTX loaded liposome on prostatic cancer. Int J Clin Exp Med. 2015;8:12182.
- Torre LA, Bray F, Siegel RL, et al. Global cancer statistics, 2012. Ca Cancer J Clin. 2015;65:87–108.
- Wang X, Wang J, Bao Y, et al. Novel reduction-sensitive pullulan-based micelles with good hemocompatibility for efficient intracellular doxorubicin delivery. RSC Adv. 2014;4:60064–60074.
- Ngamcherdtrakul W, Morry J, Gu S, et al. Cationic polymer modified mesoporous silica nanoparticles for targeted siRNA delivery to HER2+ breast cancer. Adv Funct Mater. 2015;25:2646–2659.
- Martins S, Costa-Lima S, Carneiro T, et al. Solid lipid nanoparticles as intracellular drug transporters: an investigation of the uptake mechanism and pathway. Int J Pharm. 2012;430:216–227.
- Arulmozhi V, Pandian K, Mirunalini S. Ellagic acid encapsulated chitosan nanoparticles for drug delivery system in human oral cancer cell line (KB). Colloids Surf B Biointerf. 2013;110:313–320.
- Eskandani M, Nazemiyeh H. Self-reporter shikonin-act-loaded solid lipid nanoparticle: formulation, physicochemical characterization and geno/cytotoxicity evaluation. Eur J Pharm Sci. 2014;59:49–57.
- Bauer K, Mierke C, Behrens J. Expression profiling reveals genes associated with transendothelial migration of tumor cells: a functional role for alphavbeta3 integrin. Int J Cancer. 2007;121:1910–1918.
- Pal SK, Childs BH, Pegram M. Triple negative breast cancer: unmet medical needs. Breast Cancer Res Treat. 2011;125:627–636.
- Felding-Habermann B, O'Toole TE, Smith JW, et al. Integrin activation controls metastasis in human breast cancer. Proc Natl Acad Sci. 2001;98:1853–1858.
- Huveneers S, van den Bout I, Sonneveld P, et al. Integrin alpha v beta 3 controls activity and oncogenic potential of primed c-Src. Cancer Res. 2007;67:2693–2700.
- Du J, Lu W-L, Ying X, et al. Dual-targeting topotecan liposomes modified with tamoxifen and wheat germ agglutinin significantly improve drug transport across the blood − brain barrier and survival of brain tumor-bearing animals. Mol Pharm. 2009;6:905–917.
- Jain RK. Delivery of molecular and cellular medicine to solid tumors. Adv Drug Deliv Rev. 2001;46:149–168.
- Farabegoli F, Papi A, Bartolini G, et al. (-)-Epigallocatechin-3-gallate downregulates Pg-P and BCRP in a tamoxifen resistant MCF-7 cell line. Phytomedicine. 2010;17:356–362.
- Pandey R, Ahmad Z, Sharma S, et al. Nano-encapsulation of azole antifungals: potential applications to improve oral drug delivery. Int J Pharm. 2005;301:268–276.
- Kakran M, Sahoo N, Li L. Dissolution enhancement of quercetin through nanofabrication, complexation, and solid dispersion. Colloids Surf B Biointerfaces. 2011;88:121–130.
- Thakur VS, Amin AR, Paul RK, et al. p53-Dependent p21-mediated growth arrest pre-empts and protects HCT116 cells from PUMA-mediated apoptosis induced by EGCG. Cancer Lett. 2010;296:225–232.
- Stearns ME, Amatangelo MD, Varma D, et al. Combination therapy with epigallocatechin-3-gallate and doxorubicin in human prostate tumor modeling studies: inhibition of metastatic tumor growth in severe combined immunodeficiency mice. Am J Pathol. 2010;177:3169–3179.
- Luo T, Wang J, Yin Y, et al. (-)-Epigallocatechin gallate sensitizes breast cancer cells to paclitaxel in a murine model of breast carcinoma. Breast Cancer Res. 2010;12:R8.
- Menendez JA, Vazquez-Martin A, Colomer R, et al. Olive oil's bitter principle reverses acquired autoresistance to trastuzumab (Herceptin™) in HER2-overexpressing breast cancer cells. BMC Cancer. 2007;7:80.
- Zu Y, Yuan S, Zhao X, et al. Preparation, activity and targeting ability evaluation in vitro on folate mediated epigallocatechin-3-gallate albumin nanoparticles. Yao Xue Xue Bao = Acta Pharmaceutica Sinica 2009;44:525–531.
- Siddiqui IA, Bharali DJ, Nihal M, et al. Excellent anti-proliferative and pro-apoptotic effects of (−)-epigallocatechin-3-gallate encapsulated in chitosan nanoparticles on human melanoma cell growth both in vitro and in vivo. Nanomed Nanotechnol Biol Med. 2014;10:1619–1626.
- Shuhendler AJ, Prasad P, Leung M, et al. A novel solid lipid nanoparticle formulation for active targeting to tumor αvβ3 integrin receptors reveals cyclic RGD as a double‐edged sword. Adv Healthcare Mater. 2012;1:600–608.