Abstract
Transplantation of islet is a promising method in treatment of patients with type 1 diabetes mellitus (T1DM), however, is limited by islet shortage. The aim of this study was to prepare a polyethersulfone (PES) nanofibrous scaffolds to evaluate the pancreatic differentiation of human induced pluripotent stem cells (hiPSCs). The differentiation process in tissue culture dishes and PES scaffolds was evaluated at mRNA and protein level by RT-qPCR and immunofluorescence assay, respectively. The functionality of differentiated cells was determined by insulin and C-peptide release in response to glucose challenges. The results of this study showed that cells cultured on PES nanofibrous scaffolds exhibit more pancreatic β-cell characteristics as they express more pancreatic tissue-specific genes and proteins. Furthermore, the immunoassay showed that differentiated cells in both culture plates and PES scaffolds groups are functional and secrete C-peptide and insulin in response to glucose challenges. Altogether, the results of this study demonstrated that PES nanofibrous scaffold could provide the microenvironment that promotes the differentiation of induced pluripotent stem cells (iPSCs) into insulin producing cells.
Introduction
Diabetes mellitus is a metabolic disorder with elevated blood glucose level. Despite recent progress in diabetes therapy, the prevalence of diabetes is increasing according to the World Health Organization (WHO) and American Diabetes Association (ADA) [Citation1,Citation2]. Type 1 diabetes mellitus (T1DM) is known as an autoimmune disease in which pancreatic β cells responsible for producing insulin were destroyed by immune cells. Decreasing blood glucose level by injection of exogenous insulin is the most common treatment of patients with T1DM. However, this therapy does not cure many complications related to this disease such as dyslipidaemia, kidney failure and blindness which may lead to amputation in advanced cases [Citation3].
Pancreatic islet transplantation is an alternative therapy used over the past two decades, however, this strategy is limited due to the shortage of islets cells donor [Citation4]. Recently, many studies have focused to use different sources of stem cells to produce pancreatic beta cells [Citation5]. Due to high potential self-renewability and differentiation capacity, induced pluripotent stem cells (iPSCs) are considered as an appropriate cell source to be used in alternative therapies. These cells are capable to differentiate into insulin-producing cells (IPCs) either by gene transfer or culture in differentiation medium [Citation6–9]. However, these strategies are facing low differentiation efficiency when cells are cultured on tissue culture dishes. The culture of cells on biocompatible and biodegradable polymers provides cell–cell and cell–matrix interactions as those exist in native tissues. These interactions affect signalling of cells and consequently mediate cell survival and differentiation [Citation10]. Several studies have shown the culture of cells on nanofibrous scaffolds could enhance the efficiency of making IPCs [Citation11,Citation12]. The aim of this study was to generate IPCs on electrospun polyethersulfone (PES) nanofibers and to compare the effect of this polymer in the differentiation of cells into IPCs.
Materials and methods
Stem cell expansion
Human iPSC line was expanded and characterized in Stem Cells Technology Research Center as previously described [Citation9]. These cells were cultured on mouse embryonic fibroblast (MEF) feeder layer inactivated with mitomycin-C (Sigma, St. Louis, MO) in DMEM/F12 supplemented with 20% knockout serum replacement, 0.1 mmol/L nonessential amino acids (Invitrogen, Carlsbad, CA), 2 mM l-glutamine (Sigma, St. Louis, MO), 0.1 mM β-mercaptoethanol (Merck, Kenilworth, NJ) and 10 ng/mL of recombinant human basic fibroblast growth factor bFGF (Invitrogen, Carlsbad, CA) and incubated at 37 °C in 5% CO2 and 95% humidity. The cells were passaged every four days for expansion.
