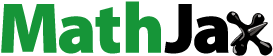
Abstract
This study focuses on the use of ethosome and microwave technologies to facilitate skin penetration and/or deposition of 5-fluorouracil in vitro and in vivo. Low ethanol ethosomes were designed and processed by mechanical dispersion technique and had their size, zeta potential, morphology, drug content and encapsulation efficiency characterized. The skin was pre-treated with microwave at 2450 MHz for 2.5 min with ethosomes applied topically and subjected to in vitro and in vivo skin drug permeation as well as retention evaluation. The drug and/or ethosomes cytotoxicity, uptake and intracellular trafficking by SKMEL-28 melanoma cell culture were evaluated. Pre-treatment of skin by microwave promoted significant drug deposition in skin from ethosomes in vitro while keeping the level of drug permeation unaffected. Similar observations were obtained in vivo with reduced drug permeation into blood. Combination ethosome and microwave technologies enhanced intracellular localization of ethosomes through fluidization of cell membrane lipidic components as well as facilitating endocytosis by means of clathrin, macropinocytosis and in particularly lipid rafts pathways. The synergistic use of microwave and ethosomes opens a new horizon for skin malignant melanoma treatment.
Graphical Abstract

Introduction
Malignant melanoma is the deadliest skin pathology with occurrence at a frequency of 132,000 cases/year globally [Citation1]. Its statistics are on the rise due to repeated and multiple exposures to UV radiations in sunlight. Malignant melanoma has a high mortality rate because it can extend down to dermis and even into systemic circulation if not intervene in time [Citation2]. Medical interventions include surgical removal of the diseased area [Citation3] and topical treatment with chemotherapeutics such as 5-fluorouracil, ingenol mebutate, interferon and imiquimod [Citation4,Citation5]. In combination with imiquimod, 5-fluorouracil exhibits a synergistic therapeutic outcome against the melanoma clinically [Citation6]. 5-Fluorouracil has been used for a long time to treat various skin cancers, but its polar nature limits its effectiveness due to poor penetration into skin layers. The skin penetration and retention capacity of drug from topical formulations such as 5-fluorouracil lotions, creams and/or solutions is challenged by the presence of stratum corneum [Citation7].
Different active and passive drug penetration strategies have been developed and studied to increase skin drug penetration and retention for local treatment but they are often accompanied by high drug permeation into the blood leading to undesired systemic side effects. Vesicular carriers have been studied since a long time ago as they show better penetrability into the skin and can also be accumulated in a high drug quantum in skin layers [Citation8,Citation9]. Ethosomes are reported to be superior in that aspect [Citation10,Citation11]. Various active technologies have been coupled with vesicular carriers to favor enhanced skin drug penetration [Citation12,Citation13]. One major problem encountered with active as well as passive drug penetration strategies is that the transdermal drug flux and permeation into the systemic circulation are high leading to systemic cytotoxic effects and poor patient compliance.
Cell membrane is semi-permeable in nature allowing the passage of small, hydrophilic or unionized drug molecules while hindering permeation of macromolecular drugs and/or carriers into the cell [Citation14]. Macromolecular and polar drugs and/or carriers uptake is mediated by endocytosis which involves a complex coordination of events leading to different endocytic pathways namely clathrin, caveolae and/or lipid rafts, macropinocytosis and receptor-mediated endocytosis [Citation15]. Intracellular drug and/or carrier delivery into cancer cells is crucial for the effective drug response and cancer treatment. On this note, various technologies have been developed and tested including silver and zwitterionic supramolecular prodrug nanoparticles [Citation16,Citation17], cyclodextrin [Citation18], cationic liposomes [Citation19], electrically assisted method [Citation20] and ultrasound [Citation21,Citation22], all of which elicit one or another endocytic pathways for intracellular drug and/or carrier delivery.
Microwaves are electromagnetic waves characterized by frequencies in the range of 300 MHz to 300 GHz which corresponds to wavelength in the range of 1 m to 1 mm [Citation23]. Microwave receives a widespread application in food [Citation24], biomedical [Citation25–28] and pharmaceutical fields [Citation29–32]. It has been exploited recently to facilitate polar drug penetration into skin for transdermal drug delivery system [Citation33]. Through a series of in vitro experiments, microwave is found to increase skin drug and/or ethosome penetration as well as retention via dermal protein fluidization and macromolecular swelling of which reduce the opportunity of drug and/or ethosomes to have deep skin permeation [Citation34].
Local malignant melanoma requires enhanced intracellular drug delivery, maximal skin drug deposition and reduced drug permeation into the blood. Thus far, all skin drug deposition and permeation experiments are conducted in vitro with reference to the use of combination technology of microwave and ethosomes. The present study investigated the in vivo skin 5-fluorouracil deposition and permeation profiles using such combinational technology, and compared against the in vitro findings. The cytotoxicity effects of microwave, drug and/or ethosomes towards SKMEL-28 human melanoma cells, cellular uptake and endocytic pathways of ethosomes, and in vivo pharmacokinetics profiles of drug were examined.
Materials and methods
Materials
Soya phosphotidylcholine (SPC; Sigma Aldrich, St. Louis, MO) and 5-fluorouracil (5-FU; AoBo Bio-Pharmaceutical Technology Co. Ltd., China) were employed as the ethosomal matrix material and drug of interest, respectively. Ethanol 96% (ThermoFisher Scientific, Waltham, MA), sodium chloride, potassium dihydrogen orthophosphate, disodium hydrogen orthophosphate (Merck, Germany) and deionized water were used as the solvent components, with sodium azide (Ajax Finechem, Australia) as the preservative when required. Fluorescein isothiocyanate (FITC; Acros Organic, Morris Plains, NJ) was used to fluorescently tag the ethosomes. Minimum essential medium, fetal bovine serum, penicillin (10,000 U/ml)/streptomycin (10,000 μg/ml) mixture and trypsin–EDTA (ThermoFisher Scientific, Waltham, MA) were used to culture and grow the SKMEL-28 human melanoma cells (ATCC® HTB-72, Manassas, VA). MTT dye 3–(4,5-Dimethylthiazol-2-yl)-2,5-diphenyltetrazolium bromide (Alfa Aesar, Ward Hill, MA) was employed as a reagent for cytotoxicity test.
