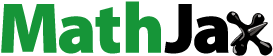
Abstract
Paeonol, as the main phenolic compound isolated from the Chinese herbs, has been confirmed to present anti-inflammatory effects on ulcerative colitis (UC) in our previous study. However, its poor solubility has hindered its development of being a favourable pharmaceutical product in treating colon diseases. In this study, we prepared the colon-specific delivery system (Pae-SME-CSC) with paeonol-loaded self-microemulsion (Pae-SMEDDS), and evaluated its in vitro and in vivo properties, especially the anti-inflammatory effects on UC rats. The anti-inflammatory effects were evaluated by the disease activity index, colon weight/length ratio, and macroscopic damage and microscopic damage scores. IL-17, IL-6, and TGF-β1 levels were also determined by enzyme-linked immunosorbent assay. The results showed that Pae-SME-CSC had good colon-targeting property in vivo and in vitro, with favourable stability. Efficacy evaluation showed that the dose of the paeonol group (100 mg/kg) exhibited no significant effect on UC (p > .05, compared with the model group), while the Pae-SME-CSC group (100 mg/kg) showed better anti-UC effects (p < .01 or p < .05), and its anti-inflammatory effect was close to that of the paeonol group (200 mg/kg) (p > .05). These results indicated that the developed Pae-SME-CSC was suitable for colon-specific drug delivery.
Introduction
Ulcerative colitis (UC), also known as chronic non-specific UC, is a long-term condition that results in inflammation and ulcers of the mucosal and submucosal layers of the colon and rectum, and its cause is still unknown [Citation1,Citation2]. The typical symptom of the disease is abdominal pain and diarrhoea mixed with mucus and blood [Citation3]. Long-term inflammation of the bowel may lead to various complications, including colorectal cancer, which harbours the most severe outcomes [Citation4]. Therefore, UC is considered as one of the precancerous lesions. Moreover, it is listed as one of the global refractory diseases by the World Health Organization because of the unclear aetiology and the absence of effective medicine. UC is characterized as difficult to cure and easy to relapse with long course of disease and cancerous tendency [Citation5]. Thus, adapting early intervention and treatment to shorten the course and reduce the lesion ranges is essential.
Despite the unclarified aetiology and pathogenesis of UC, many possible factors, such as immune regulatory dysfunction, hereditary susceptibility, infections, environmental factors, and intestinal barrier injury, play a role in the pathogenesis of UC. Besides surgical excision, UC can be treated by a number of medications, including salicylic acid, glucocorticoid, immunosuppressive agents, and immune modulatory agents [Citation6]. However, they are accompanied with toxic side effects of the kidney and liver, leukopenia, and impaired anti-infective ability in the long run [Citation7].
In traditional Chinese medicine, paeonol, 2′-hydroxy-4′-methoxyacetophenone, is the main active component isolated from Cynanchum paniculatum (Bge.) Kitag. and Aaeoina suffruticosa Andr. [Citation8,Citation9]. Paeonol has shown significant anti-inflammation [Citation10–12], anti-tumour [Citation13,Citation14], and anti-oxidant properties [Citation15]. In recent years, it has shown its biological effects for Parkinson’s disease [Citation16,Citation17], gastric cancer [Citation18], ovarian cancer [Citation19], prostate cancer [Citation20], colorectal cancer [Citation14], rheumatoid arthritis [Citation21,Citation22], osteoarthritis [Citation23], cardiovascular diseases [Citation24,Citation25], gastric ulcer [Citation26], pulmonary inflammation and fibrosis [Citation27], skin inflammation [Citation28], renal injury [Citation29,Citation30], neuroinflammation [Citation31,Citation32], and periodontitis [Citation33].
Paeonol has been demonstrated to reduce the activity of myeloperoxidase in the colon and inhibit the proliferation of iNOS, the expression of iNOS-mRNA induced by TNF-α + IFN-γ, and the activation of NF-κB and STAT1 signalling pathway in CW-2 and Jurkat cell lines [Citation34], which was possibly contributed to its anti-inflammation and anti-UC properties. In addition, in the dextran sulphate sodium-induced UC model of mice treated with paeonol, the anti-inflammatory and anti-oxidative activities of paeonol and its metabolite were related to the blocking of the MAPK/ERK/p38 signalling pathway [Citation35]. Notably, paeonol inhibited proliferation and induced apoptosis in LoVo cell lines in a dose- and time-dependent manner at a concentration of 15.63–250 mg/L, probably due to the enhanced concentration of intracellular calcium and upregulated expression of RUNX3 [Citation36]. These reported outcomes strongly indicated that paeonol displays significant anti-UC effects, which was also confirmed in our previous study. Previously, we have experimentally explored and confirmed the anti-inflammatory effects of paeonol in rats with 2,4,6-trinitrobenzenesulphonic acid (TNBS)-induced UC, and also found that the therapeutic efficacy presented dose-dependent property [Citation26]. In addition, paeonol was found to have low toxicity to mammals (LD50 orally: 3430 mg/kg rat) [Citation37], indicating that it was a relatively safe and potential compound for the oral colon-specific delivery system.
Oral colon-targeted drug delivery system is a research hotspot in treating colon diseases due to its enhanced therapeutic effect, reduced systemic side effects, and optimized patient compliance [Citation38]. Paeonol, as an active ingredient in this study, exhibits the definite anti-UC effect. However, no commercial medicinal product with paeonol for treating colon diseases is available.
The formulation study of paeonol found that it has relatively poor solubility (284.06–598.23 µg/mL) in different phosphate buffer solutions [Citation26]. This property might be unfavourable for the local treatment of colonic disease, wherein insoluble drugs are of incompletely released in the colon because the colon contains less fluid than the small intestines and stomach [Citation39]. Thus, as usually mentioned in pharmaceutical studies, poor solubility is the main problem that hinders most insoluble compounds from being developed into a favourable delivery system. Therefore, in the colon-specific preparation development in this study, the primary task is to employ a proper approach to improve paeonol hydrophobicity. To increase the absorption of paeonol in vivo, various formulations, such as nanoparticles [Citation40], gastro-retention tablets [Citation26], transethosomes [Citation41], nanocapsules [Citation42,Citation43], microparticles [Citation44], and microsponge formulations [Citation45], have been prepared.
Therefore, an oral drug delivery system targeted in the colon, with paeonol as the model drug, can be constructed by adopting proper pharmaceutical technologies. In this study, we used self-microemulsion technology to create the paeonol self-microemulsion drug delivery system (Pae-SMEDDS) with proper quality evaluation, which was anticipated to improve the solubility and stability of paeonol. Subsequently, Pae-SMEDDS was filled into colon-specific capsules to prepare the colon-specific delivery system (Pae-SMEDDS-loaded colon-specific capsule, Pae-SME-CSC), and differences in drug release, stability, pharmacokinetic behaviour, colon-targeting properties, and anti-inflammatory effects on UC were evaluated.