Scaffold fabrication and surface modification
Nanofibrous PES scaffolds were fabricated by electrospinning according to a protocol modified with Shabani et al. [Citation13]. To optimize the hydrophilicity, surface plasma treatment of PES scaffolds was performed under 40 kHz low frequency at 30 W conditions with a cylindrical quartz reactor (Diener Electronics, Ebhausen, Germany) by introducing pure oxygen into the reaction chamber at 0.4 mbar pressure and then the glow discharge was applied for 5 min.
Scanning electron microscopy
Cell-seeded and unseeded scaffolds were fixed in 2.5% paraformaldehyde and subsequently dehydrated in a series of ethanol (50%, 60%, 70%, 80%, 95% and 100% v/v, respectively). The dried scaffolds are mounted onto aluminium sample holders and coated with palladium–gold in a sputter-coater (Hitachi, Tokyo, Japan). Then, the samples were observed under a scanning electron microscope (SEM; Hitachi S-4500, Tokyo, Japan) at an accelerating voltage of 5 kV.
Differentiation into insulin-producing cells
A three-step differentiation protocol was used to induce human induced pluripotent stem cells (hiPSCs) into IPCs. In step 1, the hiPSCs were cultured (37 °C, 5% CO2) in the medium I containing serum-free high glucose DMEM (25 mmol/L) containing 0.5 mmol/L beta-mercaptoethanol and 50 ng/mL activin A (Sigma, St. Louis, MO) for seven days. In step 2, the hiPSCs were treated by the differentiation medium II containing 1% nonessential amino acids (Invitrogen, Carlsbad, CA), 20 ng/mL epidermal growth factor (EGF; Sigma, St. Louis, MO), 20 ng/mL basic fibroblast growth factor, 2 mmol/L l-glutamine, 2% B27 (Invitrogen, Carlsbad, CA) and 10 ng/mL exendin-4 (Sigma, St. Louis, MO) for seven days. Finally, in step 3, the hiPSCs were cultured for an additional seven days in differentiation medium III containing 10 ng/mL betacellulin (Sigma, St. Louis, MO), 2% B27, 10 mmol/L nicotinamide (Sigma, St. Louis, MO) and 10 ng/mL exendin-4 [Citation9]. During differentiation process, the medium was changed every two days.
Cell viability assay
The viability of iPSCs on PES nanofibrous scaffold compared to tissue culture plate was assessed via MTT assay for seven days. Briefly, sterilized nanofibers were placed in a 48-well cell culture plate, seeded at a cell density of 4 × 103 cells/cm2 in differentiation medium and incubated at 37 °C, 5% CO2. At days 1, 3, 5 and 7 after seeding hiPSCs, 50 µL MTT solution (3-[4,5-dimethyl-thiazolyl-2]-2,5-diphenyltetrazolium bromide) with a final concentration of 5 mg/mL in DMEM was added to each well. After 3 h incubation, the supernatant was discarded and the formazan crystals were solubilized with dimethylsulphoxide (DMSO). Plates were shaken for 10 min to dissolve the precipitate. The absorbance of the samples at 570 nm was measured using a BioTek Synergy HT microplate reader (BioTek Instruments Inc., Winooski, VT). Mean values of three repeats in tissue culture plates and PES scaffolds groups were used for further analysis.
Gene expression analysis
Total RNA was isolated with the RNeasy Mini Kit (Qiagen, Hilden, Germany) following the manufacturer’s protocol. Then, RNA was reverse transcribed by the random hexamer primer using a M-MuLV Reverse Transcriptase kit (Fermentas) at 42 °C for 60 min and 70 °C for 5 min in a total reaction volume of 15 μL. qPCR was performed using a SYBR premix ExTaq (Takara, Seoul, Korea) according to the manufacturer’s protocol in an ABI StepOne system with a total reaction volume of 10 μL. The primer sequences are illustrated in . All reactions were performed at 95 °C for 2 min, followed by 40 amplification cycles in triplicate, and the data were normalized to the Ct value of the reference gene β2M. The relative quantification (2−ΔΔCt) method was applied to analyse the data.