Preparation of ethosomes
The ethosomes were prepared in adoption of the method of Touitou et al. [Citation35] as described by Khan and Wong [Citation34]. Briefly, 3% of SPC dissolved in ethanol and 0.5% of 5-fluorouracil dissolved in USP phosphate buffer saline pH 7.4 were used as organic and aqueous phases respectively. The fluorescent ethosomes (F-ethosomes) were prepared using the same method by dissolving 0.02 g of FITC along with SPC in ethanol.
Physicochemical characterization of ethosomes
The particle size and zeta potential of ethosomes were determined using photon correlation spectroscopy technique (Malvern Zetasizer Nano ZS 90, Malvern Instruments Ltd., UK) at 25 °C in quartz cell and zeta potential cell with a detect angle of 90° respectively. Nine millilitres of distilled water was added to 1 ml of ethosomes prior to measurement. Three replicates were conducted and results averaged.
The morphology of ethosomes was evaluated by means of scanning electron microscopy (SEM) technique (Quanta FEG 450, FEI, Netherland). A drop of ethosomes was spread over the surfaces of a stub. The sample was dried and coated with platinum at a current intensity of 20 mA for 50 s using an auto fine coater (JFC1600, Jeol, Japan). The sputter-coated sample was examined under SEM at an accelerating voltage of 20 kV. At least three replicates were examined with the representative sections photographed. The morphology of ethosomes was also evaluated using transmission electron microscopy (TEM) technique (Tecnai G2 20 S TWIN, FEI, Netherland). One drop of ethosomes was placed on a carbon-coated copper grid (400 mesh). It was left for 5 min, blot-dried with a filter paper and stained using 2% phosphotungstic acid (EMS, USA). The stained sample was examined by TEM at a current voltage of 200 kV. At least three replicates were examined with the representative sections photographed.
The entrapped drug content in the ethosomal globules, free drug content in the continuous phase of ethosomes and drug encapsulation efficiency of ethosomes were determined by subjecting the sample to centrifugation, and had its drug content of supernatant and sediment characterized by high performance liquid chromatography (HPLC) technique as described by Khan and Wong [Citation34].
Franz diffusion cell (7 ml volume capacity/cell; Hanson MicroettePlus, USA) was used to determine the cumulative drug release profile of ethosomes with Tuffryn membrane (pore size = 0.45 μm; HT-450, Pall Corp., USA) acting as the skin barrier between donor and receptor compartments. The USP buffer pH 5.5 was used as the receptor fluid to simulate skin surfaces condition and its temperature was maintained at 32 ± 2 °C under a continuous magnetic stirring at 400 rpm. An accurately weighed 1 g of ethosomes was loaded into the donor compartment. Two millilitres of aliquots were withdrawn at specified time intervals for 24 h and the cell was replaced by fresh buffer. These aliquots were analyzed using HPLC for drug content released. Three replicates were conducted and the results averaged.
In vitro 5-fluorouracil permeation and retention
Healthy male Sprague Dawley rats (age = 3 months, body weight = 200–250 g/rat; LAFAM, Malaysia) were acclimatized in individual housing for 7 days under 12 h light/dark cycle at 25 ± 2 °C with relative humidity being kept at 65 ± 5 (The deionized water and standard pelletized food (GoldCoin Enterprise, Malaysia) were given ad libitum). The rat skin was harvested by means of the established laboratory protocol with its intactness examined by stereomicroscopy method [Citation33]. All preparative processes were approved by the institutional ethics committee adopting the international guidelines (OECD Environment, Health and Safety) on animal experimentation.
Franz diffusion cell (Hanson Research, Chatsworth, CA) was employed to evaluate the drug permeation (time interval: 0, 0.5, 1, 1.5, 2, 4, 6, 8, 12, 16, 20 and 24 h) and retention (time interval: 24 h) profiles of ethosomes through and in the skin respectively as described by Khan and Wong [Citation34]. At least three replicates were conducted and the results averaged. When required, the microwave was used to modify the physicochemical properties of skin with drug permeation and retention profiles characterized thereafter with reference to the untreated skin. The microwave was applied onto the top surfaces of epidermis using a vector network analyzer system (R&S ZVA, Rohde & Schwarz, Germany), where 1 mW microwave (0.14 mW/cm2) was delivered at the frequency of 2450 MHz for 2.5 min in a non-thermal mode where skin damages were not observable and in the contact mode to avoid electromagnetic losses, following skin equilibration with receptor fluid.
Pharmacokinetics profiling and in vivo skin retention of 5-fluorouracil
Healthy male Sprague Dawley rats (age = 3 months, body weight = 200 ± 20 g/rat; LAFAM, Malaysia) were acclimatized under 12 h light/dark cycle for 7 days in individual housing. They were provided with deionized water and animal feed ad libitum. The ambient temperature was kept at 25 ± 2 °C with relative humidity of 55 ± 2% in automated caging system installed with HEPA filter system. The study protocol was approved by the institutional ethics committee adopting the international guidelines (OECD Environment, Health and Safety) on animal experimentation.
