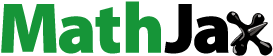
Abstract
Visceral leishmaniasis (VL) is World Health Organization designated most serious leishmaniasis with an annual mortality rate of 50,000. Even after country specific eradication programs, the disease continues to multiply with added complexities like resistance development, drug hypersensitivity and associated infections. Newer therapeutic interventions are urgently warranted to control the spread. Present study aims to arrive at terpenoid andrographolide engineered gold nanoparticle (AGAunps) facile synthesis and its efficacy evaluations against wild and drug resistant VL strains for the first time. Molecular bio-organic conjugation of AGAunp was confirmed in FT-IR and EDAX studies. Nano-gold plasmon response was recorded at 543 nm and the average size in TEM was 14 nm. SAED pattern and XRD observations proved fcc crystalline structure of nano-gold. AGAunp recorded spherical geometry in AFM and TEM. PDI value of 0.137 revealed the monodisperse nature of the nano-scale population. AGAunp exhibited strong antileishmanicidal effect both against wild type (IC50 19 ± 1.7 µM) and sodium stibogluconate (IC50 55 ± 7.3 µM)/paromomycin (IC50 41 ± 6 µM) resistant strains. Complete macrophage uptake AGAunp’s occured within two hours exposure. AGAunp macrophage cytotoxicity was significantly lower as compared to Amphotericin-B. Low toxic Andrographolide engineered gold nanoparticle emerged as promising alternatives in the control of wild and drug resistant VL.
Graphical Abstract
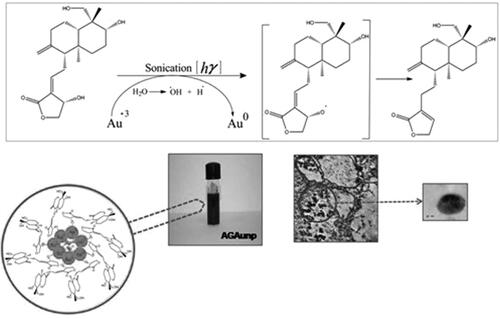
Introduction
Leishmaniasis is a complex neglected tropical disease (NTD) caused by more than 20 leishmanial parasites and transmitted to humans by ∼30 different species of phlebotomine sandflies [Citation1]. World Health Organization (WHO) specifies visceral leishmaniasis (VL) as the most serious form of leishmaniasis caused by either L. donovani or L. infantum with a mortality rate of 50,000 per year [Citation2]. Among these two causative parasites L. donovani is prominent in all age groups [Citation1] but its geographical spread is restricted to East Africa and India. L. infantum infection is mostly observed in child and immune-deficient patients of Europe, North Africa and Latin America [Citation3]. Nearly 90% of VL cases transpire in poverty stricken rural belts of India, Bangladesh, Nepal, Sudan and Brazil, with the Indian state of Bihar accounting for half the VL cases globally [Citation4]. VL infection inhabits the host’s skin for lifetime even after successful chemotherapy and the complexity escalates with the chances of relapse linked with the impairment of immune function. Pentavalent antimonials like sodium stibogluconate and meglumine antimoniate, polyene antibiotics like amphotericin-B or orally active phosphocholines like miltefosine are the present first-line therapeutics in VL management. But clinical acceptance of these agents is witnessing a steep decline due to widespread resistance development [Citation5], inherent toxicity and cost of therapy. L. donavani has developed resistance even against drug combinations like amphotericin-B with sodium stibogluconate [Citation6], which further paralyzes VL management.
Drug resistance in leishmaniasis is related to sundry of mechanisms like reduced drug uptake, enhanced drug efflux, drug biotransformation or avoidance of drug activation [Citation7]. Fluctuations in drug efflux or drug uptake and sometimes their combined effect influence resistance to first or second-line antileishmanial therapeutics. Researchers relate the homology observed between leishmanial and human multidrug resistance genome as the probable stimulus for this phenomenon [Citation5]. Considering the existing gap in VL treatment and the limited armory of existing drugs, WHO has expressed urgent call for newer therapeutics development for this NTD.
Nanoscale gold with its distinctive features like functional versatility, biocompatibility, low/non-toxicity and adjustability in size/shape is ideal for application in therapy and detection [Citation8]. Additionally, speedy entry of nano-scale metal devices in infected cellular environment compared to polymeric ones provides an added advantage. Typical synthetic route for nano-scale gold involve reduction of tetrachloroauric acid by sodium borohydride/sodium citrate with stabilizers like CTAB (cetyltrimethylammonium bromide). Acceptability of this approach is sharply declining due to the impact of reactants like CTAB on biocompatibility. Alternative greener synthesis methods are now preferred where environment friendly solvents, non-toxic reducing agents and stabilizers are explored. Plant extracts and micro-organisms mostly play the role of reducing agents in such typical synthesis process [Citation9–11]. Phyto-fabrication of nano-scale metals overshadow microorganism based synthesis as it is cost-effective, faster, easy to scale up and moreover does not require complex procedures like microorganism isolation, identification, growth optimization, culture preparation and maintenance. Chemically distinct plant derived compounds like amino acids, enzymes, flavonoids, aldehydes, ketones, amines, carboxylic acids, phenols and alkaloids can act as potential inducers for the reduction of metal ion to elemental metal. Particle geometry and size of nano-metals synthesized by plant bioactives depend on reaction variables like pH, temperature, incubation time, concentration of reducing agent/metal ion, order of addition of reagents, type and degree of agitation and protective agents used [Citation12–15]. These techniques are ideal for large-scale synthesis of well-characterized nano-scale population even though steady reproducibility in particle size, shape and polydispersity following such techniques still needs rigorous experimentation. Keeping leishmania management in the backdrop nano-scale gold is reported to affect the viability of leishmanial parasite [Citation16] and also aid in detection of parasitic genome [Citation17]. Besides AuNp exhibits size dependent uptake in macrophages where the leishmanial parasites reside during infection. This multiplies the chances of success of Aunp in leishmanial chemotherapy.
