Abstract
The aim of this study was to evaluate mannose-anchored thiolated chitosan (MTC) based nanocarriers (NCs) for enhanced permeability, improved oral bioavailability and anti-parasitic potential of amphotericin B (AmB). Transgenic Leishmania donovani parasites expressing red fluorescent protein DsRed2 and imaging-flow cytometry was used to investigate parasitic burdens inside bone marrow-derived macrophages ex vivo. Cytokine estimation revealed that MTC nanocarriers activated the macrophages to impart an explicit immune response by higher production of TNF-α and IL-12 as compared to control. Cells treated with MTC NCs showed a significantly higher magnitude of nitrite and propidium iodide (PI) fluorescence intensity in contrast to cells treated with AmB. Concerning to apparent permeability coefficient (Papp) results, the MTC NCs formulation displayed more specific permeation across the Caco-2 cell monolayer as compared to AmB. The half-life of MTC NCs was about 3.3-fold persistent than oral AmB used as positive control. Also, t oral bioavailability of AmB was increased to 6.4-fold for MTC NCs compared to AmB for positive control. Acute oral evaluation indicated that MTC NCs were significantly less toxic compared to the AmB. Based on these findings, MTC NCs seems to be promising for significant oral absorption and improved oral bioavailability of AmB in leishmaniasis chemotherapy.
Graphical Abstract
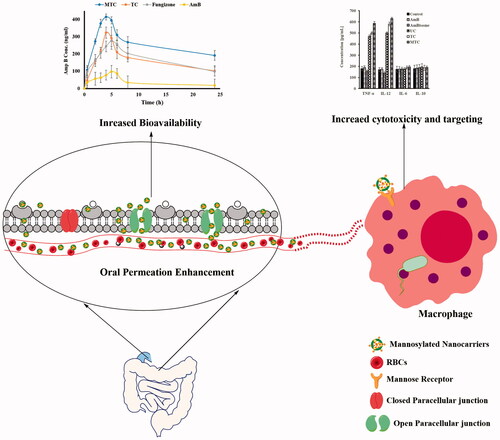
Introduction
Amphotericin B (AmB) is highly efficacious anti-leishmanial agent with 97% cure rate [Citation1]. Therefore, the clinical management of leishmaniasis is being shifted towards the use of AmB due to wide-spread resistance against the first line drugs, e.g. the antimonial compounds [Citation2]. However, marketed parenteral formulation of AmB (AmBisome®) has not reached its full therapeutic potential due to infusion-related side effects, renal toxicity and high cost. Moreover, renal toxicity following parenteral formulation is partially associated with excipients demanded in an injectable formulation [Citation3,Citation4]. Numerous endeavors have been intended for the development of targeted orally administered AmB formulations. Nevertheless, AmB ranked to the Class IV category of drugs regarding to the biopharmaceutics classification system, that is it has a low solubility and low membrane permeability due to its amphoteric characteristics [Citation5]. Thus, the aqueous solubility, membrane permeability and oral bioavailability of AmB required to be enhanced through appropriate delivery systems.
In recent years, advancements in the oral delivery of AmB have been focused on macrophage-targeted polymeric nanocarriers. In case of visceral leishmaniasis, the macrophages act as cellular reservoirs; therefore, liver spleen and lungs are amongst the highly parasitized pathological organs [Citation6]. Macrophage-targeted nanocarriers allow oral targeting to infected organs like liver, lungs and spleen while avoids organs of toxicity like the kidneys [Citation7]. Such a system is of particular interest in case of treatment of visceral leishmaniasis with AmB, a highly nephrotoxic drug. So far, the efficiency of such delivery systems, however, was limited by a rapid disintegration and/or erosion of the polymeric network. However, immobilization of thiol groups (–SH) on multifunctional polymeric backbone overcomes this essential shortcoming [Citation8,Citation9]. Because of the formation of inter- and intrachain disulfide bonds (–S–S–) during the swelling process, the stability of the thiolated (so-called thiomers) polymeric drug carrier matrix is strongly improved [Citation10].
Our research group has recently reported mannose-anchored thiolated polymeric-based AmB-loaded nanocarriers against Leishmania donovani (causing visceral leishmaniasis) and found very encouraging in vitro and in vivo anti-leishmanial efficacy [Citation11]. The thiomer-based nanocarriers were successful in localizing and retaining AmB in macrophages via increased macrophage uptake and P-gp pump inhibition. Thiomers are also well-known for their excellent mucoadhesion and permeation enhancement potential explored in various applications through different routes of administrations. The permeation-enhancing capability of thiomers is of special interest in the improvement of the oral bioavailability of poorly absorbed drugs including anti-infectious and anti-cancer agents [Citation12].
The present study was designed to further evaluate the potential of previously reported mannose-anchored thiomer-based AmB nanocarriers mainly for their permeation-enhancing properties, in vivo improvement in oral bioavailability, organ distribution, and acute oral toxicity. In addition, immunomodulation and anti-leishmanial mechanistic studies were conducted by using flow cytometry.
Materials
Chitosan (low molecular weight), sodium tripolyposhphate (TPP), thioglycolic acid (TGA), d-mannose and sodium borohydride were obtained from Sigma Aldrich (Germany), NADPH, hydroxylamine, EDAC, sodium cyanborohydride and Ellman’s reagent were purchased from Merck (USA).
Methods
Synthesis and characterization of nanocarriers
Complete detail about the synthesis of unmodified chitosan (UC) thiolated chitosan (TC) and mannose-anchored thiolated chitosan (MTC) nanocarriers loaded with AmB was adopted from our previous study [Citation11]. These nanocarriers were characterized for particle size, zeta potential, polydispersity and morphological studies via SEM analysis.