Materials and methods
Materials
The colon-targeted and gastric capsules for rats were purchased from Qiangji Pharmaceutical Co., Ltd. (Guangdong, China). Paeonol reference substance (purity: 99.9%, lot: 110708–201407) and magnolol were purchased from the National Institutes for Food and Drug Control (Beijing, China). Paeonol (purity: 99%, lot: ZL20110901) was purchased from Nanjing Zelang Medical Technology Co., Ltd. (Nanjing, China). Pooled human and rat liver microsomes were purchased from BD Gentest (Woburn, MA). Sulphasalazine (SASP) was purchased from Shanghai Sine Tianping Pharmaceutical Co., Ltd. (Shanghai, China). Pentobarbital sodium and TNBS were purchased from Sigma-Aldrich (Sigma-Aldrich Trading Co., Ltd., Shanghai, China). Cytokine enzyme-linked immunosorbent assay (ELISA) kits were purchased from Invitrogen (Grand Island, NY). Accessories of oleic acid, Labrafac Lipophile WL 1349, Labrasol, Cremophor RH40, and Transcutol HP were obtained from Gattefossé (Lyon, France). Other reagents were of analytical grade or better.
Male SD rats (200 ± 20 g) were purchased from Shanghai SIPPR-BK Laboratory Animal Co., Ltd. (Shanghai, China). All rats were housed at the specific pathogen-free-certified animal facility in the Experimental Animal Centre of Shanghai University of Traditional Chinese Medicine. The animals were housed in standard cages with wood shavings in an animal room with a carefully controlled ambient temperature (23 ± 1 °C, RH 20%) and artificial illumination (12 h light/dark cycle) and provided with standard diet. The animals were given access to water ad libitum throughout the study. This study was approved by the Ethical Committee of the Laboratory Animal Center, Shanghai University of Traditional Chinese Medicine. The protocol approval number is SYXK (Shanghai) 2014-0008.
Metabolic stability of liver microsomes
The 0 min (blank) samples were prepared by addition of an 80 µL aliquot of each incubation mixture to 300 µL quench reagent to precipitate proteins. The samples were vortexed, and then a 20 µL aliquot of the nicotinamide adenine dinucleotide phosphate hydrate (NADPH) regenerating system was added. The reaction was initiated by the addition of 80 µL of the NADPH regenerating system to 320 µL of each incubation mixture. The final incubation conditions achieved in 400 µL were 0.5 mg/mL microsomal protein, 1 µM test article/positive control, 1.3 mM NADP, 3.3 mM glucose-6-phosphate, and 0.6 U/mL glucose-6-phosphate dehydrogenase. The mixtures were incubated in a 37 °C water bath with gentle shaking. A 100 µL aliquot of each mixture was removed at 10, 30, and 90 min and placed in a clean 96-well plate, which contained 200 µL quench reagent to precipitate proteins, and then centrifuged (4000 ×g, 10 min). In total, 80 µL of supernatant was taken into 96-well assay plates pre-added with 160 µL ultrapure water and then analyzed by liquid chromatography–tandem mass spectrometry (LC-MS/MS). The slope was measured by plotting the logarithm of the remaining percentage of the compound with time. The metabolic half-life T1/2 and intrinsic clearance CLint were calculated according to the following formula, where V/M equals 1/protein concentration [Citation46,Citation47]:
Plasma protein binding
The plasma protein binding study for paeonol was carried out according to rapid equilibrium dialysis (RED) [Citation48,Citation49]. Plate with the RED device inserts were used for dialysis with plasma and buffer. Plasma incubation mixture by spiking compounds was prepared to a final concentration of 2 µM. In total, 300 μL of plasma sample was transferred to the red ring chamber, and 500 μL of buffer to the opposite side chamber (n = 2), which was covered with a membrane and incubated at 37 °C at 100 rpm in an orbital shaker for 4 h. Equal volumes (50 µL) from both plasma and buffer chambers were added into a 96-well plate. An equal volume of blank plasma or phosphate-buffered saline (PBS) was added to the required wells to create analytically identical sample matrix. For each sample, 200 μL acetonitrile solution containing internal standard (IS) was added and then centrifuged (4000 × g, 10 min). Quantitative analysis was carried out by LC-MS/MS. The fraction of bound drug was determined by the following equation:
where f is the fraction of bound paeonol in plasma and Cu and Ct are the unbound and total concentrations of the paeonol in plasma, respectively.
Preparation of the Pae-SMEDDS
As for the poor solubility of paeonol, which is unfavourable for the local treatment of colonic disease. In order to improve the dissolution of paeonol as well as its oral bioavailability, SMEDDS was utilized for paeonol preparation. The prescription of SMEDDS for 20(S)-protopanaxadiol (20(S)-PPD), with the same property of the poor solubility in our previous study [Citation50] was referred, whose formulation was composed with 20(S)-PPD (10.0%), oleic acid (22.7%), Labrafac Lipophile WL 1349 (4.3%), Transcutol HP (17.3%), Labrasol (34.3%), and Cremophor RH40 (11.4%). In this study, the drug-loading efficiency was increased by 5%, compared with the above-mentioned study. The proportion of prescriptions for drugs and excipients was as follows: paeonol (15.0%), oleic acid (21.1%), Labrafac Lipophile WL 1349 (4.0%), Transcutol HP (16.4%), Labrasol (32.5%), and Cremophor RH40 (11.0%). These materials (n = 3) were placed in a 100 ml conical flask with plug and then magnetically stirred for 30 min at 400 rpm and 40 °C. The drug loading of three batches of samples was determined by high-performance liquid chromatography (HPLC).
Characterization of Pae-SMEDDS
Before the test, Pae-SMEDDS samples were taken and mixed with 200-fold volume water at 40 °C and then magnetically stirred for microemulsion at 400 rpm. The size, polydispersity coefficient (PDI), and zeta potential of the microemulsion were measured using a Nano ZS90 laser particle size analyzer (Malvern Instruments Co., Ltd., Malvern, UK).
Transmission electron microscope (TEM) was used to characterize the morphology of the reconstituted SMEDDS [Citation51,Citation52]. Microemulsion droplets were obtained by emulsifying Pae-SMEDDS in deionized water. A drop of diluted Pae-SMEDDS was spread on a 230-mesh copper grid coated with carbon film and negatively stained with 2% phosphotungstic acid for 30 s. The grid was dried at room temperature and then observed by TEM (TECNAI G2S-TWIN, Hillsboro, OR), whose voltage was 200 kV.
Dissolution of Pae-SMEDDS
Pae-SMEDDS was filled into gastric-capsules, filling 0.060 g into every capsule with a drug loading of 15%. The dissolution studies were carried out according to dissolution test specifications of the Chinese pharmacopoeia (2015 edition, basket method) as follows: the dissolution flasks were immersed in a water bath at 37 °C and filled with 150 ml of pH 7.8 PBS. At 100 rpm rotation speed and at different time intervals (1, 3, 5, 10, 15, 20, 30, 45, and 60 min), 2 ml of dissolution media was withdrawn and filtered using 0.45 µm polyethersulphone membrane filters. Equal volume of fresh media was supplemented into the corresponding flasks. The amount of paeonol was determined by HPLC. The cumulative dissolution was calculated, and the dissolution profile was also drawn. Each experiment was performed in triplicate.
Preparation of paeonol colon-specific capsule
Pae-SMEDDS was encapsulated into the colon-targeted capsules through a 1 ml syringe with a needle, filling 0.025 g into every capsule with a drug loading of 15%, and the capsule shell was covered. The obtained capsule was named Pae-SMEDDS-loaded colon-specific capsule (Pae-SME-CSC).