All animals were randomly distributed into two groups (n = 6/group) and labelled as group A and group B. Both groups of animals were first anesthetized by intra-peritoneal ketamine–xylazine injection (ketamine (15 mg) and xylazine (2 mg)/200 g rat) followed by careful shaving of the dorsal region of rats with shaving blade and cleaning by ethanol swab. A plastic cylindrical tube (length: 14 mm, diameter: 10.1 mm and area: 0.80 cm2) was adhered to shaved region with help of cyanomethacrylate adhesive. Group A received 1 g of ethosomes (drug dose = 4.58 mg) filled via the tube nonocclusively, while group B first received the microwave treatment from the direction of epidermis at 2450 MHz for 2.5 min followed by 1 g of ethosomes. Blood samples (200 μl) were withdrawn from tail vein at time intervals of 1 h, 4 h, 8 h, 12 h and 18 h [Citation36]. The 24-h blood sample was collected via cardiac puncture while the animals were still under anesthesia. All blood samples were transferred into heparinized tubes followed by centrifugation (Microcentrifuge Z216MK, Hermle Labortechnik GmbH, Germany) at 5000 rpm for 30 min which were then stored at −20 °C till further analysis. The plasma drug concentration profiles at different time intervals were subjected to pharmacokinetics analysis where maximum plasma concentration (Cmax), time to reach maximum plasma concentration (Tmax), area under the curve from 0 to 24 h (AUC0–24) and from 0 to ∞ (AUC0–∞), as well as terminal elimination rate constant (λz) were calculated [Citation37].
After collecting 24-h blood sample, the rats were subjected to euthanasia by cervical dislocation and the skin was excised from the rats using the protocol previously outlined. The 5-FU content in the skin was determined by HPLC technique. At least hexaplicates were carried out and the results averaged.
HPLC
HPLC technique (Agilent Technologies 1200 series, Agilent Technologies, Germany) was used to determine the 5-fluorouracil content in all samples. The chromatographic separation was performed using Agilent Zorbax SB-C18 reversed-phase column (4.6 × 250 mm system packed with 5 × 10−6 m particles; pore size 8 nm). The column temperature was set at 30 °C. The mobile phase was made of 95% water and 5% methanol. It was filtered through cellulose nitrate membrane (pore size 0.45 µm; Agilent, Germany) before use. The flow rate of mobile phase was 1 ml/min. The volume of sample injection was 10 µL. The detection wavelength of 5-fluorouracil was 265 nm. At least three replicates were conducted for each sample and the results averaged.
For blood sample analysis, a calibration curve was constructed by spiking 100 μl of plasma with 2 μg, 4 μg, 6 μg, 8 μg, 10 μg, 12 μg, 14 μg, 16 μg and 20 μg of 5-fluorouracil pre-dissolved in phosphate buffer saline pH 7.4. The mixture was vortexed for 10 min followed by addition of 300 μl of methanol and further vortexed for 20 min. The mixture was then centrifuged at 5000 rpm for 30 min (Microcentrifuge Z216MK, Hermle Labortechnik GmbH, Germany). The clear supernatant was taken into Eppendorf tubes and dried overnight by heating at 40 °C. The residue was redissolved in 500 µl of mobile phase (methanol:water, 5:95). These samples were vortexed for 10 min and centrifuged at 5000 rpm for 30 min [Citation38]. The clear supernatant layer was subjected to HPLC analysis using the same parameters and conditions as outlined in in vitro 5-fluorouracil analysis. Triplicates were conducted for each sample and the results averaged. The HPLC method of analysis was validated in accordance to ICH guidelines with limit of detection of 10 ng/ml, limit of quantification of 25 ng/ml with precision of 0.80% and accuracy 97.39%, respectively.
In vitro cytotoxicity
Cytotoxic effects of microwave at 2450 MHz for 2.5 min alone and in combination with 5-fluorouracil ethosomes were assessed by MTT assay. Briefly, the resuspended SKMEL-28 human melanoma cells were plated in 96-well culture plate at a cell density of 7500 cells/100 μl/well and incubated at 37 °C/5% CO2 for 24 h to promote their adhesion. On the following day, the culture medium was removed from the wells, followed by the addition of 5-fluorouracil solution (100 μg dose) or ethosomes (21.83 μl of ethosomes containing 100 μg 5-fluorouracil) suspended in 78.17 μl fresh culture medium (total volume 100 μl/well) against a control group receiving no treatment at all. When required, the melanoma cells were pre-treated with microwave at 2450 MHz for 2.5 min in a non-contact mode and the cells were incubated directly or received ethosomes at the above mentioned dosage. All culture plates were incubated for 24 h. Subsequently, the culture medium was removed and 20 μl of MTT reagent solution (5 mg/ml in phosphate buffer saline pH 7.4) was introduced and incubated for another 3.5 h. The live cells were able to reduce the MTT reagent and converted them into needle-like formazan crystals which were transported to the cell surfaces through the process of exocytosis from endosomal/lysosomal compartment. The formed formazan crystals were dissolved by adding 150 μl of dimethylsulphoxide, shaken for 15 min at 250 rpm followed by analysis on UV microplate reader (Gen5 microplate reader, BioTek, Winooski, VT) at the wavelength of 570 nm. The cell viability percentage was calculated using the following equation [Citation39–41]:
where A1 = Absorbance of treated cells; A2 = Absorbance of control cells.
The results were presented as a mean of hexaplicates.