Animals, parasites and cell cultures
C57BL/6 mice and BALB/c mice were obtained from Harlan Laboratories (Indianapolis, IN). The mice were maintained in a pathogen-free animal facility at the Ohio State University (Columbus, OH, USA) in compliance with US National Institutes of Health and Institutional guidelines. Rabbits were purchased from National Institutes of Health, Islamabad and were maintained at Veterinary Research Institute. Pharmacokinetics evaluations by using rabbits were conducted according to protocol approved by the Bio-Ethical Committee of Quaid-i-Azam University Islamabad, Pakistan.
Dsred2-L. donovani were previously engineered from L. donovani strain LV82 expressing firefly luciferase and a red fluorescent protein [Citation13]. Expression of DsRed2 was confirmed in both promastigotes and intracellular amastigotes by fluorescent microscopy. Parasites were retained by serial passage of amastigotes isolated from the spleen of previously infected BALB/c mice. The animals were infected with 1 × 107 Dsred2-L. donovani amastigotes in 100 μL by intravenously into the tail vein.
Bone marrow-derived macrophages (BMDMs) were acquired according to previously published protocol [Citation14]. Briefly, tibia and femur bones of BALB/c mice were separated and marrow was flushed with PBS by using syringe. When recovering all bone marrow of all bones, centrifuge tubes containing the cells at 1500 rpm, for 7 min at 4 °C. Cells were recovered and plated at 1 × 106/ml [RPMI-1640 medium containing 10% (v/v) L199 cell supernatant, 10% fetal bovine serum (FBS), 2 mM l-glutamine, 50 μM 2-mercaptoethanol, and 1 × penicillin/streptomycin] in 75 cm3 flasks. After 6 days of plating, non-adherent cells were discarded and adherent macrophages were scraped from the flasks and plated at 0.5 × 106/ml in 24-well plates.
The human epithelial cell line Caco-2 has been extensively employed as a model of the intestinal epithelial barrier was obtained from American Type Culture Collection (ATCC® HTB-37™). Cells were fed with minimum essential medium (MEM) containing 20% FBS, 1% penicillin–streptomycin for 21 days.
Flow cytometry for parasitic loads evaluation
The differentiated BMDMs cells were plated into the 24-well plate at 3.5 × 106/well. After 24 h, DsRed2-L. donovani promastigotes were employed to infect BMDMs at a 1:10 ratio (1 macrophage/10 promastigotes), and the extracellular parasites were washed twice with fresh warm media. Infected BMDMs were incubated with nanoformulations of MTC, TC, UC and AmBisome®, dissolved in 100% DMSO and diluted in DMEM medium supplemented with 1% of LCCM (L0.929 cell condition medium), at 37 °C in a 5% CO2 incubator for 72 h. The maximum concentration of DMSO after dilution was 0.1% and BMDMs were treated with 1 µg/ml of native AmB or no drug constituted control groups. After 72 h, infected BMDMs were centrifuged at 1250 rpm for 5 min and resuspended in 0.5 ml of PBS, and the parasitic load was measured by flow cytometry to compute the proportion of dead/live parasites measured by the loss of fluorescence. Untreated or control treated with DMSO (0.5%) BMDMs infected with DsRed2-L. donovani were served as live cell control. The cells were washed in FACS-buffer, resuspended in 400 µL FACS-buffer and analyzed by a flow cytometer (FACS-Calibur II with CellQuest® Pro software, San Jose, CA). Acquisition velocity was fixed to low speed and the highest resolution, a programmed condition provided in flow cytometer. Cells were obtained depending on area and aspect ratio, gating out cell debris and free parasites from the examination. About 1000–5000 cells were gained. Channel 2 was used to gain DsRed2. Data were analyzed in FlowJo® software (Ashland, OR) after compensation of single color control samples employing a compensation matrix [Citation15].
Evaluation of cell membrane integrity
Leishmania donovani LV82 promastigotes aliquot up to 1 × 106 cells/100 μL were incubated with nanoformulations of MTC, TC, UC and AmBisome®, AmB dissolved in 100% DMSO and diluted in M-199 medium supplemented with 10% of FCS, at 28 °C for 72 h. After incubation, parasites were transferred into FACS tubes and washed two times by adding 2 ml of PBS (or HBSS), centrifuged at 1250g for 5 min, and then decanting the buffer from the pelleted cells. Cells were resuspended in 100 µL of Flow Cytometry Staining Buffer and incubated with 50 μL of propidium iodide (PI) (Sigma-Aldrich®, St. Louis, MO) 2 mg/ml at 25 °C, for 5 min. Membrane rupture permits the uptake of PI and its binding to cell DNA. Cell membrane integrity was evaluated conferring PI fluorescence, on BD FACS-Calibur flow cytometer, in which 10,000 events were acquired by CellQuest Pro software. Digitonin saponin 40 μM (Sigma-Aldrich®) was employed as positive control and the fluorescence intensity were compared with negative control non-stained fluorescence sample.
Cytokine ELISA
Immunomodulatory activity of MTC-based AmB-loaded nanocarriers in BMDMs isolated from C57BL/6 mice was determined using ELISA kit (Biolegend Inc., San Diego, CA) according to standard procedures provided by the manufacturer. Cells were treated with AmB (native drug) and nanoformulations of MTC, TC, UC and AmBisome® equivalent to their IC50 concentration, and untreated cells were considered as control. Supernatants were collected after 72 h of incubation at 37 °C and analyzed for the concentration of cytokines (TNF-α, IL-12, and IL-6) by ELISA plate reader at 405 nm [Citation16].
Quantification of nitric oxide
Cellular nitric oxide (NO) contents were estimated by measurement of nitrite (end product) concentration content in the culture supernatants from BMDMs cultured and treated for 72 h by the Griess assay. After ten min incubation, absorbance was evaluated using the ELISA plate reader at 540 nm. Results achieved were extrapolated from a standard curve plotted with NaNO2 at different concentrations (0–400 μM) [Citation16].