Drug release studies of Pae-SME-CSC
The release behaviour of Pae-SME-CSC was studied by basket method of the 2015 edition of Chinese Pharmacopoeia. The first stage was to release the medium in artificial gastric juice for 2 h and then change it to artificial intestinal fluid and release for 3 h. The medium was replaced with artificial colonic fluid in the last stage. The rotative velocity was 100 rpm, media temperature was 37 ± 0.5 °C, and 2 ml of released media was obtained at 2, 3, 4, 5, 6, 8, 10, and 12 h. Equal volume of fresh media (37 ± 0.5 °C) was supplemented into the corresponding flasks immediately. The amount of paeonol was determined by HPLC [Citation26]. The cumulative release was calculated, and the release profile was drawn.
Stability study
Pae-SME-CSC was placed under the condition of high temperature, high humidity, and strong illumination for 10 d. The appearance, contents, and particle size of samples were measured at 0, 5, and 10 d, respectively. The results were compared with those at day 0, and the effects of temperature, humidity and light on the stability of the drug were determined.
Pharmacokinetic study
Taking paeonol-gastric capsule (Pae-GC) as reference preparation (100 mg paeonol powder into every gastric-soluble capsule), the pharmacokinetic study of Pae-SME-CSC was carried out. Twelve rats were divided into two groups randomly, with six rats in each group, and given a single 100 mg/kg dose of Pae-GC and Pae-SME-CSC by intragastric administration. In total, 0.3 ml blood samples was collected from the retro-orbital vein and placed in anticoagulant EP tubes at the intervals of 0.25, 0.5, 1, 2, 3, 4, 6, 8, 12, 24, 36, and 48 h after single oral administration. The heparinized blood samples were immediately centrifuged at 5000 rpm for 10 min at 4 °C, and then the supernatant was obtained as the plasma analyte. Pharmacokinetic parameters were calculated from the plasma concentration–time data [Citation53].
Evaluation of colon-targeting property
Seventy rats were used in the experiment to evaluate the distribution of colon in rats after intragastric administration of Pae-SME-CSC. The rats were randomly divided into two groups and given a single 100 mg/kg dose of Pae-GC or Pae-SME-CSC. At 0, 2, 4, 6, 8 12, and 24 h after intragastric administration, each animal (n = 5 for each time point) was sacrificed by using ether anesthesia to, and colon samples were collected and weighed. Tissue samples were mixed with physiological saline to create 0.5 g/mL tissue homogenates. The tissue homogenates were stored at −80 °C until LC-MS/MS analysis. To evaluate the effect of Pae-SME-CSC in colon-targeted drug delivery, the tissue–time profiles of Pae-GC and Pae-SME-CSC were compared.
In total, 100 μL of plasma sample solution or tissue homogenate supernatant was taken into a 1.5 ml centrifuge tube, and 200 μL acetonitrile (containing IS 44.52 ng) was added to precipitate proteins. Subsequently, the obtained samples were vortexed for 60 s and centrifuged at 15,000 rpm for 10 min. The supernatant was placed in another 1.5 ml EP tube and centrifuged again. The final supernatant samples were placed in amber autosampler vials for LC-MS/MS analysis [Citation54].
LC-MS/MS analysis
The amount of paeonol in plasma and tissue samples was determined by LC-MS/MS. MS was performed on an Agilent 6460 Series Triple-Quadrupole Tandem Mass Spectrometer (Agilent Corporation, Santa Clara, CA) equipped with an electrospray ionization (ESI) source operated in positive-ion mode. For the ultra high-performance liquid chromatography analysis, an ACQUITY UPLCTM HSS C18 column (100 mm × 2.1 mm, 1.8 μm, Waters Co., Milford, MA) was used. Approximately 0.1% aqueous formic acid:acetonitrile = 35:65 (v/v) was used as mobile phase at a flow rate of 0.4 ml/min. The column temperature was maintained at 25 °C, the sample-tray temperature was kept at 4 °C, and the injection volume was fixed at 5.0 µL.
MS was performed on an Agilent 6460 Series Triple-Quadrupole Tandem Mass Spectrometer (Agilent Corporation, Santa Clara, CA) equipped with an ESI source operated in positive-ion mode. The optimized source parameters were set as follows: drying gas temperature, 350 °C; drying gas flow, 10.0 L/min; nebulizing gas pressure, 35 psi; and capillary voltage, 4000 V. Quantification was achieved using multiple reaction monitoring mode of ion pair at m/z 167.1–121.0 for paeonol and ion pair at m/z 267.1–225.9 for magnolol (IS).
Effects on UC
The rats were divided into seven groups randomly (n = 10/group): normal group (blank), model group, SASP group (500 mg/kg), Pae-GC 1 group (100 mg/kg), Pae-GC 2 group (200 mg/kg), Pae-SME-CSC 1 group (100 mg/kg), and Pae-SME-CSC 2 group (200 mg/kg). The UC model in rats was induced by 5% (w/v) TNBS-ethanol solution similar to our previously reported method [Citation26]. After 24 h of colitis induction, rats were given intragastric administration as follows: the normal group and model groups received 0.5% sodium carboxylmethyl cellulose solution at a dose of 10 ml/kg. The other treatment groups were given the corresponding dose of drugs, for seven consecutive days. Anti-inflammatory effects were evaluated by the disease activity index (DAI), colon weight/length ratio, and macroscopic and microscopic damage scores [Citation26]. Serum interleukin (IL)-17, IL-6, and transforming growth factor beta 1 (TGF-β1) levels were determined by ELISA. All data were presented as the means ± standard deviation (SD).
Statistical analyses were carried out by using SPSS 21.0 (IBM, New York, NY) for Windows. DAI, macroscopic, and histopathological scores were compared by Kruskal–Wallis non-parametric test. Other parameters were compared by one-way analysis of variance followed by least significant difference t-test, and p < .05 was considered statistically significant.
Results
Metabolic stability of liver microsomes
The results showed that the T1/2 of paeonol in human and rat liver microsomes were 20.5 min and 60.4 min, respectively, and CLint were 67.5 and 22.9 μL/min/mg, respectively.
Plasma protein binding
The bound fractions of paeonol were calculated. At 2 μM concentration, the average plasma protein binding rates of paeonol with human and rat plasma were 93.04% and 90.60%, respectively.
Characterization of Pae-SMEDDS
The prepared Pae-SMEDDS was a transparent light yellow, oily solution, with a drug loading of 14.9 ± 0.13%. The particle size, PDI, and zeta-potential of Pae-SMEDDS were 79.71 ± 1.92 nm, 0.184 ± 0.03, and −20.5 ± 1.40 mv, respectively. The morphology of the reconstituted Pae-SMEDDS is shown in . It was observed that the microemulsion droplets of approximately 50–100 nm, were homogenous, uniform spherical with good integrity, and segregated at particular distance.
Drug release study in vitro
The cumulative dissolution of Pae-SMEDDS was determined, and the dissolution profile was drawn (). The result showed that the cumulative dissolution of Pae-SMEDDS in pH 7.8 PBS was 80% when the dissolution time was 15 min, and the cumulative dissolution remained unchanged at 60 min.