Bioactives of plant origin with leishmanicidal activity and lesser risk of resistance development [Citation18] can be useful alternatives in the current context. These compounds can provide affordable therapy with minimal inherent toxicity, which adds to their value. Terpenoids are more promising members of this class due to their selectivity towards leishmania biochemical targets [Citation19]. Andrographolide (AG), is a member of the terpenoid family with reported leishmanicidal effect both in-vitro and in-vivo [Citation20]. Pharmacology of AG in leishmania is linked with its capacity to regulate reactive oxygen species and mitochondrial membrane potential [Citation21]. Our previous report with flavonoid engineered nano-gold showed high selectivity and significant efficacy in both wild and drug-resistant leishmaniasis [Citation22]. This work is the first report of facile one-pot synthesis of AG engineered gold nanoparticle (AGAuNp) in drug-resistant VL. Efficacy reports of AG and AuNP’s in leishmania spearheaded the idea that their association in nano-scale would favour synergism and size-guided cellular entry resulting in improved efficacy. Present study deals with the synthesis, characterization, antileishmanial studies, cytotoxicity assays and macrophage uptake experiments of AGAunp’s.
Materials and methods
Materials
Andrographolide [C20H30O5] (AG) was procured from Natural Remedies (Bangalore, India). Chloroauric acid (HAucl4,3H2O), Amphotericin B, paromomycin, medium 199, foetal calf serum and RPMI-1640 cell culture medium were purchased from Sigma-Aldrich (St. Louis, MO). All solvents and water used were of HPLC grade (E. Merck or Spectrochem, Mumbai, India). Sodium stibogluconate (SbV) was a kind gift from Albert David Ltd. (Kolkata, India). Penicillin and streptomycin were acquired from Gibco (CA, USA). Chemicals were used as received in the entire study without further modification or purification.
Methods
Synthesis of AG engineered gold nanoparticle (AGAunp)
AG engineered gold nanoparticle was synthesized following a facile one-pot technique. Briefly aqueous solution of HAucl4 (1.3 mM) was made using 100 ml HPLC grade water. Then 1 ml of 5.46 mM methanolic solution of AG was added to 3 ml of 1.3 mM aqueous HAucl4 and kept aside for equilibration for 5 min under gentle mixing at 45 °C. Reaction mixture was then sonicated in a bath sonicator, UD 100SH-3 L (Takashi, Tokyo, Japan) regulated at 50 HZ and constant temperature of 45 °C. The plasmon response was recorded up to 30 min at different time points by UV-vis spectrophotometric scan. Colour variation of the solution during the reaction time of 30 min was also noted. Complete reduction of Au+3 to Au0 was accompanied by colour change from yellowish to purple.
AGAunp’s were then separated by ultracentrifugation (Himac CS120GHXL, HitachiKoki, Tokyo, Japan), at 12900 rcf for 45 min. Separated AGAunp’s were re-dissolved in water and the process of ultracentrifugation was replicated to concentrate the product. Concentrated AGAunp population were finally dispersed in water and preserved at 4 °C for further experiments. Glassware used for AGAunp synthesis was cleaned with aqua regia (3:1 HCl/HNO3), rinsed with deionized water for several times and oven-dried.
Characterization of AGAunps
Optical characteristics of AGAunps were examined by double beam UV-vis spectrophotometer UV – 2550 (Shimadzu, Japan) using 1 cm path length quartz cuvettes and HPLC water for background correction. Surface plasmon resonance of synthesized AGAunp was recorded for different time intervals and different molar ratios for AG and HAuCl4 for optimization of the two reaction variables. Hydrodynamic diameter and polydispersity index (PDI) of AGAunp’s were determined against a 4 mw He-Ne laser beam, of wavelength 633 nm and a back-scattering angle of 173° in Zetasizer Nano ZS (Malvern Instruments, Malvern, UK). Zeta potentials (ζ) measurements in aqueous environment based on electrophoretic mobility under an applied electric field was calculated using Smoluchowski equation. Fourier Transform Infrared Spectroscopy (FT-IR) analysis of AG engineered gold nanoparticle was done in FT/IR-670 plus Spectrometer (Jasco, Tokyo, Japan). Powdered AG and purified AGAunp’s were grinded separately with IR grade KBr (Pike technologies, UK) and pressurized to form pellets which were then scanned over the range 4000 to 400 cm−1 involving 500 scans with a resolution of 2 cm−1. FT-IR data were stacked in Bio-Rad KnowItAll (Bio-Rad, Hercules, CA) software for comparative analysis. The Au concentration in the optimized AGAunp was determined by inductively coupled plasma mass spectrometry (ICP-MS). For this analysis AGAunp samples were digested for 2 h in aqua regia for the experiment. The instrument (Elan-DRC-e, Parkin Elmer, USA) was calibrated using solutions from instrument standards at concentrations ranging from 50 to 1000 ppb, containing 1% nitric acid. Gold concentration in AGAunp was determined using Au197 isotope following operating conditions 1050 Hz radio-frequency forward power, 12.8 L min−1 plasma carrier gas flow (argon) and 26 rpm for the nebulizer pump.
The morphology of AGAunp was investigated by atomic force microscopy (AFM) Pico plus 5500 ILM (Agilent). Aqueous AGAunp suspension (20 µl) was drop coated onto fused mica substrate and dried in air (65% humidity) for 30 min. Morphology of AGAunp’s was traced in AFM using tapping mode with cantilever resonance frequency 150–300 kHz. Images were captured and analysed with Picoview 1.10.4 software. High resolution transmission electron microscopy (HR-TEM) analysis was conducted using JEOL-JEM 2010 (JEOL, Tokyo, Japan) electron microscope to assess the size, size distribution and shape of AGAunp’s. Carbon-coated copper TEM grids were coated with aqueous nanoparticle suspension droplets, dried and then exposed to TEM analysis in bright field mode at an accelerated voltage of 200 kV. Crystallinity of the nanoparticle was evaluated from selected area electron diffraction (SAED) pattern. HR-TEM instrument was equipped with an energy-dispersive X-ray (EDAX) device to detect composition of the optimized nanoparticle. The solid-state dispersion of AGAunp’s was evaluated by using X-ray powder diffraction in X-ray diffractometer Bruker AXS D8 (Bruker, Karlsruhe, Germany). The Cukα radiation (λ = 1.5405 Å) at an accelerating voltage of 40 kV with a current intensity of 40 mA was used for the study. The XRD spectra was recorded in the 2θ range of 30–80° with a step size of 0.02° in a 2θ scattering angle and a scan speed of 0.01°/sec.