Table 1. Primer sequences used for real time-PCR in this study.
Immunofluorescence assay
To evaluate pancreatic differentiation, the differentiated cells in both tissue culture plates and PES scaffolds groups were fixed with 4% (w/v) paraformaldehyde (Sigma, St. Louis, MO) and incubated in PBS at 4 °C for 30 min. All samples were permeabilized with 0.2% Triton X-100 (Sigma, St. Louis, MO) for 10 min and blocked with 10% goat serum (Sigma, St. Louis, MO) for 1 h at RT. Samples were incubated overnight with anti-Pdx1 (1:2000; #ab47267) and anti-insulin (1:200; #ab181547) primary antibodies at 4 °C. Then, samples were rinsed two times with PBS and subsequently incubated with fluorescein isothiocyanate-labelled secondary antibodies for 90 min at RT in darkness. After washing with PBS, the nuclei were stained with 0.1 μg/mL DAPI (Sigma, St. Louis, MO) for 5 min. Images were captured under a phase contrast fluorescent microscope (Nikon, Melville, NY).
Insulin and C-peptide release assay
The differentiated cells in both tissue culture plates and PES scaffolds groups were washed with PBS and pre-incubated in freshly prepared glucose-free Krebs–Ringer bicarbonate (KRB) buffer containing 25 mM NaHCO3, 5 mM KCl, 2.5 mM CaCl2, 1.1 mM MgCl2, 120 mM NaCl and 0.1% BSA (all from Sigma, St. Louis, MO) for 2 h. Then, differentiated cells were incubated for 1 h in KRB buffer with different concentrations of glucose. The supernatant was collected and stored at −70 °C until analysation. To evaluate insulin and C-peptide secretion, the Ultrasensitive ELISA kit (#10-1132-01, #10-1141-01, Mecodia, Uppsala, Sweden) was used according to the manufacturer’s protocols.
Statistical analysis
All studies were performed in triplicate independently. The results were analysed by one-way ANOVA and Bonferroni’s post hoc test by GraphPad Prism 6 software (GraphPad Software Inc., La Jolla, CA). The data were reported as a mean ± standard deviation (SD). p values less than .05 were considered statistically significant.
Results
Morphological changes during differentiation
To generate IPCs from hiPSCs, a previously reported stepwise differentiation protocol was used with some modifications. Under the inverted and SEM, cell morphologies were recorded at the end of differentiation stages ( and ). SEM images showed the porous nanofibrous scaffold with numerous interconnected pores (). After the cells were cultured, hiPSCs took an elongated and flat shape in control group (); while the differentiated cells in the experimental group were a cluster-shaped structure which is a typical morphological feature of pancreatic islet (). Finally, these cluster-shaped structures were used for further evaluation to test the efficiency of differentiation protocol in different groups.
Figure 1. Morphological changes of cultured cells in tissue culture plates. (a) hiPSCs in DMEM (control group). (b) hiPSCs in IPC differentiation media at day 21. Scale bars are 100 µm.
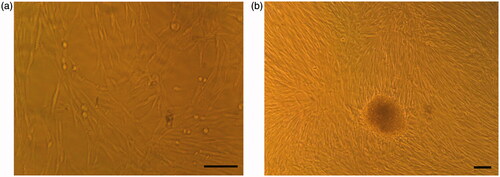
Figure 2. Morphological changes of cultured cells on the PES nanofibrous scaffold. (a) Unseeded scaffold with interconnected pores. (b) hiPSCs-seeded on the nanofibrous scaffold (control group). The cells show spindle-shaped morphology. (c) The hiPSCs-seeded scaffold in IPC differentiation media at day 21. The cells are round in shape and form spherical dense clusters. Scale bars are a, b: 30 µm and c: 10 µm.