Figure 2. (A) Dissolution curves of Pae-SMEDDS in PBS (pH 7.8) (n = 3). (B) Release curve of Pae-SME-CSC in vitro (mean ± SD, n = 6). (C) Mean plasma concentration–time profiles of paeonol after intragastric administration of Pae-GC and Pae-SME-CSC (mean ± SD, n = 6). (D) Mean tissue concentration–time profiles of paeonol in the colon after intragastric administration of Pae-GC and Pae-SME-CSC (mean ± SD, n = 5). (E) Colon weight/length ratio in the seven groups (n = 10). Values are statistically significant at *p < 0.5 versus the model group, **p < .01 versus the model group. (F) Macroscopic damage changes of the distal colon in the seven groups (n = 10): (a) normal group, (b) model group, (c) Pae-GC 1 group, (d) Pae-SME-CSC 1 group, (e) Pae-GC 2 group, (f) Pae-SME-CSC 2 group, and (g) SASP group.
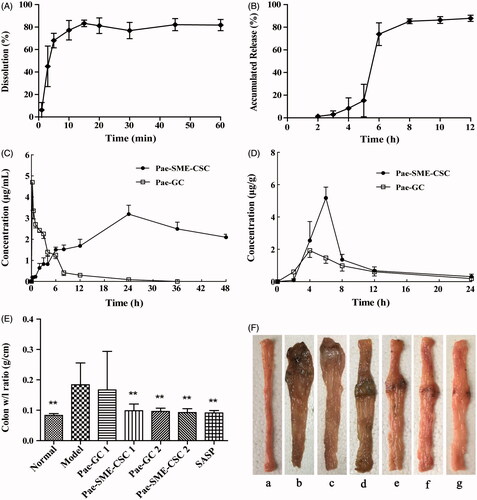
The cumulative release of Pae-SME-CSC in sequent different media was calculated, and the release profile was drawn (). In vitro release results showed that the Pae-SME-CSC was not released in the artificial gastric media within the first 2 h. Only 20% of the drug were released in the artificial intestinal media in the following 3 h, but gradually released within the first hour in the artificial colonic fluid. The release rate was close to 90% after 8 h, and eventually the drug was completely released.
Stability study
The effects of temperature, humidity, and illumination on the stability of Pae-SME-CSC in 10 d were investigated. The results are shown in . On the 10th day, under the condition of illumination (4500 ± 500 lx) and high temperature (40 °C), the drug content remained above 90%, but the particle size and PDI of Pae-SMEDDS placed in 40 °C were obviously increased unlike at day 0. The above results indicated that the stability of the preparation was less affected by light, but high-temperature storage should be avoided. In addition, under the condition of RH 92.5% ± 5%, the capsule was obviously hygroscopic, and the drug content decreased to 77.5%. When RH was 75% ± 5%, the drug content remains above 90%, but the shell of the capsule showed hygroscopic deformation. Therefore, colon-specific capsule should be stored in a dry place below 40 °C.
Table 1. Stability results of pae-SME-CSC (n = 3).
Method validation via in vivo detection
The results showed that blank plasma and tissue homogenate did not interfere with the determination of paeonol and IS. The limit of detection and the limit of quantitation of paeonol were measured at signal-to-noise ratios of 3 and 10, and the values were 30 and 100 ng/mL, respectively. The peak area ratios of paeonol to IS showed good linearity over the concentration range of 0.11–6.91 μg/mL (r = 0.9985). In low, medium, and high concentrations, the precision, accuracy, and extraction recovery were determined. The relative standard deviations (RSDs) of paeonol ranged from 5.2% to 8.2% for precision. The accuracies were 102%, 103.8%, and 99.4%, and RSDs were less than 10.3%. The extraction recoveries of paeonol were 108.6%, 101.8%, and 91.9%, and RSDs were less than 8.4%.
Pharmacokinetic study
Plasma concentration–time profiles of paeonol after intragastric administration of colon-specific capsule and reference preparation is shown in . As shown in the figure, the reference group reached the maximum plasma concentration (Cmax) of 4.70 µg/mL after 0.25 h intragastric administration. The drug was almost eliminated after 24 h and became undetectable in the plasma after 36 h. In the colon-specific capsule group, the plasma concentration was higher than that in the reference group at 6 h after administration, and Cmax of 3.19 µg/mL was reached after 24 h. Until the end of 48 h sampling after administration, plasma concentration remained at a relatively high level of 2.10 µg/mL.
The pharmacokinetics parameters are shown in . They showed that the peak time of blood concentration Tmax, elimination half-life t1/2, and mean residence time MRT0–t of the colon-specific capsule group were significantly prolonged, and the area under the concentration–time curve AUC0-t was obviously increased. The relative bioavailability F was increased by approximately six times compared with the reference preparation. This finding indicated that Pae-SME-CSC displayed sustained-release characteristics and obvious effect in improving bioavailability.
Table 2. Pharmacokinetics parameters of paeonol after intragastric administration of pae-SME-CSC and its reference preparation (Pae-GC) (n = 6).
Evaluation of colon-targeting property
Tissue concentration–time profiles of paeonol after intragastric administration of the Pae-SME-CSC and Pae-GC groups are shown in . The results of drug distribution in colon tissue showed that paeonol could be detected in colonic tissue after 2 h intragastric administration in the Pae-GC group, and the content was 0.6 µg/g. The maximum tissue concentration (1.92 µg/g) was reached after 4 h. In the Pae-SME-CSC group, the colonic tissue concentration of paeonol was detected as 2.54 µg/g after 4 h administration, and reached the maximum tissue concentration of 5.18 µg/g was reached after 6 h. In addition, paeonol could still be detected in colonic tissue until the end of 24 h sampling after administration. The area under the concentration–time curve (AUC) of the Pae-SME-CSC group was significantly higher than that of the Pae-GC group, and the maximum tissue concentration and Tmax were 2.7 times and 1.5 times higher than those of the Pae-GC group, respectively.
Effects on UC
During the experiment, no animal died. The statistical results of the experiment are shown in . No statistical difference existed in scores of DAI between the Pae-GC 1 group and the model group (p > .05). Compared with the model group, the scores of DAI were significantly decreased in the colon-specific capsule 1 group, Pae-GC 2, Pae-SME-CSC 2, and SASP groups (p < .01 or p < .05), suggesting that symptoms were relieved to a certain extent. Compared with the normal group, colon w/l ratio (0.184 ± 0.072 g/cm) was increased significantly in rats of the model group (p < .01), indicating that modeling by TNBS clysis resulted in colon congestion, edema and shortening. Consequently, the colon w/l ratio was increased and modeling was successful. Compared with the model group, the colon w/l ratio showed no significant change in the Pae-GC 1 group (p > .05). However, it was decreased significantly in the Pae-SME-CSC 1, Pae-GC 2, Pae-SME-CSC 2, and SASP groups (p < .01), suggesting that colon congestion and edema were relieved ().
Table 3. DAI score, colon weight/length ratio, and macroscopic and microscopic damage scores of all the groups (n = 10).
The macroscopic damages of the colon showed that in the normal group, textures of folds and mucous membranes were smooth on the colons, where no ulcer, erosion, congestion, or edema was observed. In the model group, the colons heavily adhered to surrounding tissues in most rats that they could be hardly divested. Moreover, tissues were very brittle that they were easily damaged. Segmental circular ulcer and thicker intestinal walls were observed at approximately 8 cm away from the anus. Brown pseudomembrane-like sphacelus adhered to the surface of ulcers, and the macroscopic damage scores were as high as 4.40 ± 1.17. In the paeonol 1 group, the colons of rats were observed adhered to each other in different degrees, whereas congestion, edema, and ulcer were observed on the intestinal walls. Compared with the model group, the improvement was not significant, whereas no statistical difference was detected in the macroscopic damage scores between the Pae-GC 1 and model groups (p > .05). By contrast, the colons generally did not adhere to each other in the Pae-SME-CSC 1, Pae-GC 2, Pae-SME-CSC 2, and SASP groups, where congestion and edema were significantly relieved. Additionally, mild ulcers were observed in these groups (). Compared with the model group, macroscopic damage scores in these treated groups were also significantly decreased (p < .01 or p < .05).