In vitro cellular uptake
The profiles of intracellular trafficking and cellular uptake of ethosomes as a function of microwave treatment of melanoma cells were assessed by confocal laser scanning microscopy technique (TSC SPE, Leica, Japan). Briefly, the washed and trypsinized SKMEL-28 human melanoma cells were transferred into a fluorodish in 1 ml of culture medium at a cell density of 50,000 cells/ml and incubated till 70–80% confluence. The cells were then washed with phosphate buffered saline pH 7.4 and treated by microwave at 2450 MHz for 2.5 min followed by addition of 1 ml of incomplete culture media (without fetal bovine serum) pre-mixed with 100 µl of F-ethosomes (drug dose = 485 μg) and incubation for 3 h at 37 °C/5% CO2. Following incubation, the excess culture medium containing F-ethosomes was removed and the cells were gently washed six times with phosphate buffer saline, followed by confocal laser microscopy analysis to identify the extent of uptake of ethosomes. All samples were viewed with 20 power objective with 12 × zooming. The images were taken in xyz plane (z-stack) with a depth of 5 μm. The microscopic conditions were kept constant throughout samples analysis. Corrected total fluorescent intensities were calculated using Leica application suite (Advanced Fluorescence 2.2.1 build 4842) by subtracting the intensity of background and multiplying with area of cells. The results were presented as a mean of triplicates.
Thermal and spectroscopy analysis of melanoma cells
Melanoma cells treated with microwave and ethosomes were subjected to thermal and vibrational spectroscopic analysis. Briefly, confluent cell cultures were treated with microwave at 2450 MHz for 2.5 min or ethosomes followed by trypsinization and centrifugation at 1200 rpm for 5 min (benchtop centrifuge PK121R, ALC international, UK). The pelleted cells were resuspended in 1 ml of phosphate buffer saline and freeze dried at −50 °C for 3 h (FDU-1200, EYELA, Japan). The dried matter (2 mg) was crimped in a standard aluminium pan and heated from 30 to 100 °C at a heating rate of 10 °C/min under a constant purging of nitrogen at 40 ml/min (Jade DSC, Perkin Elmer, Waltham, MA). The characteristic peak temperature and enthalpy values of endotherm were recorded. At least triplicates were carried out for each sample and the results averaged.
For vibrational spectroscopic analysis, the characteristic peaks of infrared transmission spectra were recorded by FTIR spectrometer (Spectrum 100, Perkin Elmer, Waltham, MA) equipped with MIRacle ATR accessory (PIKE technologies, Fitchburg, WI). The dried matter was placed onto the surface of zinc selenide crystal using pressure clamp to ensure close contact and highest sampling sensitivity. ATR-FTIR spectra were recorded at a resolution of 16 cm−1 over a wavenumber region of 675 to 4000 cm−1 with an acquisition time of 1.5 min. At least three replicates were carried out for each sample and the results averaged.
Endocytosis
Endocytic pathways elicited by microwave for intracellular transport of ethosomes were identified by comparing the endocytosis level of ethosomes in cell culture without and with the addition of specific endocytic inhibitors. Briefly, SKMEL-28 human melanoma cells at a cell density of 50,000 cells/fluoro-dish (with 80% confluence) were pre-incubated with 1 ml of sterile phosphate buffer saline pH 7.4 containing the following inhibitors at concentrations which were not toxic to the cells: 10 µg/ml of chlorpromazine, 200 µM genistein, 50 nM wortmannnin [Citation42] and 25 µg/ml nystatin [Citation43]. After 30 min of incubation, the excess inhibitory solution was removed and 100 µl F-ethosomes (drug dose = 485 μg) pre-mixed with 900 µl of incomplete culture medium (without fetal bovine serum) were introduced and incubated for 3 h. When required, the melanoma cells were pre-treated with microwave at 2450 MHz for 2.5 min in a non-contact mode prior to incubation with endocytic inhibitor solutions and F-ethosomes. After specified time interval, excess F-ethosomes were removed. The melanoma cells were washed gently 6 times with phosphate buffered saline and analyzed using confocal laser scanning microscope for uptake of F-ethosomes by cells.
Statistical analysis
Results were expressed as a mean of at least three experiments with the corresponding standard deviation. Statistical data analysis was carried out using SPSS software version 18.0 and a statistically significant difference was denoted by p < .05. Student’s t-test and analysis of variance (ANOVA)/post hoc analysis by Tukey HSD test were employed when applicable.
Results and discussion
Unilamellar ethosomes with square to near spherical geometry were produced (). They were characterized by homogenous sizes of 128.9 ± 0.4 nm and narrow polydispersibility index of 0.07 ± 0.01 (). The surface charge of the ethosomes was negative with an average value of −7.6 ± 0.6 mV. 5-Fluorouracil is a polar drug with pKa value of 8, and tends to ionize into an anionic form at pH 7.4. The SPC bears permanent negative charge on phosphate moiety and positive charge on tertiary nitrogen. The tertiary amine moiety of SPC could be clustered by PO43−/OH− rich medium which gave rise to overall negative surface charges on the ethosomes. The ethosomal core contained a drug content amounting to 0.133 ± 0.001% whereas the continuous phase had a drug content of 0.335 ± 0.001%. The drug encapsulation efficiency of the ethosomes was 91.6 ± 0.15%. The ethosomes released 78.01 ± 4.65% of drug within 24 h of dissolution ().
Figure 1. Profiles of (a) drug release from ethosomes, (b) drug permeation and (c) drug retention of ethosomes using ▲untreated skin and • skin pre-treated by microwave at 2450 MHz for 2.5 min with (d) scanning electron microscopic image, (e) transmission electron microscopic image and (f) vesicular size distribution of ethosomes. Fractional data were obtained from www.tandfonline.com [Citation34].