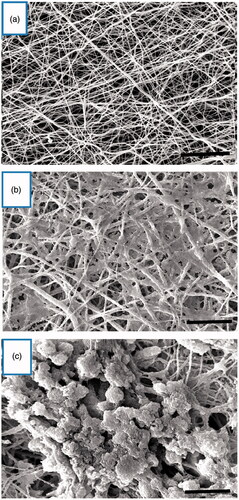
Cell viability
MTT results showed the biocompatibility of PES scaffold for hiPSCs. As shown in , the viability of the hiPSCs on the scaffold was significantly higher than the tissue culture plate group at days 5 and 7, although there were no significant differences at days 1 and 3 between these two groups.
Expression of pancreatic islet genes
Differentiation of hiPSCs into IPCs was determined by detection of pancreatic tissue-specific markers at mRNA level using the RT-qPCR method. As shown in , the expression level of Pdx1, Insulin, Glucagon and Glut2 genes in the undifferentiated hiPSCs control group was low, however, the expression of these genes increased significantly in both tissue culture plates and PES scaffolds differentiated cells. The result of RT-qPCR showed the expression of pancreatic specific genes was higher in the 3D group compared with the 2D group (1.6, 1.4, 1.5, 1.5 and 1.7 fold changes for pancreatic and duodenal homeobox 1 (Pdx-1), Glucagon, Glut-2, Ngn3 and Insulin, respectively).
Figure 4. Relative expression of pancreatic-specific genes in end-stage derived-IPCs. Gene transcripts of the PES scaffold group are compared to the tissue culture plate group. Relative gene expressions were normalized to the human β2M as a reference gene. The value is shown in each graph as mean ± SD. *p < .05, **p < .01, ***p < .001 and ****p < .0001.
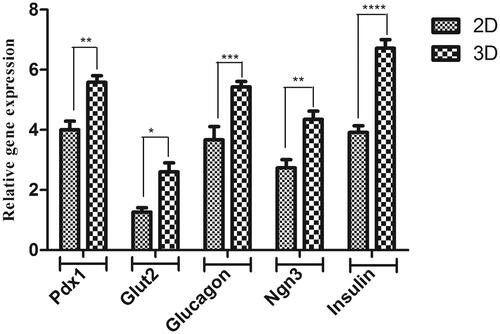
Immunofluorescence staining
Immunocytochemistry results did not show any Pdx1 and Insulin genes expression in tissue culture plates and PES scaffolds groups (data not shown). As shown in , weak or moderate expression of Pdx1 and Insulin was observed in the nuclei and cytoplasm of the differentiated tissue culture plates experimental culture at day 21. In PES scaffolds experimental culture group, strong immune-reactivity of both markers was observed in the nuclei and cytoplasm of differentiated IPCs at day 21. Furthermore, immunocytochemistry results did not show any Pdx1 and Insulin genes expression in tissue culture plates and PES scaffolds control groups (data not shown).
Figure 5. Immunocytochemical analysis of differentiated cells in end-stage derived-IPCs. Nuclei localization of Pdx1 and cytoplasmic localization of Insulin in differentiated IPCs at day 14 in the PES scaffold group (a) and tissue culture plate group (b) were detected by immunofluorescence analysis. Counter-staining of the nucleus (blue) was performed by DAPI and images were obtained by a fluorescence microscope. Scale bars are 100 µm.
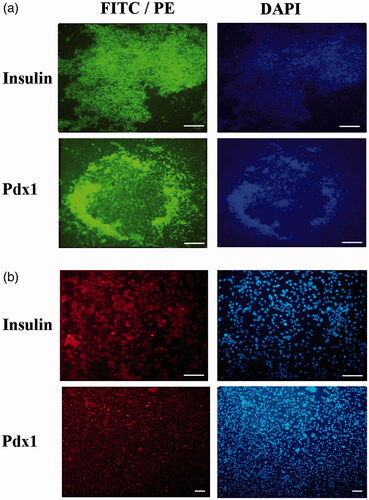
Insulin and C-peptide release in response to glucose challenges
The cultured hiPSCs in control groups indicated very low levels of insulin and C-peptide secretion in response to glucose challenges. As shown in , there were no significant differences in insulin and C-peptide secretion at 5.5 mM glucose between tissue culture plates and PES scaffolds groups. However, differences in both insulin and C-peptide secretion were significant at 15 and 25 mM glucose concentration.