As observed from the microscopic damage, the intestinal epithelial cells were intact, and the glands were arranged in order in the normal group, where neutrophil infiltration was invisible from the mucous layer to the serous layer. In the model group, colonic ulcers were developed, accompanied by proliferation of granulation tissues, defects, and abscission of most mucous membranes and glands. Many neutrophils infiltrated deeply into the muscular layer and even invaded into the serous layer, where the scores of microscopic damages on the colons were up to 3.90 ± 0.99. Neutrophil infiltration was highly visible on the mucous layer, submucosa, and mucous layer in the Pae-GC 1 group, where the scores of microscopic damages reached 3.40 ± 1.35. However, no statistical difference was observed unlike that of the model group (p < .05). On the mucous layer and submucosa in the Pae-SME-CSC 1 group, severe neutrophil infiltration was detected, whereas oedema was observed on the submucosa and slight neutrophil infiltration on the mucous layer. In this group, scores of microscopic damage were 2.90 ± 1.45 and exhibited certain statistical difference compared with those of the model group (p < .05), suggesting that ulcer tissues recovered very well. Some neutrophil infiltrations were observed on the mucous layer and submucosa in the Pae-GC 2, Pae-SME-CSC 2 and SASP groups, whereas edema was detected on the submucosa, but no significant anomaly was found on the muscular layer (). Compared with the model group, the scores of microscopic damages declined significantly (p < .01) in these groups, suggesting that ulcers were significantly relieved.
Figure 3. Microscopic damage changes (A, H&E stain, ×400; and B, H&E stain, ×100) of the distal colon in seven groups (n = 10): (a) normal group, (b) model group, (c) Pae-GC 1 group, (d) Pae-SME-CSC 1 group, (e) Pae-GC 2 group, (f) Pae-SME-CSC 2 group, and (g) SASP group.
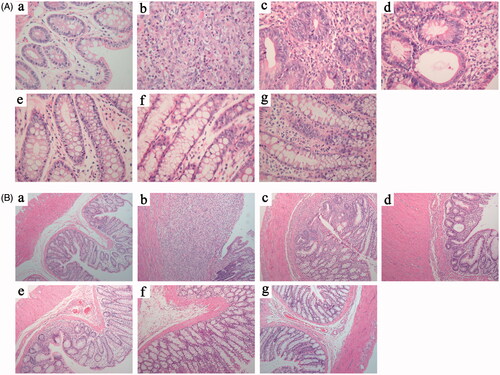
The results of ELISA detection showed that in the model group, the concentrations of IL-17 (21.97 ± 5.01 pg/mL) and IL-6 (0.470 ± 0.348 ng/mL) in rat serum were the highest, whereas TGF-β1 level (56.48 ± 5.55 ng/mL) was the lowest. Compared with the model group, no significant difference was observed in the concentrations of IL-17, IL-6, and TGF-β1 in the Pae-GC1 group (p > .05). In the Pae-SME-CSC 1 group, the concentration of IL-17 and IL-6 declined, whereas that of TGF-β1 increased (p < .05). In the Pae-GC 2, Pae-SME-CSC 2, and SASP groups, the concentration of IL-17 and IL-6 declined significantly (p < .01 or p < .05), whereas that of TGF-β1 increased significantly (p < .01) ().
Discussion
In this study, SMEDDS was used to fill the colon-specific capsules, which was an isotropic and thermodynamically transparent stable solution consisting of oil, surfactant, co-surfactant, and drug mixtures. It has many properties that make it appealling as a universal vehicle for insoluble lipophilic drug delivery. Previous reported studies illustrated that SMEDDS can enhance the solubility, dissolution, and oral aborption of insoluble drugs, including artemether and lumefantrine [Citation55], atorvastatin calcium [Citation56], terbinafine [Citation57], and methoxyflavones [Citation58]. SMEDDS can form fine oil-in-water droplets with a diameter size of less than 50 nm under mild agitation in the gastrintestinal tract. The increased surface area and small droplets allow transportation of the dug substance through the unstirred water layer to the gastrointestinal membrane, significantly increasing drug absorption. The potential advantages of SMEDDS include not only increasing drug solubility but also improving release and absorption properties through enhancing permeation across the inflamed mucosal tissues and droplet size reduction, providing a large interfacial surface area for drug absorption in the colon site [Citation59]. Recently, some studies [Citation2,Citation60,Citation61] have reported the application of SMEDDS in the development of colon-specific drug delivery system. However, systematic researches involving the preparation and in vitro/in vivo evaluations, especially studies on the in vivo colon-targeting property, the pharmacokinetic activity, and the anti-inflammatory effects on UC, are unsufficient. Therefore, in the present study, the adaptability of SMEDDS in the inflammtory colon region was investigated. As expected, the results showed that the liquid Pae-SMEDDS was delivered to the target region (colon), formed self-microemulsified droplets (79.71 ± 1.92 nm), and displayed therapeutic effects on the inflammed tissues.
In contrast to microemulsions, SMEDDS contains no water, and thus, it displays improved physical and/or chemical stablity even with long-term storage. In the stability inverstigation of this study, it is showed that Pae-SME-CSC had relatively stable content (95.8%) after 10 d under 40 °C as paeonol is known to be a volatile organic compound [Citation62]. Most of the SMEDDS formulations are in capsule or tablet dosage forms; thus, the occupied volume is small enough for administration, which improves the patient compliance and palatability [Citation63,Citation64]. Moreover, the results in this study also showed that the application of SMEDDS had effectively increased the release of paeonol in vitro and in vivo, which indicated its ability to facilitate rapid oral absorption of the loaded drug, resulting in the quick onset of action. This finding was also confirmed by another similar report [Citation65].
In this study, we also investigated the metabolic stability of liver microsomes and plasma protein binding of paeonol, which helped to obtain in vivo biological properties of paeonol, along with the pharmaceutical information already gained in our previous study. Results of metabolic stability of liver microsome study showed that the T1/2 of paeonol in human and rat liver microsomes were 20.5 min and 60.4 min, whereas CLint were 67.5 and 22.9 μL/min/mg, respectively. This finding suggested that paeonol was metabolized moderately in rat liver microsomes and rapidly in human liver microsomes. At the concentration of 2 μM, the average plasma protein binding rates of paeonol with human and rat plasma were 93.04% and 90.60%, respectively. This result indicated that the plasma protein binding of paeonol was similar between humans and rats, with high binding rate with plasma proteins.