![Figure 1. Profiles of (a) drug release from ethosomes, (b) drug permeation and (c) drug retention of ethosomes using ▲untreated skin and • skin pre-treated by microwave at 2450 MHz for 2.5 min with (d) scanning electron microscopic image, (e) transmission electron microscopic image and (f) vesicular size distribution of ethosomes. Fractional data were obtained from www.tandfonline.com [Citation34].](/cms/asset/bcf3ee2d-bff2-490f-856f-c05405b978c6/ianb_a_1431650_f0001_b.jpg)
Skin pre-treated with microwave at 2450 MHz for 2.5 min brought about minimal rise in the permeation flux of drug (; ANOVA, p > .05). The skin retention of the drug determined at the end of 24 h of permeation test was significantly higher (395.4 ± 7.9 μg) compared to the case when ethosomes were used alone (281.3 ± 13.9 μg) (; student’s t-test, p < .05). Microwave increased the drug/ethosomes penetration into the skin by fluidizing the polar and non-polar skin components, and compromising the barrier properties of the stratum corneum [Citation33]. It fluidized specific extracellular protein in the dermal regime which resulted in the formation of permeation barrier through narrowing of the intercellular pathways [Citation34]. The summative effects promoted skin drug/ethosomes retention [Citation34]. The levels of drug permeation did not differ markedly between cases of using untreated and microwave-treated skins. In addition to the formation of dermal barrier under the influence of microwave, the negatively charged drug/ethosomes could have experienced repulsion from the anionic lipids of deeper skin layers thereby deterring their permeation transdermally.
Pharmacokinetics profiling and in vivo skin retention of 5-fluorouracil
The in vivo bioavailability of 5-fluorouracil from ethosomes as a function of skin pre-treatment with microwave was assessed using rat models. Unlike in vitro drug permeation profiles, the in vivo permeation of drug from ethosomes was significantly reduced with rats having their skin treated with microwave (; ANOVA, p < .05). This was denoted by reduced Cmax, AUC0–24 and AUC0–∞ of the drug formulated as ethosomes and administered onto the microwave-treated skin (). Such observations could be due to the difference in physiology of living and dead skin tissue. In the living skin, the tight junctions play a pivotal role in forming and maintaining the epithelial and endothelial barriers. Tight junctions are intercellular contacts that seal the space between the cellular barrier [Citation44]. The microwave was inclined to interact with the polar moieties of a skin constituent. The tight junction was made of a variety of extracellular proteins including claudins, occludin, tricellulin and zonula occludin [Citation45]. The interaction between microwave and tight junctions of the skin was envisaged to bring about the narrowing of paracellular pathway for drug diffusion. On the note that the microwave could penetrate the skin in a volumetric manner and had a relatively long wave length, the narrowing of cellular barrier at the endothelium level could possibly reduce the drug availability in the systemic circulation, while keeping the drug retained in the skin instead (). The reduction in systemic drug absorption and an increase in skin drug retention from ethosomes as a function of skin pre-treatment with microwave were deemed to be favorable when local skin malignant melanoma was concerned.
Figure 2. In vivo profiles of a. drug permeation, b. drug retention and c. 5-FU pharmacokinetics parameters of ethosomes via ▲ untreated skin and • skin pre-treated by microwave at 2450 MHz for 2.5 min. λz = terminal elimination rate constant, t1/2 = drug half-life, Cmax = peak plasma drug concentration, Tmax = time to reach peak plasma drug concentration, AUC0–24 = area under the plasma drug concentration-time plot from 0 to 24 h, AUC0–∞ = area under the plasma drug concentration-time plot from time 0 to infinity.
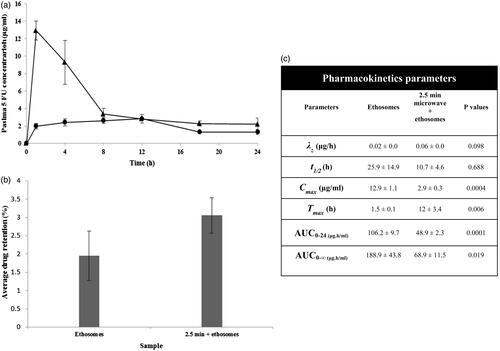
Cytotoxicity and cellular uptake studies
The anti-cancer property of a therapeutic agent was dependent on its cytotoxicity attribute. In the present study, the in vitro cytotoxicity profiles of microwave or ethosomes alone and their combination were assessed by MTT assay. The cytotoxicity profiles of SKMEL-28 human melanoma cells pre-treated with microwave at 2450 MHz for 2.5 min with and without ethosomes application were shown in . The microwave pre-treatment exerted significant cytotoxic effects alone as well as in combination with 5-FU-loaded ethosomes (student’s t-test, p < .05) against the neat 5-FU solution applied at the same drug dosage. The use of drug-loaded ethosomes alone promoted cytotoxicity of 5-FU, possibly due to enhanced drug permeation into the melanoma cells. The pre-treatment of the melanoma cells with microwave directly killed the melanoma cells and could have enhanced cell membrane fluidity which was translated to a further rise in the permeation of ethosomes/drug into the cells. The ultimate fate derived from the use of combination microwave and ethosome technologies was enhanced cellular cytotoxicity. Such approach could benefit the cancer chemotherapy where microwave can act as a radiation anti-cancer agent while promoting ethosomes/drug penetration into the skin and melanoma cells where the target tumors resided.
Figure 3. Cytotoxicity profiles of SKMEL-28 melanoma cells as a function of drug, ethosomes and pre-treatment of cells with microwave at 2450 MHz for 2.5 min.