Antileishmanial evaluation
Animals
Female BALB/c mice of 20–25 g body weight and approximately of same age were used for the entire study. Mice were housed in polypropylene cages under standard laboratory conditions of relative humidity 50% ± 10%, temperature 22 °C ± 2 °C and 12/12 h light–dark cycle. Mice were fed with standard pellet food and water ad libitum. The experimental protocols were approved by the Jadavpur University Animal Ethics Committee (approval no. AEC/PHARM/1601/12/2016). Procedures and techniques followed in the present work were in accordance with the committee’s guideline.
Parasites and culture condition
Leishmania donovani AG83 (MHOM/IN/83/AG83) promastigotes was obtained as a gift from Indian Institute of Chemical Biology, Council of Scientific and Industrial Research, Kolkata, India. The promastigotes were routinely cultured in the medium 199 supplemented with 10% heat inactivated foetal bovine serum, 2 mM L- glutamine, 20 mM HEPES, 100 U/ml penicillin and 100 µg/ml streptomycin at 25 °C [Citation21].
Axenic amastigotes generation
Axenic amastigotes were developed from promastigotes of Leishmania donovani by culturing them in MMA/20 medium in an acidic environment (pH 5.5). The amastigotes were preserved by sub- passage of 105 parasites per ml repeated every fifth day under experimental conditions of 37 °C temperature and 5% CO2 environment [Citation23,Citation24].
Isolation of mice peritoneal macrophages and macrophage viability assay
BALB/c mice was used for isolation of peritoneal macrophages. The mice were injected with 1.5 ml of sterile thioglycolate medium i.p. and on the 4th day after injection the animals were sacrificed by cervical dislocation. Peritoneal exudates of mice were harvested by intra-peritoneal lavage in cold RPMI-1640 medium. Cells were centrifuged at 200 × g for 15 min, counted with a haemocytometer and re-suspended at a concentration of 4 × 105/ml in RPMI-1640 medium without supplements. The viable macrophage population was identified by trypan blue exclusion assay [Citation25]. The mean data of three independent cell counts of viable and non-viable cells were calculated using microscope haemocytometer assembly and used for analysis.
Development of sodium stibogluconate and paromomycin resistant amastigote strain
Drug resistant Leishmania donovani cells were developed as per earlier reports from our group [Citation22]. Briefly, resistance was induced in Leishmania donovani AG83 promastigotes by gradual increasing dose of sodium stibogluconate (SSG) or paromomycin (PMM) in medium 199 with additional supplements [Citation24]. Dose escalation of SSG/PMM was continued until viable population reduced to around 20%. The strain generated with 10% viable cell population of initial count was finally cultured on medium 199 agar plates and a single colony from the culture was selected for further experiments. Drug resistant promastigote phenotypes were differentiated into amastigotes after 120 h of culture in MMA/20 medium in acidic environment (pH 5.5) and 5% CO2/air atmosphere maintained at 37 °C.
Drug sensitivity assay against axenic amastigote
Drug sensitivity to axenic Leishmania donovani amastigotes were evaluated using a modified method of promastigote direct counting growth inhibition assay [Citation26]. For IC50 (50% inhibitory concentration) determination of test agents, 3 × 105 cells per ml amastigote concentration (equivalent to early log phase concentration) were grown in 96 well plates. The amastigotes were then allowed to multiply in contact with serial dilution of test agents or medium alone until late log phase (1 × 106 cells/ml) in maintenance media under 37 °C in a 5% CO2 environment. After three days of exposure, the cells were filtered through 27-gauge needle for separation of clumps and cell count was calculated with microscope haemocytometer assembly. All experimental data were collected in triplicate.
Susceptibility assay to axenic amastigotes in macrophages
Susceptibility of test agents to intracellular amastigotes was evaluated as per following protocol. Briefly 4 × 105 isolated macrophages/ml in 500 µl of RPMI-1640 media were introduced into each well of cell culture plates and incubated for 2 h at 37 °C in 5% CO2 atmosphere. This was followed by replacement of media by 500 µl of warm (37 °C) RPMI-1640 medium supplemented with 10% FCS, 20 mM l-glutamate, 16 mM NaHCO3, penicillin (50 U/ml) and streptomycin (50 µg/ml) after 2 h.
Macrophage/parasite ratio was made to reach 1:10 by addition of 500 µl suspension of RPMI-1640 media containing 4 × 106 axenic amastigotes per ml to each well of the culture plate. Plates were incubated for 4 h at 37 °C in 5% CO2 to allow localization followed by aspiration of the culture medium for separation of free parasites. Test agents were suspended or dissolved in fresh RPMI-1640 medium (1 ml) to reach appropriate concentration and then added to triplicate wells followed by incubation for 72 h at 37 °C in 5% CO2 atmosphere. The medium was again aspirated and the cover slips were removed, fixed and air dried. The cells were exposed to giemsa stain followed by counting of 100 cells on the glass cover slips. For each concentration of test agent, three independent experiments in triplicate were performed for efficacy determination. Results were expressed as the ratio between the infection proportions of treated and untreated macrophage cultures.