The simultaneous application of in vitro and in vivo investigation is a general approach in the overall evaluation of colon-specific delivery system [Citation66,Citation67]. In this study, we evaluated the colon-targeting property of Pae-SME-CSC by investigating the release behavior in vitro and the distribution of the colon tissue in vivo. In vitro release results showed that Pae-SME-CSC was not released in the artificial gastric juice within the first 2 h, and only 20% of the drug were released in the artificial intestinal juice in the following 3 h but gradually released within the first hour in the artificial colonic fluid. The release rate was close to 90% after the 8 h, and eventually the drug was completely released, which indicated that Pae-SME-CSC could achieve the expected colon-specific effects in vitro with the application of colon-specific capsules. The results of drug distribution in colon tissue at different intervals showed that AUC of colon-specific capsule was significantly higher than that of the Pae-GC group, and the maximum tissue concentration and Tmax were 2.7 times and 1.5 times higher than those of the reference group, respectively. In addition, paeonol could still be detected in colonic tissue until the end of sampling (24 h), suggesting that the drug of the reference group may have been released in the stomach and small intestine. Given the first pass effect, the amount of drug that reached the colon was decreased. However, in the Pae-SME-CSC, after gastric emptying (2 h) and transportation to the small intestine transporting (2–4 h), most of the drug were not released before reaching the colon. The first pass effect was supposed to be effectively reduced, so that more drugs were enriched in the colon site. Pae-SME-CSC exhibited good localized drug release effect in the colon.
In this study, Pae-GC was used as a reference preparation, and LC-MS/MS was used to investigate the pharmacokinetic behavior of Pae-SME-CSC in rats. The results showed that Cmax of Pae-SME-CSC was close to the same dose of Pae-GC. However, the peak time of blood concentration (Tmax), elimination half-life (t1/2) and mean residence time (MRT0–t) of Pae-SME-CSC were significantly prolonged, and AUC0–t was obviously increased. Moreover, the relative bioavailability (F) was increased by six times compared with the reference preparation. These results strongly indicated that Pae-SME-CSC presented a similar sustained-released property and obviously improved bioavailability.
With the same dose of paeonol as a comparison, the anti-inflammatory effects of Pae-SME-CSC on UC in vivo were systematically evaluated. The results showed that the Pae-GC 1 group (100 mg/kg) displayed no significant effect on UC (p > .05), whereas the Pae-SME-CSC group had a better anti-UC effects at the same dosage (p < .01 or p < .05), whose efficacy was close to that of the Pae-GC 2 group (200 mg/kg) (p > .05), when compared with the model group. The effective dose of Pae-GC group was 200 mg/kg, whereas that of Pae-SME-CSC group was 100 mg/kg. The results indicated that the preparation had achieved the purpose of reducing the effective therapeutic dose [Citation68]. In addition, the concentration of cytokines in the serum of rats showed that the model group rats presented higher serum levels of IL-17 and IL-6 but lower levels of TGF-β1 than the rats in other groups. However, the normal group was the opposite. Studies showed that IL-17 and IL-6 are pro-inflammatory factors, and TGF-β1 is an anti-inflammatory factor [Citation69,Citation70].
As mentioned in our previous study mentioned, the therapeutic efficacy of paeonol on UC is dose dependent and closely associated with the regulation of Treg/Th 17 balance, which has also been indicated in the other studies [Citation71–73] on anti-UC effects. Th17 cells are included to the CD4 + T-cell subset and are characterized by secretion of IL-17, which plays an important role in the intestinal mucosal immune response and inflammatory process [Citation74]. Treg cells could suppress the inflammatory response cascade and maintain the balance of the intestinal immune response by secreting and regulating anti-inflammatory cytokines (e.g. TGF-β1). Compared with the model group in this study, the levels of IL-17 and IL-6 in rat serum were significantly reduced, whereas TGF-β1 was increased in the Pae-SME-CSC 1 pae-GC 2, and Pae-SME-CSC 2 groups, which were significantly improved on anti-UC effects. Therefore, the results in this study were consistent with the previous results, indicating that paeonol may inhibit colitis inflammation by regulating the balance of Treg/Th17 via downregulating Th17 cells and upregulating Treg cells. Other research reports demonstrated that Treg/Th 17 imbalance is also presented in many inflammatory and autoimmune diseases, such as rheumatoid arthritis [Citation75–77], which might be associated with the anti-inflammatory effects of paeonol on rheumatoid arthritis.
Conclusion
In this study, paeonol, used as a model drug, was used to develop a nanoscale oral colon-specific formulation (Pae-SME-CSC) with the application of Pae-SME-SMEDDS. Systematical evaluations of Pae-SME-CSC, including the studies on the stability, in vitro and in vivo colon-targeting properties, pharmacokinetics, and anti-inflammatory effects on UC, were carried out. All experimental results showed that Pae-SME-CSC displayed satisfactory colon-specific properties in vitro and in vivo, relatively high bioavailability, and preferable anti-inflammatory activities on UC, as expected. This work has provided a new promising topical drug candidate for the clinical treatment of UC and a favourable formulation strategy for the development of insoluble bioactive components extracted from traditional Chinese medicine for the local treatment of colon diseases.
Disclosure statement
The authors report no conflicts of interest in this work.
Additional information
Funding
References
- Yang Y, He J, Suo Y, et al. Tauroursodeoxycholate improves 2,4,6-trinitrobenzenesulfonic acid-induced experimental acute ulcerative colitis in mice. Int Immunopharmacol. 2016;36:271–276.
- Li Q, Zhai W, Jiang Q, et al. Curcumin–piperine mixtures in self-microemulsifying drug delivery system for ulcerative colitis therapy. Int J Pharm. 2015;490:22–31.
- Agis ER, Savas B, Melli M. Impact of colonic mucosal lipoxin A4 synthesis capacity on healing in rats with dextran sodium sulfate-induced colitis. Prostaglandins Other Lipid Mediat. 2015;121:63–69.
- Trivedi PP, Jena GB. Ulcerative colitis-induced hepatic damage in mice: studies on inflammation, fibrosis, oxidative DNA damage and GST-P expression. Chem Biol Interact. 2013;201:19–30.
- Wang YH, Ge B, Yang XL, et al. Proanthocyanidins from grape seeds modulates the nuclear factor-kappa B signal transduction pathways in rats with TNBS-induced recurrent ulcerative colitis. Int Immunopharmacol. 2011;11:1620–1627.
- Samsami-Kor M, Daryani NE, Asl PR, et al. Anti-inflammatory effects of resveratrol in patients with ulcerative colitis: a randomized, double-blind, placebo-controlled pilot study. Arch Med Res. 2015;46:280–285.
- Vong LB, Mo J, Abrahamsson B, et al. Specific accumulation of orally administered redox nanotherapeutics in the inflamed colon reducing inflammation with dose-response efficacy. J Control Release: Off J Control Release Soc. 2015;210:19–25.
- Yun CS, Choi YG, Jeong MY, et al. Moutan cortex radicis inhibits inflammatory changes of gene expression in lipopolysaccharide-stimulated gingival fibroblasts. J Nat Med. 2013;67:576–589.
- Jamal J, Mustafa MR, Wong PF. Paeonol protects against premature senescence in endothelial cells by modulating Sirtuin 1 pathway. J Ethnopharmacol. 2014;154:428–436.
- Gong X, Yang Y, Huang L, et al. Antioxidation, anti-inflammation and anti-apoptosis by paeonol in LPS/d-GalN-induced acute liver failure in mice. Int Immunopharmacol. 2017;46:124–132.
- Fu PK, Yang CY, Huang SC, et al. Evaluation of LPS-induced acute lung injury attenuation in rats by aminothiazole-paeonol derivatives. Molecules. 2017;22:1605.