Figure 6. In vitro release of insulin and C-peptide in IPCs derived at day 21 of differentiation. (a and b) Insulin secretion and C-peptide release changes in two groups of IPCs and control group in response to various concentrations of glucose from 5.5 to 25 mM. Values are expressed as mean ± SD (n = 6). *p < .05.
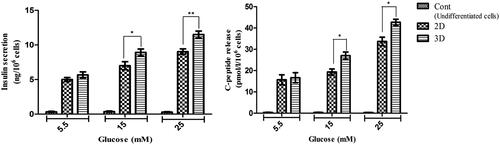
Discussion
The emergence of iPSCs technology encouraged the researchers to use these cells for the production of beta cells. Due to their capacity in self-renewing, less immunogenicity and differentiation potential, iPSCs became preferred choice to generate various tissues such as liver [Citation14], neural [Citation15], bone [Citation16], cartilage [Citation17], etc. Similarly, researchers have tried to use iPSCs and produce insulin-secreting cells to treat patients with T1DM [Citation6,Citation9]. Several studies have shown that iPSCs can effectively differentiate into IPCs with a greater success in the case of three-dimensional cell culture environments [Citation11,Citation18]. As generated from the reprogramming of the patient’s somatic cells, iPSCs are considered as an appropriate option for cell therapy in clinics [Citation19].
In this study, the differentiation potential of hiPSCs into IPCs on PES nanofibers scaffold compared with cells differentiated in tissue culture dishes was examined. During differentiation period, the morphology of cells gradually changed and finally spherical-round clusters formed where observed under inverted microscopy and SEM in the case of tissue culture plates and PES scaffolds, respectively. These clusters which formed after 21 days of differentiation were similar to islets observed within pancreatic tissues.
RT-qPCR and immunofluorescence analysis revealed the expression of pancreatic specific markers in differentiated cells. These markers include Pdx-1, a transcriptional factor which plays important roles in pancreatic development [Citation20–22], Glucagon which is produced in the alpha cells within the pancreas [Citation23], and Glut-2 that facilitates glucose uptake as a transmembrane carrier and known as a marker of maturity in pancreatic β-cells [Citation24,Citation25]. The result of this study showed expression of these factors was higher in cells cultured on PES nanofibrous scaffold compared to those cultured as two-dimensional in tissue culture plates. Different studies showed that in the PES scaffolds microenvironment, global gene expression, differentiation and proliferation increased [Citation17,Citation26–28]. Furthermore, in this circumstance, cellular behaviour altered due to cellular interactions to neighbouring cells and extracellular matrix [Citation29–31]. Multiple studies have revealed the role of ECM in islet survival [Citation32–34]. It seems that nanofibrous scaffold could mimic a microenvironment existing in vivo within the pancreas. Cells cultured on scaffolds increased their interaction with each other which could increase secretion of insulin from differentiated cells. Furthermore, it is observed that differentiated cells in PES scaffolds environments increased cellular organelles involved in protein synthesis and secretion [Citation35].