Cytotoxicity assay and selectivity index
The cytotoxicity experiments were conducted in 96-well cell culture plates having standard macrophage population grown in RPMI-1640 medium supplemented with 10% FCS, 20 mM L-glutamate, 16 mM NaHCO3, penicillin (50 U/ml) and streptomycin (50 µg/ml). The wells were seeded with drug solutions and the viable macrophages were counted under microscope using haemocytometer after 48 h of exposure at 37 °C in a 5% CO2 incubator. Selectivity index (SI) is a parameter which predicts the highest exposure of the test solution which produces the desired effect without any toxic implications [Citation27]. The selectivity index of the drug and the nanoparticle are calculated as per the formula
Where, CC50 referred as the concentration required to kill 50% of the host cell population and IC50 is the concentration required to kill 50% of the parasites inside the host cell. Generally, if SI value is ≥10, then the test agent is acknowledged to have promising activity [Citation28] for further evaluations.
Macrophage uptake studies in TEM
Macrophages were seeded at a concentration of 2 × 106 cells per well on six well tissue culture plates and incubated overnight for growth under 5% CO2 at 37 °C. After 24 h of incubation, macrophages were treated with a fixed concentration (10 µM) of AGAunp for different time interval (10 min, 30 min, 1 h and 2 h) based uptake studies. On completion of the scheduled time period cells were washed with 0.1 M phosphate buffer saline (PBS) and pellets were centrifuged for three repeated cycles at 3000 rpm for 10 min.
Cleaned cells were collected and fixed for 2 h in a blend of 2% paraformaldehyde and 2.5% glutaraldehyde in 0.1 M PBS [Citation29]. Then the cells were washed and finally stained with 1% Osmium tetraoxide for 1 h at 4 °C. Staining was followed by dehydration of the cells using graded concentration of acetone (30, 50, 70, 90 and 100%) and toluene. Stained cells were housed in epoxy resin and sliced into 80-nm thin sections for TEM analysis (FP 5018/40 (TECHNAI G2 SPIRIT BioTWIN, Czech Republic) at 75 kV.
Statistical analysis
The results of the study are stated as mean ± standard deviation (standard of mean). Biological data were obtained in triplicate and were statistically analyzed by Student’s t-test. Differences were considered statistically significant when p < .05.
Results and discussion
Structure characterization
Synthetic routes for arriving at nano-scale gold mainly revolves around two types of reactions condensation and reduction [Citation30–32]. Condensation method accumulates particles and grows them on a cooled template using the condensation of gold ions, while the reduction method employs reducing agents to reduce the metal ions to yield nano-scale gold. The most accepted reduction method is the Frens method which uses citrate ions in aqueous media not only to reduce the gold salt at boiling temperature but also to stabilize the nano-gold by electric repulsion [Citation33]. But Frens method is limited to nano-gold up to ∼50 nm in size, beyond which the nano-scale population are polydispersed and non-spherical. Another reduction technique employs stronger reducing agent sodium borohydride to prepare smaller (2–10 nm) nano-gold which are stabilized by physically adsorbed boron species on their surface [Citation34]. In both cases because of weak physisorption of the capping agent with nano-scale gold, aggregation occurs upon repeated centrifugation, dilution, dialysis and upon contact with biological media [Citation35]. Thus, surface engineering with stable capping agent is essential to prepare stable and tunable nano-gold with lesser tendency of aggregation. Plant-bioactives like AG with dual role of reducing and capping agent aptly fit into this gap providing an eco-friendly path for facile one pot synthesis of nano-scale gold.
For optimization of reaction variables of AGAuNP synthesis, different reactions were carried out by varying the molar concentration of AG against a fixed molar concentration (1.3 mM) HAuCl4 under sonication for 30 min. The appearance of stable purple colour due to the quantum confinement effect is directly linked to the size of the nano-gold. The free electrons confine themselves within gold nano-population and displays distinctive collective coherent oscillations called surface plasmon resonance signifying formation of nano-scale gold. Appearance of stable purple colour was confirmed as the end point for all reaction mixtures. The UV peak intensities of reaction mixtures increased up to molar ratio of (HAuCl4: AG) 1:1.4 and then decreased with a shift of plasmon resonance peak from 543 to 556 nm (). Free radical scavenging activity of AG (0.1 mg/ml) against DPPH radical is lesser (40.02%) compared to 0.1 mg/ml quercetin (89%) [Citation36]. Antioxidant quercetin form stable end product by donating hydroxyl hydrogen to interrupt free radical chain oxidation. Chemically AG belongs to the category of α,β unsaturated lactones, that are unable to attain extensive resonance stabilized system of conjugated double bonds. Thus, unlike our previous report of quercetin stabilized nano-gold at low temperature conditions [Citation22], AG induced reduction of Au+3 to Au0 required assistance by initiators like temperature and sonication. Sonication results in acoustic cavitations which liberates high energy in the form of temperature and pressure [Citation37] facilitating the transformation of HAuCl4 into nano-scale gold. Single SPR spectra was recorded for all molar variations of HAuCl4: AG confirming the presence of spherical isotropic nano-scale population in accordance with Mie’s theory [Citation38]. ICPMS analysis of gold concentration in AGAunp recorded a value of 265 µg/ml where the reaction was initiated with gold concentration 331 µg/ml. Therefore, calculated percentage yield for gold in AG induced reduction was 80%.
Figure 1. (A) UV-Vis spectra of AGAunps using different molar ratio of HAuCl4: AG (a) 1:1, (b) 1:1.1, (c) 1:1.2, (d) 1:1.4, (e) 1:1.6, (f) 1:1.8. (B) UV-Vis spectra as a function of reaction time for the reaction of Au and AG at (a) 10 min, (b) 20 min, (c) 25 min, (d) 30 min, (e) 40 min and (f) 50 min.