- Meng Y, Wang M, Xie X, et al. Paeonol ameliorates imiquimod-induced psoriasis-like skin lesions in BALB/c mice by inhibiting the maturation and activation of dendritic cells. Int J Mol Med. 2017;39:1101–1110.
- Zhang L, Tao L, Shi T, et al. Paeonol inhibits B16F10 melanoma metastasis in vitro and in vivo via disrupting proinflammatory cytokines-mediated NF-κB and STAT3 pathways. IUBMB Life. 2015;67:778–788.
- Li M, Tan SY, Wang XF. Paeonol exerts an anticancer effect on human colorectal cancer cells through inhibition of PGE2 synthesis and COX-2 expression. Oncol Rep. 2014;32:2845–2853.
- Liu J, Wang S, Feng L, et al. Hypoglycemic and antioxidant activities of paeonol and its beneficial effect on diabetic encephalopathy in streptozotocin-induced diabetic rats. J Med Food. 2013;16:577–586.
- Ye M, Yi Y, Wu S, et al. Role of paeonol in an astrocyte model of Parkinson’s disease. Med Sci Monit. 2017;23:4740–4748.
- Shi X, Chen YH, Liu H, et al. Therapeutic effects of paeonol on methyl-4-phenyl-1,2,3,6-tetrahydropyridine/probenecid-induced Parkinson’s disease in mice. Mol Med Rep. 2016;14:2397–2404.
- Lyu ZK, Li CL, Jin Y, et al. Paeonol exerts potential activities to inhibit the growth, migration and invasion of human gastric cancer BGC823 cells via downregulating MMP2 and MMP9. Mol Med Rep. 2017;16:7513–7519.
- Zhou HM, Sun QX, Cheng Y. Paeonol enhances the sensitivity of human ovarian cancer cells to radiotherapy-induced apoptosis due to downregulation of the phosphatidylinositol-3-kinase/Akt/phosphatase and tensin homolog pathway and inhibition of vascular endothelial growth factor. Exp Ther Med. 2017;14:3213–3220.
- Xu Y, Zhu JY, Lei ZM, et al. Anti-proliferative effects of paeonol on human prostate cancer cell lines DU145 and PC-3. J Physiol Biochem. 2017;73:157–165.
- Zhai KF, Duan H, Luo L, et al. Protective effects of paeonol on inflammatory response in IL-1beta-induced human fibroblast-like synoviocytes and rheumatoid arthritis progression via modulating NF-kappaB pathway. Inflammopharmacology 2017;25:523–532.
- Liu N, Feng X, Wang W, et al. Paeonol protects against TNF-alpha-induced proliferation and cytokine release of rheumatoid arthritis fibroblast-like synoviocytes by upregulating FOXO3 through inhibition of miR-155 expression. Inflamm Res. 2017;66:603–610.
- Lou Y, Wang C, Tang Q, et al. Paeonol inhibits IL-1beta-induced inflammation via PI3K/Akt/NF-kappaB pathways: in vivo and vitro studies. Inflammation. 2017;40:1698–1706.
- Choy KW, Lau YS, Murugan D, et al. Chronic treatment with paeonol improves endothelial function in mice through inhibition of endoplasmic reticulum stress-mediated oxidative stress. PLoS One. 2017;12:e0178365.
- Ma L, Chuang CC, Weng W, et al. Paeonol protects rat heart by improving regional blood perfusion during no-reflow. Front Physiol. 2016;7:298.
- Zong SY, Pu YQ, Xu BL, et al. Study on the physicochemical properties and anti-inflammatory effects of paeonol in rats with TNBS-induced ulcerative colitis. Int Immunopharmacol. 2017;42:32–38.
- Liu MH, Lin AH, Ko HK, et al. Prevention of bleomycin-induced pulmonary inflammation and fibrosis in mice by paeonol. Front Physiol. 2017;8:193.
- Xue P, Wang Y, Zeng F, et al. Paeonol suppresses solar ultraviolet-induced skin inflammation by targeting T-LAK cell-originated protein kinase. Oncotarget. 2017;8:27093–27104.
- Wu J, Xu L, Sun C, et al. Paeonol alleviates epirubicin-induced renal injury in mice by regulating Nrf2 and NF-kappaB pathways. Eur J Pharmacol. 2017;795:84–93.
- Fan HY, Qi D, Yu C, et al. Paeonol protects endotoxin-induced acute kidney injury: potential mechanism of inhibiting TLR4-NF-kappaB signal pathway. Oncotarget. 2016;7:39497–39510.
- He LX, Tong X, Zeng J, et al. Paeonol suppresses neuroinflammatory responses in LPS-activated microglia cells. Inflammation. 2016;39:1904–1917.
- Lin C, Lin HY, Chen JH, et al. Effects of paeonol on anti-neuroinflammatory responses in microglial cells. Int J Mol Sci. 2015;16:8844–8860.
- Chang CY, Fu E, Chiang CY, et al. Effect of paeonol on tissue destruction in experimental periodontitis of rats. Am J Chin Med. 2014;42:361–374.
- Ishiguro K, Ando T, Maeda O, et al. Paeonol attenuates TNBS-induced colitis by inhibiting NF-kappaB and STAT1 transactivation. Toxicol Appl Pharmacol. 2006;217:35–42.
- Jin X, Wang J, Xia ZM, et al. Anti-inflammatory and anti-oxidative activities of paeonol and its metabolites through blocking MAPK/ERK/p38 signaling pathway. Inflammation. 2016;39:434–446.
- Li M, Tan SY, Zhang J, et al. Effects of paeonol on intracellular calcium concentration and expression of RUNX3 in LoVo human colon cancer cells. Mol Med Rep. 2013;7:1425–1430.
- Harada M, Yamashita A. [Pharmacological studies on the root bark of paeonia moutan. I. Central effects of paeonol]. Yakugaku Zasshi. 1969;89:1205–1211.
- Luo JY, Zhong Y, Cao JC, et al. Efficacy of oral colon-specific delivery capsule of low-molecular-weight heparin on ulcerative colitis. Biomed Pharmacother. 2011;65:111–117.
- Yehia SA, Elshafeey AH, Elsayed I. Pulsatile systems for colon targeting of budesonide: in vitro and in vivo evaluation. Drug Deliv. 2011;18:620–630.
- Chen C, Jia F, Hou Z, et al. Delivery of paeonol by nanoparticles enhances its in vitro and in vivo antitumor effects. Int J Nanomed. 2017;12:6605–6616.
- Chen ZX, Li B, Liu T, et al. Evaluation of paeonol-loaded transethosomes as transdermal delivery carriers. Eur J Pharm Sci. 2017;99:240–245.
- Yao J, Zeng D, Zhang Y, et al. Effect of solvents on forming poly(butyl-2-cyanoacrylate) encapsulated paeonol nanocapsules. J Biomater Sci Polym Ed. 2017;28:240–256.
- Yao J, Zhang Y, Hu Q, et al. Optimization of paeonol-loaded poly(butyl-2-cyanoacrylate) nanocapsules by central composite design with response surface methodology together with the antibacterial properties. Eur J Pharm Sci. 2017;101:189–199.
- Li SS, Li GF, Liu L, et al. Optimization of paeonol-loaded microparticle formulation by response surface methodology. J Microencapsul. 2015;32:677–686.