Mucoadhesion and water absorbing capacity
Mucoadhesion based on rheological synergism parameters for developed nanoformulations were evaluated by using cone-plate viscometer as previously described by [Citation17]. In addition, water absorption and swelling capacity of the synthesized nanocarriers were evaluated by using the protocol reported by our research group.
Permeation enhancing
Permeation of the thiomer-based nanocarriers was estimated by following the method described earlier [Citation18]. CaCo-2 cells were seeded onto 12-well transwell polyester plates with a pore size of 0.4 µM and 13.85 diameters. The cells were cultured in MEM medium supplemented with 20% (v/v) FBS and stored in a CO2 incubator at 37 °C with 5% CO2. The cells were grown and differentiated for 15 days with the change of media after every 48 h. Before the experiments, each monolayer was washed with 1 ml of PBS. To the apical chamber, 1 ml of transport medium and to the basal side, 2 ml of the medium was added. Nanoparticles were suspended in MEM without phenol red to give a final concentration of 1% (m/v). The whole assembly was kept at 5% CO2 for 30 min for the equilibrium. The media of the donor chamber (apical) was substituted with 0.5 ml of MEM without phenol and 0.5 ml of 1% (m/v) AmB-loaded nanocarriers. Aliquots of 100 µL were withdrawn from the acceptor chamber (basolateral) every 30 min over the period of 3 h and immediately replaced with an equal volume of transport medium. The apparent permeability co-efficient (Papp) for AmB were calculated as reported previously [Citation19].
In vivo bioavailability studies
Improvement in oral bioavailability and pharmacokinetics of AmB was evaluated in rabbits. The studies involving animals were performed by following the guidelines approved by the bioethics committee of Quad-i-Azam University, Islamabad, Pakistan. Healthy rabbits weighing 2000 ± 150 g were kept in the animal house with free access to water and food a day before the experiment. The rabbits were divided into six groups having five animals in each group. Group 1 was treated with pure AmB, Group 2, 3, 4 and 5 were administered with AmBisome, UC, TC and MTC nanocarriers, respectively, via oral gavage needle. Group 6 served as control and was given NS. From the ear marginal vein of each rabbit, the blood samples were withdrawn at predefined time intervals (0.5, 1, 2, 4, 6, 8, 12, 24, 36 and 48 h) with a sterile syringe. The samples were transferred to 1.5 ml Eppendorf-containing anticoagulant (100 µL). The plasma was separated from the collected samples by centrifugation at 4000 rpm for 15 min and stored at −20 °C until further use. AmB was extracted from the plasma and quantified by using a validated high-performance liquid chromatography (HPLC) method [Citation20].
Evaluation of toxicity and safety profile
The methodology for in vitro hemolysis assay, acute oral toxicity, serum biochemistry analysis, hematology analysis, organ-to-body ratio, histopathology of vital organs and tissue distribution analysis is provided in the Supplementary Material.
Statistical analysis
All the results were generated using two-way analysis of variance (ANOVA) to compare the results of different treatments with AmB, AmBisome, UC, TC and MTC. The results were further analyzed with Tukey’s test and Student’s t-test with level of significance p < .05. All the results are presented as mean ± standard deviation (S.D).
Results and discussion
Particle synthesis and characterization
TC and MTC nanocarriers loaded with AmB were successfully synthesized and characterized for different physicochemical parameters. The results for the particle size, zeta potential, polydispersity and AmB payload are shown in . The results indicated the successful synthesis of TC and MTC with AmB pay load above 70% with positive zeta potential.
Table 1. Physicochemical characterization of TC and MTC nanocarriers loaded with AmB.
Evaluation of parasitic load and cell membrane integration by flow cytometry
We investigated the proficiencies of flow cytometry in the evaluation of parasitic loads and determined macrophage infectivity intensities after infection of macrophages with L. donovani parasites. Leishmania donovani DsRed2 were used as a tool to detect parasites by fluorescence which is expressed only in viable parasites that permits us to precisely distinguish between viable and dead parasites. Flow cytometry analyses of AmB formulations (UC, TC, MCT and AmBisome) at six different drug concentrations (1–0.004 μg/ml AmB) were performed with BMDM-containing L. donovani DsRed2. The cytogram in was acquired with non-infected macrophages; it displayed a homogeneous population identified by a typical FL2-H red fluorescence lower than 101 units. The cytogram in was acquired with infected macrophages; it revealed two well-defined populations evident rendering to their comparative fluorescences. Non-infected macrophages exhibited a less fluorescence (lower than 101 units), while the relative fluorescence identified for L. donovani DsRed2-containing macrophages was higher than 101 units. Our results indicated that 31.9% of the BMDMs were infected in untreated controls, only 0.15% of them remained infected after treatment with 1 μg/ml MTC and 3.17, 8.96, 20.3 and 23.9% of them contained parasites after treatment with TC, UC, AmBisome and AmB, respectively. As shown in , the relative numbers of FL2-H red fluorescence events were measure and compared with the lower right quadrant gated percentage of red fluorescence. The intensity was decreased from 29.5% (untreated L. donovani DsRed2-containing macrophages; , lower right quadrants) to 15.7, 12.2, 7.70, 1.90 and 0.13% for AmB, AmBisome, UC, TC, and MTC, respectively. As decrease in florescence intensity is directly related to reduction of parasites inside the macrophages, indicating the higher efficacy of developed MTC nanoformulation than AmB.