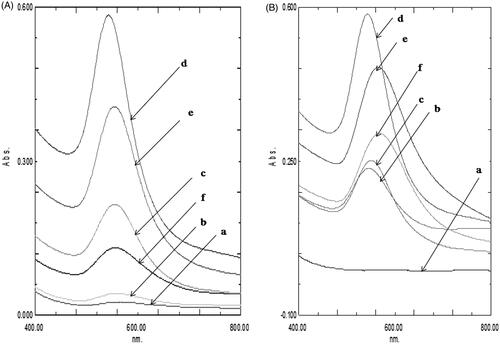
The reaction was tracked meticulously by recording nano-gold surface plasmon resonance as a function of reaction time () in a spectrophotometer. The optimization of reaction variables for bottom up synthesis of AG engineered nano-gold was achieved through multiple trial and error runs. The reduction reaction of gold with AG started after 15 min as indicated by the appearance of purple colour in the aqueous environment. Gold SPR band was centred at about 543 nm and the reduction of gold salt reached saturation within 30 min of reaction time. Further increase in reaction time did not increase the peak intensity at 543 nm signifying the completion of reaction. Experiments runs with more than 30 min reaction time revealed a red shift of SPR band of gold to 565 nm with the formation of agglomeration. Though plant extracts are now widely experimented for synthesis of nano-scale gold, the approach is limited by factors like formation of reaction biproducts and their purification. These issues were addressed by using pure diterpenoid AG for AGAunp synthesis.
Particle size and zeta potential
Dynamic Light Scattering (DLS) methods was used to determine particle size, size distribution, PDI and zeta potential of AGAunp. The hydrodynamic mean diameter, PDI and zeta potential for synthesized AGAunp were 83.25 nm, 0.137 and −21.3 mV, respectively (). Size distribution studies of AGAunp in DLS revealed a gaussian shape with a characteristic V-shaped phase plot observed in nano-scale population with negative zeta potential. Increase in PDI is associated with decrease in monodispersity of the sample under observation. The recorded PDI of AGAunp indicates the monodisperse nature of the nano-gold population [Citation38]. TEM studies are routinely used for detection of size, size distribution and shape of diverse nano-scale entities but they fail to provide data regarding presence of agglomeration in solution. This can be overcome by DLS which can detect the hydrodynamic diameter of nano-scale population in solution. Our DLS results reveal a comparatively larger hydrodynamic size of AGAunp in measurement conditions compared to TEM results discussed later. The size enhancement can be the impact of water absorption on electrostatically stabilized nano-scale gold populations [Citation39].
TEM and AFM analysis
HRTEM analysis of AGAunp population revealed a narrow size distribution as observed in DLS with an average diameter of 14.004 nm (). Average AGAunp diameter D (14.004 nm) with standard deviation σ (8.512) were calculated using EquationEquations (1)(1)
(1) and Equation(2)
(2)
(2) where di represents the diameter of AGAunp, ni represents the number of AGAunp having diameter di and N stands for the total number of AGAunp counted in the observation scale. AGAunp population appeared fairly monodispersed in TEM in accordance with PDI value recorded in DLS. AGAunp mostly exhibited spherical geometry as evident from the scale of observation.
(1)
(1)
(2)
(2)
Figure 3. (A) TEM micrograph of AGAunp’s, (B) Histogram of AGAunp size distribution, (C) High resolution TEM micrograph of single AGAunp and (D) AGAunp SAED pattern.
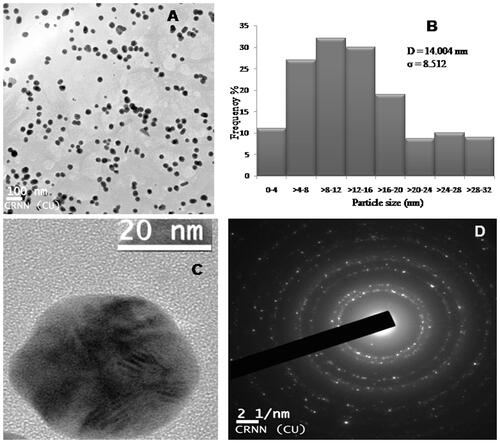
Single AGAunp was focused () in HRTEM and selected area electron diffraction pattern (SAED) was investigated (). HRTEM observation clearly indicated crystal growth lattice planes with lattice fringe spacing of 0.18 nm. The clear lattice fringes in HRTEM image and bright circular rings corresponded to (111), (200), (220) and (311) planes. The findings substantiated the crystalline nature of AGAunp.
EDAX spectrum was investigated from the TEM samples to interpret the elemental composition of AGAunp’s (). EDAX data contained carbon and oxygen peaks but was devoid of any nitrogen peaks. The results confirm the association of AG molecules for engineering the formation of nano-scale gold. AFM scan of AGAunp correlated well with the TEM findings confirming spherical shape characteristics and smooth surface texture ().
XRD spectra
XRD spectra of purified AGAunp powder is presented in . Bragg’s diffraction in AGAunp was recorded at 38.20°, 44.42°, 64.75°, 77.60°, which corresponds to (111), (200), (220), (311) sets of lattice plane reflecting fcc structure of metallic gold (JCPDSno.04–0784). A sharp Bragg’s diffraction signal corresponding to (111) lattice compared to (200), (220), (311) lattice planes indicates that gold nano-crystal formation was mainly driven by (111) facets of metallic gold. XRD pattern thus revealed the crystalline nature of the AGAunps. Some minor peaks also appeared in the XRD data which may be due to the associated biomolecules on AGAunp surface.
FT-IR spectroscopy
FTIR spectra characterize the type of bonds and functional groups. Different molecules possess their own vibrational energy thus excitation of molecules with IR laser would result in the absorption at specific wavelength. Similar trend of FTIR spectra was observed for both AG and AGAunp represented in . AG response was apparent in both cases, as indicated by O–H stretching vibrations at 3399 cm−1 due to of intermolecular hydrogen bonded structure and C–O stretching at 1033 cm−1 and at 1076 cm−1 for primary and the secondary alcoholic functions. Characteristic AG lactone responded at 1727 cm−1 while corresponding in plane O–H bending appeared at 1415 cm−1 [Citation40]. Presence of AG in AGAunp was further confirmed by conjugated C = C peak at 1674 cm−1 which corroborated with our previous report [Citation41]. Thus, FTIR data analysis confirms the stabilization of nano-scale gold by diterpenoid AG.