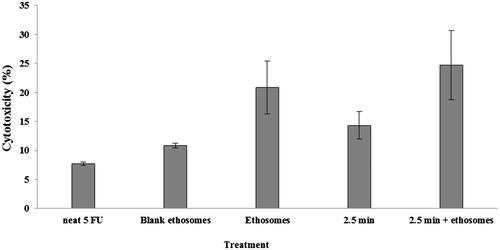
The intracellular drug delivery is crucial for effective manifestation of the anti-cancer activity of 5-FU. Through incubating the melanoma cells with FITC-entrapped ethosomes (F-ethosomes) and analzyed by means of confocal laser microscopy technique, it was found that the ethosomes were intracellularly entrapped in the melanoma cells (). In contrast to cases of using ethosomes alone, pre-treatment of melanoma cells with microwave increased F-ethosomes accumulation in the cells (), inferring by a higher intensity of fluorescence in cells as a result of facilitated intracellular delivery of ethosomes (). The microwave could possibly fluidize the cell membrane to enable permeation of ethosomes into the intracellular compartment of the melanoma cells. Thermal analysis showed that microwave pre-treatment of melanoma cells which received ethosomes significantly reduced the cell line enthalpy associated with lipid domain to 1.2 ± 1.0 J/g (student’s t-test, p < .05) which was found to be 6.4 ± 1.2 J/g in the case of untreated cells (). The cell membrane contains various components namely carbohydrates, lipids and proteins with the primary structural backbone being lipids and phospholipids. The types of phospholipids found in the cell membrane are phosphatidylcholine, phosphatidylethanolamine, phosphatidylserine, phosphatidylinositol, phosphatidic acid and cholesterol. They are responsible to impart compactness to the cell membrane [Citation46,Citation47]. The pre-treatment of cells by microwave at 2450 MHz for 2.5 min appeared to reduce the compactness of the phospholipidic bilayer of cell membrane by fluidizing membrane lipidic components which then enhanced the intracellular ethosomal accumulation. Such observation was further supported by molecular vibrational analysis where a significant increase in the FTIR wavenumber of peaks ascribing symmetric phosphate moiety (PO2-; 1055–1075 cm−1) of membrane was noted when the combination of microwave and ethosomal treatment was adopted ().
Figure 4. Confocal laser micrographs of melanoma cells treated with (a) F-ethosomes, (b) microwave 2450 MHz for 2.5 min + F-ethosomes, and their (c, d) and (e) corrected total cell fluorescence intensities. i = without and with inhibitors applied where ii = chlorpromazine, iii = nystatin, iv = genistein and v = wortmannin.
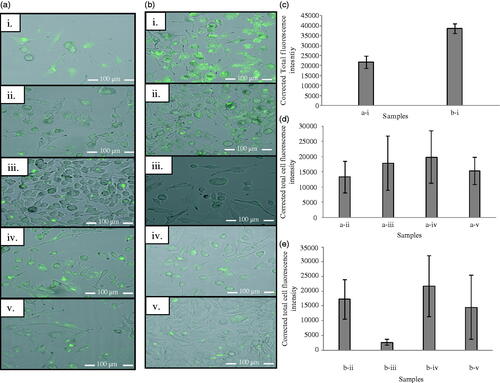
Figure 5. (a) DSC thermogram (T= °C, ΔH = J/g) and (b) ATR-FTIR spectra of freeze dried i. untreated melanoma cells and cells treated with ii. ethosomes, iii. microwave 2450 MHz for 2.5 min and iv. microwave 2450 MHz for 2.5 min + ethosomes.
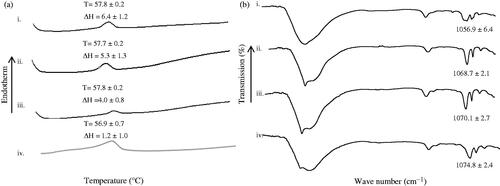
Chlorpromazine is a cationic amphiphilic endocytosis inhibitor which acts by inhibiting the clathrin coated pit formation by reversible translocation of clathrin and its adaptor proteins from the plasma membrane to intracellular vesicles [Citation48]. Genistein is a tyrosin kinase inhibitor which inhibits the formation of cavealoe formation while nystatin acts on lipid rafts-mediated endoytosis via sequestration of cholesterol in the plasma membrane of the cell [Citation15]. Macropinocytosis is an actin-dependent endocytosis which is initiated from cell surface membrane ruffles. Wortmannin is a potent inhibitor of phosphotidyl inositol 3-kinase which is necessary for completion of membrane ruffles thus inhibiting the non-selective uptake of solute molecules, nutrients and antigens via macropinocytosis [Citation49]. Inferring from intracellular F-ethosomes trafficking studies using such inhibitors, it was found that the ethosomes intracellular delivery was primarily promoted through clathrin-mediated endocytic pathway with minor involvements of lipid raft and macropinocytosis (). The clathrin-mediated endocytosis is initiated by strong binding of a ligand to a specific cell surface receptor. This results in the clustering of the ligand–receptor complexes in coated pits on the plasma membrane which is formed by the assembly of cytosolic coat proteins, the main assembly unit being clathrin. The coated pits are then invaginated and pinched off from the plasma membrane to form the intracellular clathrin-coated vesicles with size in the range of 100–150 nm [Citation15]. The size limit of particles endocytosed via the clathrin-dependent pathway as such is typically less than 200 nm [Citation50]. With reference to the present study, the size of the F-ethosomes was 133.5 ± 1.9 nm. This could aptly explain that the endocytosis of ethosomes was primarily elicited via the clathrin-dependent endocytic pathway with lesser involvements of macropinocytosis and lipid raft routes.