Figure 1. (1) Evaluation of parasitic loads using flow cytometery of BMDMs infected with transgenic L. donovani parasites expressing the red fluorescent protein DsRed2. Treatment was conducted at concentration of 1 μg/ml for 72 h with AmB (C1), Ambisome (D1) and nanoformulation based on UC (E1), TC (F1) and MTC (G1). For non-infected BMDMs (A1) florescence intensity (FL2-H) was <101 units and florescence intensity (FL2-H) was higher than 101 units against infected BMDMs (B1). (2) Contour plots of flow cytometry analysis of PI-labeled L. donovani LV82 promastigotes. Untreated promastigotes: negative control (A2) and 25 μM digitonin as positive control (B2). Treatment was conducted at their inhibitory concentration (IC50) for 72 h with AmB (C2), Ambisome (D2) and nanoformulation based on UC (E2), TC (F2) and MTC (G2).
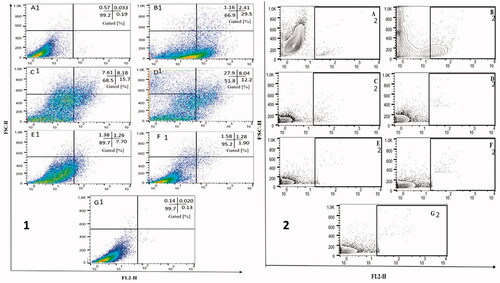
Due to the emergence of AmB-resistant parasites, it would be of concern to explore the pertinent cell death mechanism in MTC oral nanoformulation-treated L. donovani, hence that these could be used as a tool to evaluate the altering susceptibility of these parasites to AmB. The information achieved from the mechanistic study of killing would permit development of efficacious anti-leishmanial interventions toward the parasites and those displaying a kind of resistance to AmB. In order to evaluate, whether the mechanism of anti-parasitic potential triggered by AmB nanoformulations involves the necrotic death pathway, we determined plasma membrane integrity. Membrane integrity can also be determined through permeation of vigorous dyes such as PI. Permeation of PI in L. donovani LV82 promastigotes treated with AmB formulations (UC, TC, MCT and AmBisome) for 72 h was measured by flow cytometry. While parasites treated with 25 µM digitonin showed marked increase in the uptake of PI, revealing of membrane destruction, treatment with UC, TC, MCT and AmBisome did not persuade any significant changes in fluorescence intensity as shown in . Non-treated promastigotes (negative control) exhibited a less fluorescence (lower than 101 units) in , while the relative fluorescence identified for digitonin-treated promastigotes (positive control) was higher than 101 units conferring permeabilization of the plasma membrane, PI diffuse and bind to nucleic acids.
Figure 2. (A) NO production by BMDMs infected with L. donovani parasites after 72 h treatment with chitosan-coated nanoformulations (UC, TC and MTC) and uncoated AmBisome, AmB. Infected macrophages without treatment served as control. Results are expressed as mean ± SD (n = 3). Data were analysed using a Mann–Whitney test. (B) Effect of treatment with chitosan-coated nanoformulations (UC, TC and MTC) and uncoated AmBisome, AmB solution in BMDMs infected with L. donovani parasites. Infected macrophages without treatment was used as control. Cytokines levels.
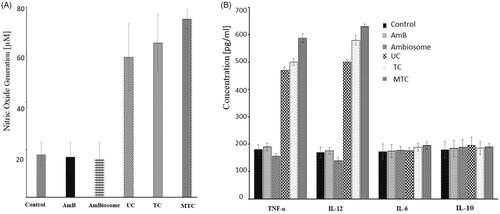
NO production
Currently, efficacy of AmB nanoformulations (UC, TC, MTC and AmBisome) toward the extracellular promastigotes a significant selectivity against the parasites inside the macrophages, and the likely immunomodulatory efficacy of AmB nanoformulations in Leishmania-infected BMDMs were explored. The Leishmania-mediated killing could be an incident related with the up-regulation of NO by BMDMs, trigged by chitosan [Citation21]. So, Griess assay was conducted in order to measure the nitrite, the end product of cellular NO, produced after treatment with chitosan-coated nanoformulations (UC, TC and MTC) and uncoated AmBisome, AmB. Supernatant of BMDMs treated with chitosan-coated nanoformulations (UC, TC and MTC) showed a considerably higher amount of nitrite in comparison to BMDMs treated with uncoated AmBisome and AmB and untreated cells (). In contrast to normal cells (untreated), nitrite production was 9.57-fold (p < .05) and 1.95-fold (p < .05) higher when cells were treated with chitosan-coated nanoformulations and uncoated formulations (AmBisome and AmB).
Immunomodulatory evaluation
Macrophages are the main effector cells responsible for eradication of parasites, which can be triggered by distinctive signals, leading to their expansion into functionally distinctive subsets with diverse disease outcomes [Citation22]. Accordingly, appropriate stimulation of macrophages is essential for eradicating this intracellular pathogen [Citation23]. Cytokines such as IL-6 and IL-10 perform a serious role in regulating macrophage stimulation. Numerous clinical over and above experimental studies demonstrated that both IL-6 and IL-10 are involved in pathogenesis of visceral leishmaniasis [Citation24]. It has also been described that patients with active visceral leishmaniasis have great serum levels of IL-10 and IL-6 and a greater percentage of IL-10 prior to therapy, representing their association with disease persistence. Leishmania parasites escape from the production of deadly microbicidal NO by inhibiting the expression of NO synthase [Citation25]. Therefore, intracellular parasite killing would also be affected by production of intracellular NO. Immunomodulatory studies reveled that chitosan-coated nanoformulations (UC, TC and MTC) stimulated macrophage via generation of Th1-type cytokines (IL-12 and TNF-α) along with downregulation of Th2-type cytokines (IL-6 and IL-10) as shown in . Moreover, treatment with chitosan-coated nanoformulations showed improved production of NO. These perquisites are validating the improved leishmanicidal activity of chitosan-coated nanoformulations in comparison to uncoated AmB and commercial formulations AmBisome. The immunomodulatory response revealed by UC, TC and MTC is attributed to chitosan present on the surface of nanoformulations which is suggested to have macrophage initiation proficiency via upregulation of Th1-type signals and downregulation of Th2 signals. Hence, restoration of Th1-type macrophagic immune signals would support the leishmaniasis therapy.