Different growth factors including EGF, bFGF, betacellulin and activin A were used in this study to differentiate hiPSCs into insulin-secreting cells. Different studies showed that EGF and bFGF which play important roles in cell proliferation and survival have a key role in pancreatic lineage neogenesis. These growth factors were secreted from endocrine precursors during islet neogenesis and used as chemo-attractants in islet cell clustering [Citation36–38]. Activin A is a member of the transforming growth factor-beta (TGF-β) superfamily. This protein increases proliferation of beta and ductal-cells during pancreas development, as well as enhancing insulin secretion in response to glucose stimuli [Citation39]. It is observed that Activin A could induce high expression of endocrine genes including Pdx-1, insulin and glucagon in mouse embryonic stem cells [Citation40]. Some studies showed that betacellulin as an EGF family could promote differentiation of the different types of stem cells into insulin-secreting cells [Citation41,Citation42]. One of the factors used in this study to IPC differentiation of hiPSCs was a B27 supplement. B27 is used as a neural supplement and preserve neural cells characteristics in culture [Citation43]. Different studies proved that stem cells can differentiate into IPCs in neural pathways [Citation44,Citation45]. As a result, this supplement could support pancreatic differentiation used in different IPC differentiation studies.
Altogether, the results of this study showed that iPSCs cultured on PES nanofibrous scaffolds differentiate into IPCs with proper morphological, molecular and functional characteristics. As a result, it is suggested that PLLA/PVA could be used as an ideal scaffold to provide a proper microenvironment promoting pancreatic differentiation of iPSCs.
Conclusions
The present study showed the capacity of PES nanofibers as a three-dimensional matrix to enhance pancreatic differentiation of hiPSCs. As shown in this study, derived IPCs on PES nanofibers exhibit higher expression of pancreatic-specific markers at mRNA and protein levels compared to the tissue culture plate group. Furthermore, these IPCs were functional in response to glucose challenges. The results of our study showed the effectiveness of PES nanofibers to promote differentiation of hiPSCs into IPCs; however, more research is needed to investigate the function of these IPCs in vivo.
Acknowledgements
We wish to thank Stem Cell Technology Research Center for the use of their laboratory facilities.
Disclosure statement
There is no conflict of interest in this study.
References
- Shaw JE, Sicree RA, Zimmet PZ. Global estimates of the prevalence of diabetes for 2010 and 2030. Diabetes Res Clin Pract. 2010;87:4–14.
- Association AD. Diagnosis and classification of diabetes mellitus. Diabetes Care. 2014;37(Suppl.1):S81–S90.
- Kar P, Holt RI. The effect of sulphonylureas on the microvascular and macrovascular complications of diabetes. Cardiovasc Drugs Ther. 2008;22:207–213.
- Anazawa T, Okajima H, Uemoto S. Pancreatic islet transplantation. Nihon Rinsho Japan J Clin Med 2015;73:2117–2122.
- Ellis C, Ramzy A, Kieffer TJ. Regenerative medicine and cell-based approaches to restore pancreatic function. Nat Rev Gastroenterol Hepatol. 2017;14:612–628.
- Zhang D, Jiang W, Liu M, et al. Highly efficient differentiation of human ES cells and iPS cells into mature pancreatic insulin-producing cells. Cell Res. 2009;19:429–438.
- Kieffer TJ, Woltjen K, Osafune K, et al. Beta-cell replacement strategies for diabetes. J Diabetes Investig. 2017 [cited Oct 6]. DOI: https://doi.org/10.1111/jdi.12758
- Piran M, Enderami SE, Piran M, et al. Insulin producing cells generation by overexpression of miR-375 in adipose-derived mesenchymal stem cells from diabetic patients. Biologicals. 2017;46:23–28.
- Enderami SE, Mortazavi Y, Soleimani M, et al. Generation of insulin‐producing cells from human‐induced pluripotent stem cells using a stepwise differentiation protocol optimized with platelet‐rich plasma. J Cell Physiol. 2017;232:2878–2886.
- Green JJ, Elisseeff JH. Mimicking biological functionality with polymers for biomedical applications. Nature. 2016;540:386–394.
- Aloysious N, Nair PD. Enhanced survival and function of islet-like clusters differentiated from adipose stem cells on a three-dimensional natural polymeric scaffold: an in vitro study. Tissue Eng A. 2014;20:1508–1522.