Antileishmanial activity
Metal or metal oxide nanoparticle applications in leishmania are limited with metal ones having the edge as their oxidative capacity lead to more damage to the parasite membrane, enzyme and DNA [Citation42] But presence of inherent cellular toxicity is the major cause of concern for clinical success of both metal and metal oxide nanoparticle. Terpenoid compounds are preferred in leishmania due to their specific affinity for the leishmanial parasite [Citation19] and their ability to alter the parasite cell membrane fluidity at IC50 concentration range [Citation43]. Green synthesis of zero-valent nano-gold stabilized by terpenoid AG was targeted to minimize nanoparticle toxicity and enhance pharmacological benefits due to the association of the biomolecule. Our earlier experiments with nanoparticulated AG in PLGA showed promising leishmanicidal activity against axenic amastigotes and amastigote infested macrophages [Citation21] which further supported the inception for this work. As AG is sparingly soluble in water so the bioactive was initially solvated in DMSO (DMSO concentration did not exceed 0.1% (v/v) in any treatment) with further dilution in culture media for cell-culture studies.
The susceptibilities of both the axenic and intracellular amastigotes to AG and AGAunp were evaluated following standard protocols (Supplemental material). The IC50 values in each case observation ( and ) were compared against known anti-leishmanial drugs sodium stibogluconate (SSG), Paromomycin (PMM) and Amphotericin B. AGAunp expressed about 3-fold better activity than AG against wild type axenic amastigotes (IC5019 ± 1.7 µm) and amastigote infected macrophages (IC50 12 ± 3 µm) ( and ). Interestingly, this activity enhancement also persisted against intracellular PMM resistant (IC50 21 ± 2 µm) and SSG resistant (IC50 44 ± 4 µm) strains (). This may be linked to size-guided fast macrophage entry and enhanced adhesion onto nonflagellated amastigotes [Citation44] due to negative surface charge of AGAunp. Engineering of gold nanoparticle with AG influenced its antileishmanial potency as experiments with citrate stabilized gold nanoparticle proved pharmacologically ineffective up to a dose of 1 mM. AGAunp induced 3- to 5-fold better leishmanicidal effect compared to standard drug PMM in intracellular drug resistant conditions. Impact of AG engineered gold nanoparticle and standard drug SSG on wild type and drug resistant leishmanial strains exhibited similar results. Concerns for gold nanoparticle bioaccumulation and tissue toxicity are widespread. Smaller size nanoparticle (<10 nm) are reportedly more toxic. Though Amphotericin B topped the list among the tested agents in terms of antileishmanial potency, it expressed extreme cytotoxicity (CC50 21 µm) compared to AGAunp (CC50 1813 µm) raising alarming safety concerns (). In fact, cytoxicity profile of AGAunp revealed that it is much safer even than PMM (CC50 274 µm) and SSG (CC50 41 µm) and thereby holds promise in clinical applications. SI value of AGAunp was better than Amphotericin B for wild type (151.08) and PMM resistant intracellular parasites (86.3) which further narrows down the toxicity window of this newer nanoparticle ().
Figure 8. AG/AGAunp/Standard Antileishmanial drugs sensitivity profile against axenic amastigotes for Leishmania donovani and its drug resistant cell lines. WT (Wild Type), SSG (Sodium stibogluconate resistant) and PMM (paromomycin resistant) cell lines. IC50 (μM) values were determined as mean ± SD (n = 4). *p < .05 significant difference compared with SSG.
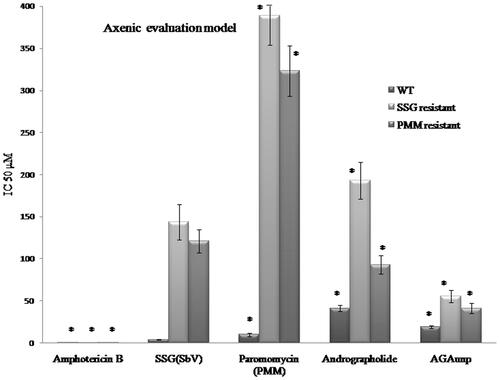
Figure 9. AG/AGAunp/Standard Antileishmanial drugs sensitivity profile against intracellular amastigotes for Leishmania donovani and its drug resistant cell lines. WT (Wild Type), SSG (Sodium stibogluconate resistant) and PMM (paromomycin resistant) cell lines. IC50 (μM) values were determined as mean ± SD (n = 4). *p < .05 significant difference compared with SSG. £p < .5 no significant difference compared with SSG.
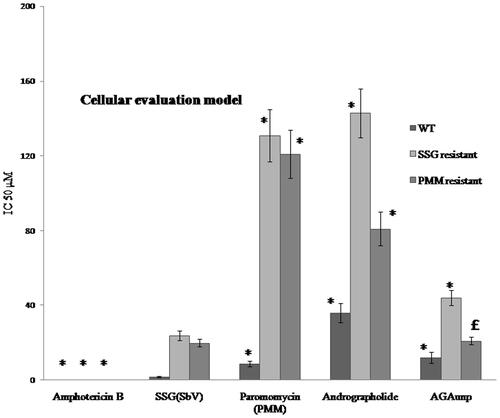
Table 1. Resistance index (RI), selectivity index (SI) and cytotoxicity of AG/AGAunp/Standard antileishmanial drugs against axenic and intracellular amastigotes for Leishmania donovani and its drug resistant cell linesTable Footnotea.
ABC transporters play a major role to generate drug resistance in Leishmania donovani. AG induced suppression of P-gp mediated drug efflux [Citation45] and failure of regular cellular mechanisms to evacuate engineered nanoparticle may be the probable factors behind the success of AGAunp’s in resistant VL conditions. The structure of AG with its lactone group resembles polyene antibiotics which might be responsible for its microbicidal properties. In addition, AG and its derivatives with α,β unsaturated γ-lactone having exo-olefin group or diene group conjugated with the lactone carbonyl are reported as potent phagocytosis inducers against M1 tumour cell line [Citation46] which further justifies its cytostatic and growth inhibitory activity against Leishmania donovani. Nano-scale gold is also reported to cause impairments in parasite oxygen metabolism [Citation47] so a possible synergism with AG may be the reason behind profound in-vitro leishmanicidal effect of AGAunp.