In contrast, pre-treatment of melanoma cells by microwave increased the intracellular localization of ethosomes via a combination of receptor-mediated pathways () with particular involvement of lipid raft-mediated endocytosis where a significant reduction in fluorescence intensity up to 93.2% with nystatin application was observed in addition to minor involvement of macropinocytosis and clathrin-mediated endocytosis. Lipid rafts are cholesterol and sphingolipids enriched microdomains found in cell membrane. Since the microwave was able to fluidize the cell membrane lipidic domains (), an increase in cholesterol mobility might occur and the endocytosis was promoted via increased lipid rafts formation.
Conclusions
Skin pre-treatment with microwave at 2450 MHz for 2.5 min increased the 5-fluorouracil retention in skin from ethosomes in vitro and in vivo. The transdermal permeation flux and total drug absorbed into the systemic circulation were however significantly reduced compared to sole ethosomal application in vivo. Pre-treatment of SKMEL-28 human melanoma cells with microwave at 2450 MHz for 2.5 min alone as well as in combination with ethosomes exerted a significant cytotoxic effect against the case of cells receiving the neat drug. Microwave pre-treatment fluidized the lipidic cell membrane component of melanoma cells which increased the intracellular delivery and/or accumulation of drug/ethosomes primarily through lipid rafts-mediated endocytosis with minor involvement of clathrin-dependent pathway and macropinocytosis. The microwave, coupling with 5-fluorouracil ethosomes, is envisaged to be a new horizon for malignant melanoma treatment in future.
Disclosure statement
No potential conflict of interest was reported by authors.
Additional information
Funding
References
- Moschetta M, Telegrafo M, Lucarelli NM, et al. Metastatic breast disease from cutaneous malignant melanoma. Int J Surg Case Rep. 2014;5:34–36.
- Narayanan Dl, Saladi RN, Fox JL. Ultraviolet radiation and skin cancer. Int J Dermatol. 2010;49:978–986.
- Neville JA, Welch E, Leffell DJ. Management of nonmelanoma skin cancer in 2007. Nat Clin Pract Oncol. 2007;4:462–469.
- Haque T, Rahman KM, Thurston DE, et al. Topical therapies for skin cancer and actinic keratosis. Eur J Pharm Sci. 2015;77:279–289.
- Bahner JD, Bordeaux JS. Non-melanoma skin cancers: photodynamic therapy, cryotherapy, 5-fluorouracil, imiquimod, diclofenac, or what? Facts and controversies. Clin Dermatol. 2013;31:792–798.
- Florin V, Desmedt E, Vercambre-Darras S, et al. Topical treatment of cutaneous metastases of malignant melanoma using combined imiquimod and 5-fluorouracil. Investig New Drugs. 2012;30:1641–1645.
- Menon GK, Cleary GW, Lane ME. The structure and function of the stratum corneum. Int J Pharm. 2012;435:3–9.
- Khan NR, Harun MS, Nawaz A, et al. Nanocarriers and their actions to improve skin permeability and transdermal drug delivery. Curr Pharm Des. 2015;21:2848–2868.
- Hussain A, Samad A, Ramzan M, et al. Elastic liposome-based gel for topical delivery of 5-fluorouracil: in vitro and in vivo investigation. Drug Deliv. 2014;14:1–15.
- Ahad A, Raish M, Al-Mohizea AM, et al. Enhanced anti-inflammatory activity of carbopol loaded meloxicam nanoethosomes gel. Int J Biol Macromol. 2014;67:99–104.
- Shen LN, Zhang YT, Wang Q, et al. Enhanced in vitro and in vivo skin deposition of apigenin delivered using ethosomes. Int J Pharm. 2014;460:280–288.
- Chen G, Hao B, Ju D, et al. Pharmacokinetic and pharmacodynamic study of triptolide-loaded liposome hydrogel patch under microneedles on rats with collagen-induced arthritis. Acta Pharm Sin B. 2015;5:569–576.
- Kasetvatin C, Rujivipat S, Tiyaboonchai W. Combination of elastic liposomes and low frequency ultrasound for skin permeation enhancement of hyaluronic acid. Colloids Surf B Biointerfaces. 2015;135:458–464.
- Au JL, Yeung BZ, Wientjes MG, et al. Delivery of cancer therapeutics to extracellular and intracellular targets: determinants, barriers, challenges and opportunities. Adv Drug Deliv Rev. 2016;97:280–301.
- Khalil IA, Kogure K, Akita H, et al. Uptake pathways and subsequent intracellular trafficking in nonviral gene delivery. Pharmacol Rev. 2006;58:32–45.
- Chen Y, Wang Y, Wang H, et al. Zwitterionic supramolecular prodrug nanoparticles based on host-guest interactions for intracellular drug delivery. Polymer. 2016;97:449–455.
- Sun X, Shi J, Zou X, et al. Silver nanoparticles interact with the cell membrane and increase endothelial permeability by promoting VE-cadherin internalization. J Hazard Mater. 2016;317:570–578.
- Réti-Nagy K, Malanga M, Fenyvesi É, et al. Endocytosis of fluorescent cyclodextrins by intestinal Caco-2 cells and its role in paclitaxel drug delivery. Int J Pharm. 2015;496:509–517.
- Li Y, Gao L, Tan X, et al. Lipid rafts-mediated endocytosis and physiology-based cell membrane traffic models of doxorubicin liposomes. Biochim Biophys Acta Biomembr. 2016;1858:1801–1811.
- Sweeney DC, Reberšek M, Dermol J, et al. Quantification of cell membrane permeability induced by monopolar and high frequency bipolar bursts of electrical pulses. Biochim Biophys Acta Biomembr. 2016;1858:2689–2698.
- Wu X, Giridhar BA, Kim BL, et al. Ultrasound-mediated intracellular delivery of fluorescent dyes and DNA into microalgal cells. Algal Res. 2016;15:210–216.