- Amer LD, Mahoney MJ, Bryant SJ. Tissue engineering approaches to cell-based type 1 diabetes therapy. Tissue Eng B: Rev. 2014;20:455–467.
- Shabani I, Haddadi-Asl V, Seyedjafari E, et al. Improved infiltration of stem cells on electrospun nanofibers. Biochem Biophys Res Commun. 2009;382:129–133.
- Mahmoodinia Maymand M, Soleimanpour-Lichaei HR, Ardeshirylajimi A, et al. Improvement of hepatogenic differentiation of iPS cells on an aligned polyethersulfone compared to random nanofibers. Artif Cells Nanomed Biotechnol. 2017. DOI: https://doi.org/10.1080/21691401.2017.1345929
- Lee H-K, Sanchez CV, Chen M, et al. Three dimensional human neuro-spheroid model of Alzheimer’s disease based on differentiated induced pluripotent stem cells. PLoS One. 2016;11:e0163072.
- Ardeshirylajimi A. Applied induced pluripotent stem cells in combination with biomaterials in bone tissue engineering. J Cell Biochem. 2017;118:3034–3042.
- Mahboudi H, Soleimani M, Enderami SE, et al. The effect of nanofibre-based polyethersulfone (PES) scaffold on the chondrogenesis of human induced pluripotent stem cells. Artif Cells Nanomed Biotechnol. 2017. DOI: https://doi.org/10.1080/21691401.2017.1396998
- Hoveizi E, Nabiuni M, Parivar K, et al. Definitive endoderm differentiation of human-induced pluripotent stem cells using signaling molecules and IDE1 in three-dimensional polymer scaffold. J Biomed Mater Res A. 2014;102:4027–4036.
- Maehr R, Chen S, Snitow M, et al. Generation of pluripotent stem cells from patients with type 1 diabetes. Proc Natl Acad Sci. 2009;106:15768–15773.
- Stoffel M, Stein R, Wright CV, et al. Localization of human homeodomain transcription factor insulin promoter factor I (IPF1) to chromosome band 13q12.1. Genomics. 1995;28:125–126.
- D’Amour KA, Bang AG, Eliazer S, et al. Production of pancreatic hormone-expressing endocrine cells from human embryonic stem cells. Nat Biotechnol. 2006;24:1392–1401.
- Bai C, Gao Y, Li Q, et al. Differentiation of chicken umbilical cord mesenchymal stem cells into beta-like pancreatic islet cells. Artif Cells Nanomed Biotechnol. 2015;43:106–111.
- Jiang G, Zhang BB. Glucagon and regulation of glucose metabolism. Am J Physiol-Endocrinol Metabol. 2003;284:E671–E6E8.
- Zhao F-Q, Keating AF. Functional properties and genomics of glucose transporters. Curr Genomics. 2007;8:113–128.
- Guillam M-T, Hümmler E, Schaerer E, et al. Early diabetes and abnormal postnatal pancreatic islet development in mice lacking Glut-2. Nat Genet. 1997;17:327–330.
- Nadri S, Barati G, Mostafavi H, et al. Differentiation of conjunctiva mesenchymal stem cells into secreting islet beta cells on plasma treated electrospun nanofibrous scaffold. Artif Cells Nanomed Biotechnol. 2017. DOI: https://doi.org/10.1080/21691401.2017.1416391
- Enderami SE, Soleimani M, Mortazavi Y, et al. Generation of insulin‐producing cells from human adipose‐derived mesenchymal stem cells on PVA scaffold by optimized differentiation protocol. J Cell Physiol. 2017 [cited Nov 18]. DOI: https://doi.org/10.1002/jcp.26266
- Javad H, Parvin P, Masoud S, et al. Application of a novel bioreactor for in vivo engineering of pancreas tissue. J Cell Physiol. 2017 [cited May 13]. DOI: https://doi.org/10.1002/jcp.26004
- Birgersdotter A, Sandberg R, Ernberg I. Gene expression perturbation in vitro—a growing case for three-dimensional (3D) culture systems. Semin Cancer Biol. 2005;15:405–412.