Macrophage uptake studies
AGAunps entry into macrophages was studied in TEM under 75 kV exposures. Macrophage internalization of AGAunp population was monitored on the basis of their time to time entry as presented in . Initial period of the study was marked by scanty AGAunp uptake as evident from TEM micrograph after 15 min exposure. Gradual increase in intracellular AGAunp population was observed with time and peak uptake occurred within 2 h of incubation (). After 2 h exposure, most of the AGAunp population internalized within the macrophages and very few remained in the extra cellular environment. No visible change of the cell wall was observed during the entire exposure time of AGAunp with macrophages. Cellular internalization of nano-scale gold is specific to its shape features and surface charge. Spherical shape nano-gold similar to AGAunp is reported to have a faster uptake compared to rod-shaped nano-gold [Citation48]. Similarly, nano-scale gold with negative surface charge like AGAunp exhibits improved macrophage internalization than those with neutral surface charge but of the same size as scavenger receptors exhibits preferential recognition of anionic sites [Citation49]. Size-directed cellular internalization, surface functionality due to AG association and ionic diffusion of nano-scale gold in culture media all act in concert to influence the macrophage uptake of AGAunp.
Conclusions
Terpenoid Andrographolide triggered facile one-pot green synthesis of zero-valent gold was achieved under sonication at a reaction temperature of 45 °C with gold SPR response at 543 nm. HRTEM, AFM and DLS experiments confirmed the uniform monodispersed spherical nature of AGAunp with a diameter of 14.004 nm. Synthesized AGAunp were crystalline in nature and effectively induced the leishmanicidal effect both in free and parasite teemed macrophage conditions. AGAunp was significantly better tolerated by normal macrophages compared to clinically accepted antileishmanial therapeutics like Amphotericin B, Sodium stibogluconate and Paramomycin. High degree of selectivity expressed by AGAunp against resistant variants of VL warrants further extensive investigations to cement their presence clinically as alternative therapeutics in VL management. This work marks the first success report of AG engineered gold nanoparticle with minimal toxicity and high degree of specificity to control drug-resistant neglected tropical disease, VL.
Das_et.al_Supplemental_Material.zip
Download Zip (1 MB)Acknowledgements
The authors would like to express gratitude to Dr. Nahid Ali and Dr. Ashish Sen (Jr.) of Indian Institute of Chemical Biology, Kolkata, India for their technical assistance in nanoparticle macrophage localization studies. Dr. Partha Roy would like to acknowledge Prof. Samit Roy, Chancellor, Adamas University for extending the University facilities for the successful completion of the work.
Disclosure statement
The authors declare no conflict of interests.
Additional information
Funding
References
- Chappuis F, Sundar S, Hailu A, et al. Visceral leishmaniasis: what are the needs for diagnosis, treatment and control. Nat Rev Microbiol. 2007;5:873–882.
- Desjeux P. Leishmaniasis: current situation and new perspectives. Comp Immunol Microbiol Infect Dis. 2004;27:305–318.
- Lukes J, Mauricio IL, Schönian G, et al. Evolutionary and geographical history of the Leishmania donovani complex with a revision of current taxonomy. Proc Natl Acad Sci USA. 2007;104:9375–9380.
- Maltezou HC. Drug resistance in visceral leishmaniasis. J Biomed Biotechnol. 2010;2010:1–8.
- Croft SL, Sundar S, Fairlamb AH. Drug resistance in leishmaniasis. Clin Microbiol Rev. 2006;19:111–126.
- García-Hernández R, Manzano JI, Castanys S, et al. Leishmania donovani develops resistance to drug combinations. PLoS Negl Trop Dis. 2012;6:e1974.
- Croft SL, Coombs GH. Leishmaniasis – current chemotherapy and recent advances in the search for novel drugs. Trends Parasitol. 2003;19:502–508.
- Dreaden EC, Mackey MA, Huang XH, et al. Beating cancer in multiple ways using nanogold. Chem Soc Rev. 2011;40:3391–3404.
- Li X, Xu H, Chen ZS, et al. Biosynthesis of nanoparticles by microorganism and their applications. J Nanomater. 2011;2011:1–16.
- Mittal AK, Chisti Y, Banerjee UC. Synthesis of metallic nanoparticles using plant extracts. Biotechnol Adv. 2013;31:346–356.
- Iravani S. Green synthesis of metal nanoparticles using plants. Green Chem. 2011;13:2638–2650.
- Castro L, Blazquez ML, Munoz JA, et al. Biosynthesis of gold nanowires using sugar beet pulp. Proc Biochem. 2011;46:1076–1082.
- Chandran SP, Chaudhary M, Pasricha R, et al. Synthesis of gold nanotriangles and silver nanoparticles using Aloe vera plant extract. Biotechnol Prog. 2006;22:577–583.
- Song JY, Jang HK, Kim BS. Biological synthesis of gold nanoparticles using Magnolia kobus and Diopyros kaki leaf extracts. Proc Biochem. 2009;44:1133–1138.
- Armendariz V, Herrera I, Peralta–Videa JR, et al. Size controlled gold nanoparticle formation by Avena sativa biomass: use of plants in nanobiotechnology. J Nanopart Res. 2004;6:377–382.
- Sazgarnia A, Taheri AR, Soudmand S, et al. Antiparasitic effects of gold nanoparticles with microwave radiation on promastigots and amastigotes of Leishmania major. Int J Hyperthermia. 2013;29:79–86.
- Sattarahmady N, Movahedpour A, Heli H, et al. Gold nanoparticles-based biosensing of Leishmania major kDNA genome: visual and spectrophotometric detections. Sens Actuators B. 2016;235:723–731.