Permeation-enhancing studies
One of the objectives of the study was to improve the oral bioavailability of the AmB by enhancing the permeation of the AmB-loaded nanocarriers. It is highly recognized that influx and efflux transporters for instance P-glycoprotein (P-gp) available on the intestinal epithelial cells membrane have a considerable influence on drug absorption [Citation26,Citation27]. Besides P-gp, paracellular route is another pathway which can significantly improve the bioavailability of orally administered drugs and drug-loaded nanocarriers. Paracellular route, the microscopic gap presents between adjacent enterocyte, guarded by proteins, which can be reversibly opened via various strategies to increase oral permeation. Thiomers are reported for their potential involvement in opening paracellular route. Therefore, it was essential to evaluate the impact of thiomer-based nanocarriers in improved oral bioavailability of AmB. The effect of verapamil (P-gp inhibitor) on the absorption profile of AmB was also evaluated. Results of permeation studies with AmB on Caco-2 cells are represented in and Papp values with enhancement ratios are summarized in . Our results demonstrated that the presence of P-gp inhibitor, verapamil (100 μg/ml), transport of AmB was slightly increased indicating AmB is a non-substrate for P-gp as compared to the buffer control. These results of Papp indicated that the intestinal absorption of AmB was markedly improved up to four-folds with MTC (p < .05) as compared to pure AmpB. The results suggested that the prime mechanism of permeation improvement by TC and MTC is opening of paracellular route which facilitated the AmB transport along with the inhibition of protein tyrosine phosphatase (PTP) [Citation28]. The opening of tight junction is attributed to the interaction of thiomers with desmosomes present at tight junctions via disulfide linkage. Thus, reversibly opening them for drug transport. The inhibition of PTP can be accomplished by a disulfide bond (S–S) generation with the cysteine site of the protein. Consequently, a higher degree of tyrosine phosphorylation of the membrane protein occludin, contributing to the opening of the tight junctions. Hence, an appreciably improved permeability through tight junctions was examined.
Figure 3. (A) Permeation enhancement of AmB from AmB, AmB in the presence of verapamil, TC and MTC across Caco-2 cell line through ex vivo studies, (B) swelling studies of UC, TC and MTC, (C) plasma concentration of AmB after oral administration of AmB suspension, TC, MTC and Ambisome (oral dose =20 mg/kg). Blood samples were taken at predefined time till 24 h and analyzed through HPLC for AmB quantification, and (D) in vitro hemolysis assay performed on fresh human red blood cells. All the analysis was done in triplicate and results are shown as mean ± SD.
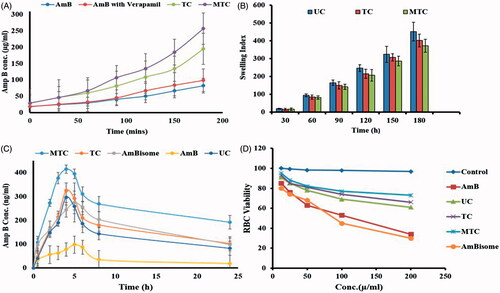
Table 2. Results of viscoelastic parameters, i.e. storage modulus (G′), loss modulus (G′′) and apparent viscosity of nanoformulations UC, TC, and MTC.
Mucoadhesive studies
Mucoadhesion of the nanoparticles is of considerable importance for the oral delivery of the drugs. The mucoadhesive nanoparticles remain attached to the mucus for the longer period of time and provide increased contact time which in turn results in the better absorption of the nanoparticles. Also, increased contact time with the intestinal lining providing a continuous pool of the drug for extended period of time for the better absorption of drugs. The mucoadhesive character of the prepared TC and MTC nanoformulations was assessed by the rheological synergism. The thiol groups are likely to interact with the mucin by the disulfide bond formation, chain interlocking, electrostatic interactions and produce conformational changes. In this regard, the viscoelastic behavior of the completely hydrated UC, TC and MTC and mucin may be considered as the reflection of the mucoadhesive strength. Rheological synergism has been proposed as an in vitro parameter to measure the mucoadhesive properties of polymeric formulations. Higher the rheological synergism, the stronger the interactions with the mucus predicting better gastric absorption of the nanoparticles. The viscoelastic parameters were measured and presented in . For TC and MTC the G′ and G′′-values are higher for the mucin formulation mixture compared to the UC mucin formulations indicating a 10-fold higher storage modulus values within 2 h. This increase is may be due to the interaction of thiol groups with the mucin by the formation of disulfide bonds. The mucin is rich with cysteine subunits having –SH groups. The –SH group of thiomers interacts via a disulfide exchange reaction forming (–S–S–) thus developing a covalent bond with the mucus and strong mucoadhesive strength. The lack of considerable increase in the viscoelastic parameters of the UC is due to the lack of thiol groups in the polymer backbone. These finding clearly demonstrate the advantages of using thiolated polymers for the purpose oral absorption of the nanoparticles.
Table 3. Results showing ex vivo permeation enhancement in terms of concentration transported across the intestine and apparent permeability along with improvement ratios of AmB in the presence of verapamil and synthesized TC and MTC.
Water absorbing capacity
The water absorbing capacity of the nanoparticles significantly influence the mucoadhesion with skin or mucosa, drug release and stability. Once attached to mucus membrane the nanoparticles absorb water via capillary action form the underlying mucus membrane and swell up thus developing the mucoadhesion and initiating drug release. However, the swelling behavior must be in optimal limits to control the release over a sufficient time period. An immediate and abrupt swelling is likely to develop weak adhesion whereas too slow swelling may result in localized mucosa-related issues and retard drug release. Therefore, the swelling of the UC, TC and MTC was performed and the results of the swelling studies are presented in . Thiolated nanoparticle showed a slow and gradual increase in swelling that is desired for the optimal mucoadhesion while the UC exhibited a rapid and excessive swelling character which may negatively affect the mucoadhesion and stability and abrupt drug release behavior. On the other hand, MTC decreased swelling which may be attributed to the presence of surface mannose groups which might resulted in decreased water uptake resulting in low swelling index as compared to TC.