- Birhanu G, Akbari Javar H, Seyedjafari E, et al. An improved surface for enhanced stem cell proliferation and osteogenic differentiation using electrospun composite PLLA/P123 scaffold. Artif Cells Nanomed Biotechnol. 2017. DOI: https://doi.org/10.1080/21691401.2017.1367928
- Islami M, Mortazavi Y, Soleimani M, et al. In vitro expansion of CD 133+ cells derived from umbilical cord blood in poly-l-lactic acid (PLLA) scaffold coated with fibronectin and collagen. Artif Cells Nanomed Biotechnol. 2017. DOI: https://doi.org/10.1080/21691401.2017.1358733
- Bosco D, Meda P, Halban PA, et al. Importance of cell–matrix interactions in rat islet beta-cell secretion in vitro: role of alpha6beta1 integrin. Diabetes. 2000;49:233–243.
- Stendahl JC, Kaufman DB, Stupp SI. Extracellular matrix in pancreatic islets: relevance to scaffold design and transplantation. Cell Transplant. 2009;18:1–12.
- Mooranian A, Negrulj R, Takechi R, et al. Electrokinetic potential-stabilization by bile acid-microencapsulating formulation of pancreatic β-cells cultured in high ratio poly-l-ornithine-gel hydrogel colloidal dispersion: applications in cell-biomaterials, tissue engineering and biotechnological applications. Artif Cells Nanomed Biotechnol. 2017. DOI: https://doi.org/10.1080/21691401.2017.1362416
- Baharvand H, Hashemi SM, Ashtiani SK, et al. Differentiation of human embryonic stem cells into hepatocytes in 2D and 3D culture systems in vitro. Int J Dev Biol. 2004;50:645–652.
- Norgaard GA, Jensen JN, Jensen J. FGF10 signaling maintains the pancreatic progenitor cell state revealing a novel role of Notch in organ development. Dev Biol. 2003;264:323–338.
- Herbst RS. Review of epidermal growth factor receptor biology. Int J Radiat Oncol Biol Phys. 2004;59:S21–SS6.
- Hardikar AA, Marcus-Samuels B, Geras-Raaka E, et al. Human pancreatic precursor cells secrete FGF2 to stimulate clustering into hormone-expressing islet-like cell aggregates. Proc Natl Acad Sci. 2003;100:7117–7122.
- Florio P, Luisi S, Marchetti P, et al. Activin A stimulates insulin secretion in cultured human pancreatic islets. J Endocrinol Invest. 2000;23:231–234.
- Park M-K, Han C, Lee K-H, et al. Effects of activin A on pancreatic ductal cells in streptozotocin-induced diabetic rats. Transplantation. 2007;83:925–930.
- Zalzman M, Anker-Kitai L, Efrat S. Differentiation of human liver-derived, insulin-producing cells toward the β-cell phenotype. Diabetes. 2005;54:2568–2575.
- Mashima H, Shibata H, Mine T, et al. Formation of insulin-producing cells from pancreatic acinar AR42J cells by hepatocyte growth factor. Endocrinology. 1996;137:3969–3976.
- Brewer GJ, Torricelli J, Evege E, et al. Optimized survival of hippocampal neurons in B27‐supplemented neurobasal™, a new serum‐free medium combination. J Neurosci Res. 1993;35:567–576.
- Lin H-Y, Tsai C-C, Chen L-L, et al. Fibronectin and laminin promote differentiation of human mesenchymal stem cells into insulin producing cells through activating Akt and ERK. J Biomed Sci. 2010;17:56.
- Alpert S, Hanahan D, Teitelman G. Hybrid insulin genes reveal a developmental lineage for pancreatic endocrine cells and imply a relationship with neurons. Cell. 1988;53:295–308.