- Tardoski S, Gineyts E, Ngo J, et al. Low-intensity ultrasound promotes clathrin-dependent endocytosis for drug penetration into tumor cells. Ultrasound Med Biol. 2015;41:2740–2754.
- Moghimi HR, Alinaghi A, Erfan M. Investigating the potential of non-thermal microwave as a novel skin penetration enhancement method. Int J Pharm. 2010;401:47–50.
- Goksu EI, Sumnu G, Esin A. Effect of microwave on fluidized bed drying of macaroni beads. J Food Eng. 2005;66:463–468.
- Ramani VS, Sun Myint A, Montazeri A, et al. Preoperative chemoradiotherapy for rectal cancer: a comparison between intravenous 5-fluorouracil and oral capecitabine. Color Dis. 2010;12:37–46.
- Giombini A, Giovannini V, Di Cesare A, et al. Hyperthermia induced by microwave diathermy in the management of muscle and tendon injuries. Br Med Bull. 2007;83:379–396.
- Korpan NN, Saradeth T. Clinical effects of continuous microwave for postoperative septic wound treatment: a double-blind controlled trial. Am J Surg. 1995;170:271–276.
- Jacob C. Treatment of hyperhidrosis with microwave technology. Semin Cutan Med Surg. 2013;32:2–8.
- Wong TW, Wahab S, Anthony Y. Drug release responses of zinc ion crosslinked poly(methyl vinyl ether-co-maleic acid) matrix towards microwave. Int J Pharm. 2008;357:154–163.
- Qasem RJ. The effect of microwave thermal denaturation on release properties of bovine serum albumin and gluten matrices. AAPS PharmSciTech. 2006;7:E1–E7.
- Anuar NK, Wui WT, Ghodgaonkar DK, et al. Characterization of hydroxypropylmethylcellulose films using microwave non-destructive testing technique. J Pharm Biomed Anal. 2007;43:549–557.
- Rani UG, Konreddy AK, Mishra S, et al. Synthesis and applications of polyacrylamide grafted agar as a matrix for controlled drug release of 5-ASA. Int J Biol Macromol. 2014;65:375–382.
- Wong TW, Anuar NK. Physicochemical modulation of skin barrier by microwave for transdermal drug delivery. Pharm Res. 2013;30:90–103.
- Khan NR, Wong TW. Microwave-aided skin drug penetration and retention of 5-fluorouracil-loaded ethosomes. Expert Opin Drug Deliv. 2016;13:1209–1219.
- Touitou E, Dayan N, Bergelson L, et al. Ethosomes – novel vesicular carriers for enhanced delivery: characterization and skin penetration properties. J Control Release. 2000;65:403–418.
- Qiao J, Gu C, Shang W, et al. Effect of green tea on pharmacokinetics of 5-fluorouracil in rats and pharmacodynamics in human cell lines in vitro. Food Chem Toxicol. 2011;49:1410–1415.
- Zhang Y, Huo M, Zhou J, et al. PKSolver: an add-in program for pharmacokinetic and pharmacodynamic data analysis in Microsoft Excel. Comput Methods Programs Biomed. 2010;99:306–314.
- Bose A, Elyagoby A, Wong TW. Oral 5-fluorouracil colon-specific delivery through in vivo pellet coating for colon cancer and aberrant crypt foci treatment. Int J Pharm. 2014; 468:178–186.
- Lee JY, Kim JS, Cho H, et al. Poly(styrene)-b-poly(DL-lactide) copolymer-based nanoparticles for anticancer drug delivery. Int J Nanomed. 2014;9:2803–2813.
- Paolino D, Celia C, Trapasso E, et al. Paclitaxel-loaded ethosomes®: potential treatment of squamous cell carcinoma, a malignant transformation of actinic keratoses. Eur J Pharm Biopharm. 2012;81:102–112.
- Paolino D, Cosco D, Muzzalupo R, et al. Innovative bola-surfactant niosomes as topical delivery systems of 5-fluorouracil for the treatment of skin cancer. Int J Pharm. 2008;353:233–242.
- Peng SF, Tseng MT, Ho YC, et al. Mechanisms of cellular uptake and intracellular trafficking with chitosan/DNA/poly(γ-glutamic acid) complexes as a gene delivery vector. Biomaterials. 2011;32:239–248.
- Manunta M, Tan PH, Sagoo P, et al. Gene delivery by dendrimers operates via a cholesterol dependent pathway. Nucleic Acids Res. 2004;32:2730.
- Sapra B, Jindal M, Tiwary AK. Tight junctions in skin: new perspectives. Ther Deliv. 2012;3:1297–1327.
- Morita K, Miyachi Y. Tight junctions in the skin. J Dermatol Sci. 2003;31:81–89.
- van Meer G, Voelker D, Feigenson G. Membrane lipids: where they are and how they behave. Nat Rev Mol Cell Biol. 2008;9:112–124.
- Ohline SM, Campbell ML, Turnbull MT, et al. Differential scanning calorimetric study of bilayer membrane phase transitions. J Chem Educ. 2001;78:1251–1256.
- Wang LH, Rothberg KG, Anderson RGW. Mis-assembly of clathrin lattices on endosomes reveals a regulatory switch for coated pit formation. J Cell Biol. 1993;123:1107–1117.
- Wymann MP, Bulgarelli-Leva G, Zvelebil MJ, et al. Wortmannin inactivates phosphoinositide 3-kinase by covalent modification of Lys-802, a residue involved in the phosphate transfer reaction. Mol Cell Biol. 1996;16:1722–1733.
- Rejman J, Oberle V, Zuhorn IS, et al. Size-dependent internalization of particles via the pathways of clathrin- and caveolae-mediated endocytosis. Biochem J. 2004;377:159–169.