In vitro bioavailability and pharmacokinetic studies
The particles of size 5–150 µm can be taken up the villus tips and intestinal macrophages captures the particles of size 1 µm while the particles of size 300–400 nm can be transported across the enterocytes via transcellular route [Citation29]. Thiolated nanocarriers (TC and MTC) were mainly designed for the enhanced permeation and thus oral bioavailability of AmB. Thiomers can open the tight junction between the enterocytes and thus enhance the uptake of nanoparticles. Furthermore, the macrophage targeting nature of the MTC efficiently targets the intestinal macrophages thus adding to the AmB transport across the enterocytes. All these can enhance the oral uptake of AmB which is otherwise minimally absorbed. The in vivo oral bioavailability was performed on rat and plasma drug concentration, quantified via HPLC was plotted against time interval to achieve the plasma level time graph which is shown in . Plasma drug concentrations of orally administered TC, MTC, AmB and AmBisome are shown and various pharmacokinetic parameters calculated are summarized in . The AUC was significantly increased with MTC as compared to AmB and AmBisome showing the success of nanocarrier in transporting drug into systemic circulation. Designed nanocarriers have the ability to retain the drug inside the macrophages for the longer period of time compared AmBisome and AmB. Ability to retain the drug may be attributed to the P-gp inhibition properties of thiomers that prevented the rapid efflux of the drug. Cmax was improved to 4.2- and 1.6-folds as compared to AmB and AmBisome respectively. Half-life (t1/2) was increased to 3.3- and 2.1-folds as compared to AmB and AmBisome. An increase of 6.4-folds in bioavailability was observed as compared to AmB. Hence, MTC significantly improved oral bioavailability of AmB compared to other formulations.
Table 4. Results of in vivo bioavailability and important pharmacokinetic parameters obtained after oral administration of AmB solution in deionized water, TC and MTC.
In vitro hemolysis assay
In vitro hemolysis assay was performed in order to evaluate the compatibility of TC and MTC against the blood that is one of the prime consideration in the nano-based drug delivery systems. Fresh human blood RBCs were used for this purpose and hemolytic effect was assessed by using different concentrations of AmB, AmBisome TC and MTC. MTC-blank serve as control. The results are presented in the indicated the toxic effects of AmB even at the lowest concentrations while AmBisome showed 45% viability at 20 mg concentration. The toxicity was well-controlled with TC and MTC which showed better compatibility over a large range. MTC control showed least toxicity indicating the safety of carrier.
Tissue distribution analysis
In visceral leishmaniasis, the causative parasite resides inside the macrophages and then these macrophages are entrapped by the liver and spleen. The Leishmania parasite escape the killing mechanism of macrophages owing to its unique redox biology and infects other macrophages thus causing the inflammation of liver and spleen [Citation30]. Our approach was to target the cellular reservoirs that ultimately accumulate in the liver and spleen thus providing the selective targeting of pathological condition providing the increased AmB concentration at the target site. Therefore, we have evaluated the tissue distribution of AmB and found a significantly higher concentration of AmB in liver in case of MTC nanocarriers (101 µg) compared to the free AmB (43 µg). While in case of kidney, the MTC showed less concentration of AmB as compared free AmB. These results () indicate that macrophage targeting was successful in providing the maximum exposure of drug at the desired site while minimizing the exposure to the organs prone to toxic effects.
Figure 4. (A) Organ-to-body ratio of different treatment evaluated on liver, kidneys and heart, (B) tissue distribution calculated in terms of organ drug concentration via HPLC analysis of tissue homogenate, (C) LFTs and (D) RFTs performed of mice serum after 14 days’ acute oral toxicity evaluation.
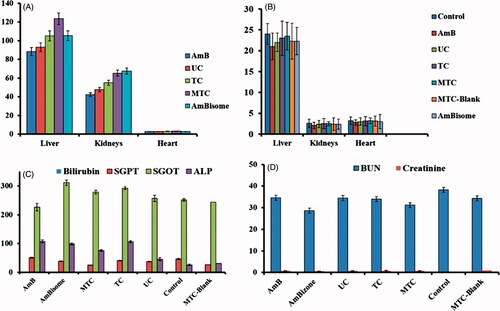
Acute oral toxicity
One of the draw back to available intravenous therapy of AmB (AmBisome) is the chronic nephrotoxicity. The macrophage targeting make it possible for the nanocarriers to selectively target the visceral organs like liver and spleen which is the target site for the visceral leishmaniasis. Thus, the designed nanocarriers minimized the exposure of AmB to the organs likely to be affected by the AmB. The acute oral toxicity induced by TC and MTC was evaluated in female Swiss mice and compared to AmB and MTC Blank in order to establish the safety profile of the nanocarriers and ingredients. A high concentration of AmB, TC and MTC equivalent to 50 mg/kg of AmB were administered orally. The mice did not show any changes in skin tone, behavior and digestion changes during the first 24 h of administration. No mortality was observed during the course of study. After 14 days, the blood was collected in sterilized vials for the biochemical analysis. The mice were then euthanized to collect the different organs for further studies.
Organ-to-body index
The organ-to-body index is an indication of any toxicity induced by the treatment in animal body. Organ–body index was evaluated by carefully removing the vital organs including liver, kidneys and heart from the euthanized mice and washed immediately with normal saline. The organ-to-body ration of different organs were then compared with control. The results in liver weight was slightly decreased in case of AmB showing the toxic effects while for the TC, MTC and MTC-blank, they showed very little effect on liver. The kidneys were also affected with the toxic effects of the Am Bas the organ-to-body ratio of kidneys was decreased compared to the other nanocarriers. No significant effects were observed on the heart due to AmB and prepared various formulations.