- Li SS, Li GF, Liu L, et al. Evaluation of paeonol skin-target delivery from its microsponge formulation: in vitro skin permeation and in vivo microdialysis. PLoS One. 2013;8:e79881.
- Lin C, Miao Y, Qian M, et al. Enantioselective metabolism of flufiprole in rat and human liver microsomes. J Agric Food Chem. 2016;64:2371–2376.
- Hu T, Zhou X, Wang L, et al. Effects of tanshinones from Salvia miltiorrhiza on CYP2C19 activity in human liver microsomes: enzyme kinetic and molecular docking studies. Chem Biol Interact. 2015;230:1–8.
- Wang Y, Xie G, Liu Q, et al. Pharmacokinetics, tissue distribution, and plasma protein binding study of chicoric acid by HPLC-MS/MS. J Chromatogr B: Analyt Technol Biomed Life Sci. 2016;1031:139–145.
- Waters NJ, Jones R, Williams G, et al. Validation of a rapid equilibrium dialysis approach for the measurement of plasma protein binding. J Pharm Sci. 2008;97:4586–4595.
- Wang B, Pu Y, Xu B, et al. Self-microemulsifying drug delivery system improved oral bioavailability of 20(S)-protopanaxadiol: from preparation to evaluation. Chem Pharm Bull. 2015;63:688–693.
- Laxmi M, Bhardwaj A, Mehta S, et al. Development and characterization of nanoemulsion as carrier for the enhancement of bioavailability of artemether. Artif Cells Nanomed Biotechnol. 2015;43:334–344.
- Li F, Song S, Guo Y, et al. Preparation and pharmacokinetics evaluation of oral self-emulsifying system for poorly water-soluble drug Lornoxicam. Drug Deliv. 2015;22:487–498.
- Xiao Y, Zhang YH, Sheng YX, et al. LC-MS determination and pharmacokinetic studies of paeonol in rat plasma after administration of different compatibility of Su-Xiao-Xin-Tong prescriptions. Biomed Chromatogr. 2008;22:527–534.
- Xie Y, Zhou H, Wong YF, et al. Study on the pharmacokinetics and metabolism of paeonol in rats treated with pure paeonol and an herbal preparation containing paeonol by using HPLC-DAD-MS method. J Pharm Biomed Anal. 2008;46:748–756.
- Bhandari S, Bhandari V, Sood J, et al. Improved pharmacokinetic and pharmacodynamic attributes of artemether-lumefantrine-loaded solid SMEDDS for oral administration. J Pharm Pharmacol. 2017;69:1437–1446.
- Yeom DW, Son HY, Kim JH, et al. Development of a solidified self-microemulsifying drug delivery system (S-SMEDDS) for atorvastatin calcium with improved dissolution and bioavailability. Int J Pharm. 2016;506:302–311.
- Baheti A, Srivastava S, Sahoo D, et al. Development and pharmacokinetic evaluation of industrially viable Self-microemulsifying Drug Delivery Systems (SMEDDS) for terbinafine. Curr Drug Deliv. 2016;13:65–75.
- Mekjaruskul C, Yang YT, Leed MG, et al. Novel formulation strategies for enhancing oral delivery of methoxyflavones in Kaempferia parviflora by SMEDDS or complexation with 2-hydroxypropyl-β-cyclodextrin. Int J Pharm. 2013;445:1–11.
- Constantinides PP. Lipid microemulsions for improving drug dissolution and oral absorption: physical and biopharmaceutical aspects. Pharm Res. 1995;12:1561–1572.
- Zhang L, Zhu W, Yang C, et al. A novel folate-modified self-microemulsifying drug delivery system of curcumin for colon targeting. Int J Nanomed. 2012;7:151–162.
- Zhou J, Tan L, Xie J, et al. Characterization of brusatol self-microemulsifying drug delivery system and its therapeutic effect against dextran sodium sulfate-induced ulcerative colitis in mice. Drug Deliv. 2017;24:1667–1679.
- Li Y, Ma H, Wan Y, et al. Volatile organic compounds emissions from luculia pinceana flower and its changes at different stages of flower development. Molecules. 2016;21:531.
- Xiumin LI, Man GE, Minzi LU, et al. The in vitro and in vivo evaluation of fenofibrate with a self- microemulsifying formulation. Curr Drug Deliv. 2015;12:308–313.
- Duc Hanh N, Mitrevej A, Sathirakul K, et al. Development of phyllanthin-loaded self-microemulsifying drug delivery system for oral bioavailability enhancement. Drug Dev Ind Pharm. 2015;41:207–217.
- Taha E, Ghorab D, Zaghloul AA. Bioavailability assessment of vitamin A self-nanoemulsified drug delivery systems in rats: a comparative study. Med Princ Pract. 2007;16:355–359.
- Patel MM. Formulation and development of di-dependent microparticulate system for colon-specific drug delivery. Drug Deliv Transl Res. 2017;7:312–324.
- Singh S, Kotla NG, Tomar S, et al. A nanomedicine-promising approach to provide an appropriate colon-targeted drug delivery system for 5-fluorouracil. Int J Nanomed. 2015;10:7175–7182.
- Wachsmann P, Moulari B, Beduneau A, et al. Surfactant-dependence of nanoparticle treatment in murine experimental colitis. J Control Release. 2013;172:62–68.
- Xu L, Shen P, Bi Y, et al. Danshen injection ameliorates STZ-induced diabetic nephropathy in association with suppression of oxidative stress, pro-inflammatory factors and fibrosis. Int Immunopharmacol. 2016;38:385–394.
- Wang X, Yang X, Wen C, et al. Grass carp TGF-β1 impairs IL-1β signaling in the inflammatory responses: evidence for the potential of TGF-β1 to antagonize inflammation in fish. Dev Comp Immunol. 2016;59:121–127.
- Zhang M, Zhou Q, Dorfman RG, et al. Butyrate inhibits interleukin-17 and generates tregs to ameliorate colorectal colitis in rats. BMC Gastroenterol. 2016;16:84.
- Zhu XM, Huang YM, Fan JF, et al. Aberrant frequency of IL-10-producing B cells and its association with the balance of Treg/Th17 in children with inflammatory bowel disease. Pharmazie. 2015;70:656–660.
- Eastaff-Leung N, Mabarrack N, Barbour A, et al. Foxp3+ regulatory T cells, Th17 effector cells, and cytokine environment in inflammatory bowel disease. J Clin Immunol. 2010;30:80–89.
- Onishi RM, Gaffen SL. Interleukin-17 and its target genes: mechanisms of interleukin-17 function in disease. Immunology. 2010;129:311–321.
- Park JS, Oh Y, Park O, et al. PEGylated TRAIL ameliorates experimental inflammatory arthritis by regulation of Th17 cells and regulatory T cells. J Control Release: *off J Control Release Soc. 2017;267:163–171.
- Ma A, Yang Y, Wang Q, et al. Antiinflammatory effects of oxymatrine on rheumatoid arthritis in rats via regulating the imbalance between Treg and Th17 cells. Mol Med Rep. 2017;15:3615–3622.
- Yuan X, Tong B, Dou Y, et al. Tetrandrine ameliorates collagen-induced arthritis in mice by restoring the balance between Th17 and Treg cells via the aryl hydrocarbon receptor. Biochem Pharmacol. 2016;101:87–99.