- Polonio T, Efferth T. Leishmaniasis: drug resistance and natural products (review). Int J Mol Med. 2008;22:277–286.
- Ogungbe IV, Setzer WN. In-silico Leishmania target selectivity of antiparasitic terpenoids. Molecules. 2013;18:7761–7847.
- Sinha J, Mukhopadhyay S, Das N, et al. Targeting of liposomal andrographolide to L. donovani-infected macrophages in vivo. Drug Deliv. 2000;7:209–213.
- Roy P, Das S, Bera T, et al. Andrographolide nanoparticles in leishmaniasis: characterization and in vitro evaluations. Int J Nanomedicine. 2010;5:1113–1121.
- Das S, Roy P, Mondal S, et al. One pot synthesis of gold nanoparticles and application in chemotherapy of wild and resistant type visceral leishmaniasis. Colloids Surf B Biointerfaces. 2013;107:27–34.
- Debrabant A, Joshi MB, Pimenta PF, et al. Generation of Leishmania donovani axenic amastigotes: their growth and biological characteristics. Int J Parasitol. 2004;34:205–217.
- Senero D, Lemesra JL. Axenically cultured amastigote forms as an in-vitro model for investigation of antileishmanial agents. Antimicrob Agents Chemother. 1997;41:972–976.
- Ghosh S, Kar N, Bera T. Oleanolic acid loaded poly lactic co- glycolic acid- vitamin E TPGS nanoparticles for the treatment of Leishmania donovani infected visceral leishmaniasis. Int J Biol Macromol. 2016;93:961–970.
- Callahan H, Kelley C, Peretra T, et al. Microtubule inhibitors: structure-activity analysis suggest rational models to identify potentially active compounds. Antimicrob Agents Chemother. 1996;40:947–952.
- Mondal S, Roy P, Das S, et al. In vitro susceptibilities of wild and drug resistant Leishmania donovani amastigote stages to andrographolide nanoparticle: role of vitamin E derivative TPGS for nanoparticle efficacy. PLoS One. 2013;8:e81492.
- Nwaka S, Hudson A. Innovative lead discovery strategies for tropical diseases. Nat Rev Drug Discov. 2006;5:941–955.
- Nativo P, Prior IA, Brust M. Uptake and intracellular fate of surface-modified gold nanoparticles. ACS Nano. 2008;2:1639–1644.
- Kimling J, Maier M, Okenve B, et al. Turkevich method for gold nanoparticle synthesis revisited. J Phys Chem B. 2006;110:15700–15707.
- Thakor AS, Jokerst J, Zavaleta C, et al. Gold nanoparticles: a revival in precious metal administration to patients. Nano Lett. 2011;11:4029–4036.
- Perrault SD, Chan WC. Synthesis and surface modification of highly monodispersed, spherical gold nanoparticles of 50 − 200 nm. J Am Chem Soc. 2009;131:17042–17043.
- Frens G. Controlled nucleation for the regulation of the particle size inmonodisperse gold suspensions. Nat Phys Sci. 1973;241:20–22.
- Jana NR. Gram-scale synthesis of soluble, near-monodisperse gold nanorods and other anisotropic nanoparticles. Small. 2005;1:875–882.
- Daniel MC, Astruc D. Gold nanoparticles: assembly, supramolecular chemistry, quantum-size-related properties, and applications toward biology, catalysis, and nanotechnology. Chem Rev. 2004;104:293–346.
- Akowuah GA, Zhari I, Norhayati I, et al. HPLC and HPTLC densitometric determination of andrographolides and antioxidant potential of Andrographis paniculata. J Food Comp Anal. 2006;19:118–126.
- Suslick KS. Sonochemistry. Science. 1990;247:1439–1445.
- Srinath BS, Ravishankar Rai V. Biosynthesis of highly monodispersed, spherical gold nanoparticles of size 4–10 nm from spent cultures of Klebsiella pneumonia. 3 Biotech. 2015;5:671–676.
- Tam NC, Scott BM, Voicu BC, et al. Facile synthesis of Raman active phospholipid gold nanoparticles. Bioconjug Chem. 2010;21:2178–2182.
- Bellamy LJ. 1980. The Infrared Spectra of Complex Molecules. New York (NY): Chapman and Hall publishing.
- Cava MP, Chan WR, Haynes LJ, et al. The structure of andrographolide. Tetrahedron. 1962;18:397–403.
- Jebali A, Kazemi B. Nano-based antileishmanial agents: a toxicological study on nanoparticles for future treatment of cutaneous leishmaniasis. Toxicol in Vitro. 2013;27:1896–1904.
- Camargos HS, Moreira RA, Mendanha SA, et al. Terpenes increase the lipid dynamics in the leishmania plasma membrane at concentrations similar to their IC50 values. PLoS One. 2014;9:e104429.
- Lala S, Pramanik S, Mukhopadhay S, et al. Harmine: evaluation of its antileishmanial properties in various delivery systems. J Drug Target. 2004;12:165–174.
- Srivalli KMR, Lakshmi PK. Overview of P-glycoprotein inhibitors: a rational outlook. Braz J Pharm Sci. 2012;48:353–368.
- Matsuda T, Kuroyanagi M, Sugiyama S, et al. Cell differentiation-inducing diterpenes from Andrographis paniculata Nees. Chem Pharm Bull. 1994;42:1216–1225.
- Grumezescu AM. Nano- and Microscale Drug Delivery Systems: design and Fabrication. Amsterdam (The Netherlands): Elsevier. 2017.
- Chithrani BD, Ghazani AA, Chan WC. Determining the size and shape dependence of gold nanoparticle uptake into mammalian cells. Nano Lett. 2006;6:662–668.
- Arnida, Janát-Amsbury MM, Ray A, et al. Geometry and surface characteristics of gold nanoparticles influence their biodistribution and uptake by macrophages. Eur J Pharm Biopharm. 2011;77:417–423.