Serum biochemistry
Serum biochemistry was carried out to assess the toxic effects of the TC, MTC, MTC blank and AmB on liver and kidneys. Liver and kidneys are most likely to be effected as former is involved in metabolism and later is involved in excretion of drug and its metabolites. The effect of all treatments on liver and kidney was assessed via liver function tests (LFTs) and renal function tests (RFTs). The results of LFTs are shown in that revealed slight changes in the values of LFTs as compared to the control. Cellular integrity of liver is depicted in transaminases (SGPT) which is produced within liver cells [Citation31]. The higher blood level of SGPT indicates cell damage or necrosis leading to leakage of this enzyme in blood. An increased level of SGPT was observed with AmB and AmB-loaded nanocarriers as compared to control, yet remained within the acceptable limits. However, it was lest affected by MTC control. Cellular integrity and its linkage with bile duct is represented by ALP which is characteristic finding of cholestatic liver disease. ALP level was slightly increased with all treatments suggesting some obstruction in bile duct. MTC decreased ALP level as compared to control. Bilirubin level did not change significantly. Liver is the prime source of all serum proteins and any changes in total protein is an indicator of liver abnormality. The total protein content remained unaffected with TC and MTC. The effect of TC and MTC control on LFTs was insignificant (p < .005) as compared to AmB, revealing compatibility of MTC [Citation32,Citation33].
The effect on kidney was assessed through RFTs. The results in showed no significant deviation from reference values of RFTs. Creatinine level remained unaffected with all treatments. However, BUN was increased with MTCs as compared to control which remained within the reference ranges.
Tissue histology
The removed vital organs were macroscopically examined for any visible change in structure of damage. These vital organs are metabolic targets of any toxic sign induced. The issue slides of these organs were carefully prepared through microtome and stained for microscopic evaluation for toxicity evidence. The prepared slides were observed for any change on cellular morphology or pathological changes in cell morphology via (Olympus BX51M, Tokyo, Japan). The liver cells in control were observed to be round and polygonal with clear nucleus as shown in . Slight changes in cellular morphology with appearance of fatty globules were observed in liver slides (, c2–3) treated with formulations. No changes were observed in heart histology as compared to control as shown in , a1–3. The histological examination of kidneys (, b1–3) did not show any evident change in cellular morphology supporting the safety of ENLs due to renal clearance. Similarly, no changes were observed in heart tissues a visible in , a1–3.
Figure 5. Microscopic examination of tissue histology of vital organ (liver, kidney and heart) to examine any necrosis or histological change as compare to control for these organs after treatment with formulations: (a) heart tissue of control; (1a) treated with MTC, (2a) treated with MTC-control; (b) kidney tissue of control, (1b) treated with MTC, (2b) treated with MTC-blank; (c) liver tissue of control, (1c) treated with MTC and (2c) treated with MTC-blank.
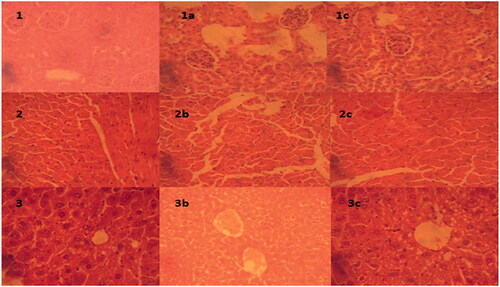
Conclusion
Mannose-anchored thiomer-based AmB nanocarriers were evaluated for their therapeutic potential via parasitic load, macrophage cell membrane integrity, immunomodulator effects, enhanced oral bioavailability, selective organ uptake and improved safety profiles. These MTC showed 6.4-folds improved oral bioavailability of AmB, higher enough to target visceral Leishmania following 3.3-folds increased oral administration as well. These findings also provide evidence for controlled release of drug over an extended period of time marinating the MEC. Moreover, the study showed biocompatibility of formulations with red blood cells as compared to AmB and commercially available AmBisome formulation. The acute oral toxicity evaluation also suggested the biocompatibility of MTC in terms of its effect on LFTs and RFTs supported by the histological evaluation of liver and kidney. Thus, the designed mannose-anchored thiomer-based nanocarriers suggest a potential platform for improved oral bioavailability of AmB through enhanced intestinal permeation along with mannose-targeted macrophage internalization for better treatment of visceral leishmaniasis.
Hafiz_Shoaib_Sarwar_et_al._Supplementary_Material.docx
Download MS Word (16.9 KB)Acknowledgements
The authors have no other relevant affiliations or financial involvement with any organization or entity with a financial interest in or financial conflict with the subject matter or materials discussed in the manuscript apart from those disclosed.
Disclosure statement
No potential conflict of interest was reported by the authors.
Additional information
Funding
References
- Wasan KM, Wasan EK, Gershkovich P, et al. Highly effective oral amphotericin B formulation against murine visceral leishmaniasis. J Infect Dis. 2009;200:357–360.
- Croft SL, Sundar S, Fairlamb AH. Drug resistance in leishmaniasis. Clin Microbiol Rev. 2006;19:111–126.
- Tonomura Y, Yamamoto E, Kondo C, et al. Amphotericin B-induced nephrotoxicity: characterization of blood and urinary biochemistry and renal morphology in mice. Hum Exp Toxicol. 2009;28:293–300.
- Daraee H, Etemadi A, Kouhi M, et al. Application of liposomes in medicine and drug delivery. Artif Cells Nanomed Biotechnol. 2016;44:381–391.
- Amidon GL, Lennernäs H, Shah VP, et al. A theoretical basis for a biopharmaceutic drug classification: the correlation of in vitro drug product dissolution and in vivo bioavailability. Pharmaceut Res. 1995;12:413–420.
- Engwerda CR, Ato M, Kaye PM. Macrophages, pathology and parasite persistence in experimental visceral leishmaniasis. Trends Parasitol. 2004;20:524–530.
- Chaubey P, Mishra B. Mannose-conjugated chitosan nanoparticles loaded with rifampicin for the treatment of visceral leishmaniasis. Carbohydr Polym. 2014;101:1101–1108.
- Bernkop-Schnürch A, Schwarz V, Steininger S. Polymers with thiol groups: a new generation of mucoadhesive polymers? Pharm Res. 1999;16:876–881.
- Bonengel S, Bernkop-Schnürch A. Thiomers-from bench to market. J Control Release. 2014;195:120–129.
- Leitner VM, Walker GF, Bernkop-Schnürch A. Thiolated polymers: evidence for the formation of disulphide bonds with mucus glycoproteins. Eur J Pharm Biopharm. 2003;56:207–214.
- Shahnaz G, Edagwa BJ, McMillan J, et al. Development of mannose-anchored thiolated amphotericin B nanocarriers for treatment of visceral leishmaniasis. Nanomedicine. 2017;12:99–115.
- Sohail MF, Javed I, Hussain SZ, et al. Folate grafted thiolated chitosan enveloped nanoliposomes with enhanced oral bioavailability and anticancer activity of docetaxel. J Mater Chem B. 2016;4:6240–6248.
- Ng LG, Hsu A, Mandell MA, et al. Migratory dermal dendritic cells act as rapid sensors of protozoan parasites. PLoS Pathog. 2008;4:e1000222.
- Weischenfeldt J, Porse B. Bone marrow-derived macrophages (BMM): isolation and applications. Cold Spring Harb Protoc. 2008 [cited 2008 Dec 1]. DOI:https://doi.org/10.1101/pdb.prot5080.
- Terrazas C, Oghumu S, Varikuti S, et al. Uncovering Leishmania-macrophage interplay using imaging flow cytometry. J Immunol Methods. 2015;423:93–98.
- de Costa-Silva TA, Grecco SS, de Sousa FS, et al. Immunomodulatory and antileishmanial activity of phenylpropanoid dimers isolated from Nectandra leucantha. J Nat Prod. 2015;78:653–657.
- Iqbal J, Shahnaz G, Dünnhaupt S, et al. Preactivated thiomers as mucoadhesive polymers for drug delivery. Biomaterials. 2012;33:1528–1535.
- Rahmat D, Müller C, Barthelmes J, et al. Thiolated hydroxyethyl cellulose: design and in vitro evaluation of mucoadhesive and permeation enhancing nanoparticles. Eur J Pharm Biopharm. 2013;83:149–155.
- Rahmat D, Sakloetsakun D, Shahnaz G, et al. HEC-cysteamine conjugates: influence of degree of thiolation on efflux pump inhibitory and permeation enhancing properties. Int J Pharm. 2012;422:40–46.
- Radwan MA, AlQuadeib BT, Šiller L, et al. Oral administration of amphotericin B nanoparticles: antifungal activity, bioavailability and toxicity in rats. Drug Deliv. 2017;24:40–50.
- Wang H, Yu X, Su C, et al. Chitosan nanoparticles triggered the induction of ROS-mediated cytoprotective autophagy in cancer cells. Artif Cells Nanomed Biotechnol. 2018;9:1–9.
- Mosser DM, Edwards JP. Exploring the full spectrum of macrophage activation. Nat Rev Immunol. 2008;8:958–969.
- Mukbel RM, Patten C Jr, Gibson K, et al. Macrophage killing of Leishmania amazonensis amastigotes requires both nitric oxide and superoxide. Am J Trop Med Hyg. 2007;76:669–675.
- Kane MM, Mosser DM. The role of IL-10 in promoting disease progression in leishmaniasis. J Immunol. 2001;166:1141–1147.
- Forget G, Gregory DJ, Whitcombe LA, et al. Role of host protein tyrosine phosphatase SHP-1 in Leishmania donovani-induced inhibition of nitric oxide production. Infect Immun. 2006;74:6272–6279.
- Fang G, Tang B, Chao Y, et al. Cysteine-functionalized nanostructured lipid carriers for oral delivery of docetaxel: a permeability and pharmacokinetic study. Mol Pharm. 2015;12:2384–2395.
- Ahmad N, Alam MA, Ahmad R, et al. Preparation and characterization of surface-modified PLGA-polymeric nanoparticles used to target treatment of intestinal cancer. Artif Cells Nanomed Biotechnol. 2017 [cited 2017 May 14]: [15 p.]. DOI:https://doi.org/10.1080/21691401.2017.1324466.
- Iqbal J, Shahnaz G, Perera G, et al. Thiolated chitosan: development and in vivo evaluation of an oral delivery system for leuprolide. Eur J Pharm Biopharm. 2012;80:95–102.
- Javed I, Hussain SZ, Ullah I, et al. Synthesis, characterization and evaluation of lecithin-based nanocarriers for the enhanced pharmacological and oral pharmacokinetic profile of amphotericin B. J Mater Chem B. 2015;3:8359–8365.
- Sarwar HS, Akhtar S, Sohail MF, et al. Redox biology of Leishmania and macrophage targeted nanoparticles for therapy. Nanomedicine. 2017;12:1713–1725.
- Agbaje E, Adeneye A, Daramola A. Biochemical and toxicological studies of aqueous extract of Syzigium aromaticum (L.) Merr. & Perry (Myrtaceae) in rodents. Afric J Tradit Complement Altern Med. 2009;6:241–254.
- Ozer J, Ratner M, Shaw M, et al. The current state of serum biomarkers of hepatotoxicity. Toxicology. 2008;245:194–205.
- Thapa B, Walia A. Liver function tests and their interpretation. Indian J Pediatr. 2007;74:663–671.