Abstract
The cationic dimethyldioctadecylammonium/trehalose 6,6,9-dibehenate (DDA/TDB) liposome is as a strong adjuvant system for vaccines, with remarkable immunostimulatory activity. The mucosal administration of vaccines is a potential strategy for inducing earlier and stronger mucosal immune responses to infectious diseases. In this study, we assessed whether the intranasal administration of cationic DDA/TDB liposomes combined with influenza antigen A (H3N2) can be used as a highly efficacious vaccine to induce mucosal and systemic antibody responses. Confocal laser scanning microscopy and a flow-cytometric analysis showed that the uptake of the cationic DDA/TDB liposome carrier was significantly higher than that of neutral 1,2-distearoyl-sn-glycero-3-phosphocholine/cholesterol (DSPC/Chol) or cationic 1,2-dioleoyl-3-trimethylammonium-propane/3β-(N-[N′,N′-dimethylaminoethane]-carbamoyl (DOTAP/DC-Chol) liposomes. Our results indicate that the cationic DDA/TDB liposome is more effective in facilitating its uptake by dendritic cells (DCs) in vitro than the DSPC/Chol or DOTAP/DC-Chol liposome. DCs treated with DDA/TDB liposomes strongly expressed CD80, CD86, and MHC II molecules, whereas those treated with DSPC/Chol or DOTAP/DC-Chol liposomes did not. C57BL/6 mice intranasally immunized with H3N2-encapsulating cationic DDA/TDB liposomes had significantly higher H3N2-specific s-IgA levels in their nasal wash fluid than those treated with other formulations. The DDA/TDB liposomes also simultaneously enhanced the serum IgG IgG2a, IgG1, and IgG2b antibody responses. In summary, DDA/TDB liposomes effectively facilitated their uptake by DCs and DCs maturation in vitro, and induced significantly higher mucosal IgA, systemic IgG, IgG1, and IgG2b antibody titres than other formulations after their intranasal administration in vivo. These results indicate that DDA/TDB liposomes are a promising antigen delivery carrier for clinical antiviral applications.
Introduction
Vaccination is a crucial approach to preventing illness and death caused by infectious diseases. In recent years, subunit vaccines, based on purified or synthetic antigens, have shown significantly improved safety profiles compared with those of traditionally prepared vaccines [Citation1,Citation2]. However, subunit vaccines are weakly immunogenic by themselves [Citation3]. Adjuvants based on immunopotentiators and/or delivery systems are crucial components of vaccines and they are extremely effective in potentiating the immunological responses to vaccines [Citation4,Citation5]. Therefore, the co-administration of the appropriate adjuvant is essential, especially for subunit vaccines [Citation2,Citation6].
Liposomes are important delivery carriers for vaccines because of their excellent versatility, which allows them to transport various kinds of antigens [Citation7–9]. Previous studies have suggested that the adjuvanticity of cationic liposomes is greater than that of neutral liposomes [Citation10–12]. In many studies, the cationic liposomal adjuvant system composed of dimethyldioctadecylammonium (DDA) and the immunostimulator trehalose 6,6-dibehenate (TDB) has been described as having immunostimulatory properties [Citation3,Citation5,Citation13–15]. This adjuvant displays exceptional immunostimulatory activity because it causes deposition of the antigen and induces local inflammation at the site of injection, ultimately generating strong humoral and cell-mediated immune responses [Citation15–19]. Therefore, the DDA/TDB liposome is probably an ideal adjuvant for inducing effective immunity.
The route of immunostimulation is one of the key factors affecting the immune response. The mucosal surfaces, including those of the respiratory and gastrointestinal tracts, are the main routes of entry for most infectious agents [Citation20,Citation21]. The appropriate delivery of vaccines to mucosal sites has the advantage of inducing multiple immunological barriers, both at the mucosal effector sites and in the systemic compartment, via a non-invasive route [Citation22]. Interestingly, the nasal mucosa is considered a feasible route for vaccinations that excite both mucosal and systemic immune responses, often via the nasopharynx-associated lymphoid tissue (NALT) [Citation23]. The NALT contains specialized M-like cells, as well as large numbers of B cells, T cells, and antigen-presenting cells (APCs), such as dendritic cells (DCs), which are required for the initiation and regulation of antigen-specific immune responses [Citation24,Citation25].
Luminescent semiconductor nanocrystals, also known as quantum dots (QDs), have many advantages over organic dyes, including their broad absorption spectra together with their narrow emission spectra, high photostability, and excellent resistance to degradation by chemicals. These unique optical properties make QDs useful for multiplexing applications with biological labels, sensors, laser materials, and thin-film light-emitting devices [Citation26,Citation27]. It has been proposed that liposomes can be used as carriers to load considerable amounts of both QDs and antigens, for use as a biological label to visualize the internalization of drugs by cells, and an adjuvant for simultaneously inducing effective immunity ().
Figure 1. Structure of theranostic liposomes (schematic). Combination of antigens and hydrophobic QDs with DDA/TDB liposomal encapsulation.
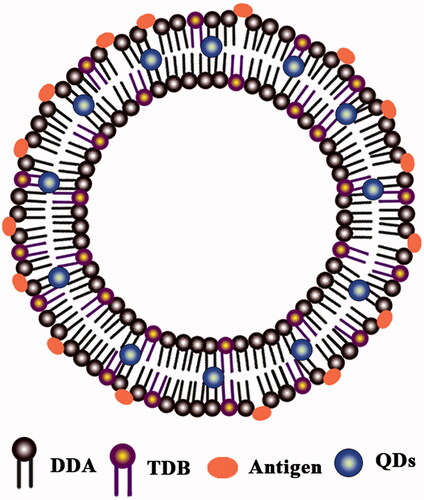
However, few studies have investigated both the effects of cationic DDA/TDB liposomes on DCs in vitro and as mucosal vaccine adjuvants administered via the intranasal route in vivo. The aim of this study was to study the potential utility of DDA/TDB liposomes as an adjuvant system for vaccines administered intranasally, to induce both mucosal and systemic immune responses. The influenza antigen (H3N2) used as the model antigen and the QDs used as the model imaging agent were formulated in cationic DDA/TDB liposomes. To better understand the mucosal adjuvant effects of the cationic DDA/TDB liposomes, we investigated the uptake of the QD-labeled liposome vaccine formulations by DCs and their stimulation of mouse DCs in vitro. The mucosal and humoural antibody titres were determined with enzyme-linked immunosorbent assays (ELISAs) to assess the mucosal and systemic immune responses.
Materials and methods
Materials
1,2-Distearoyl-sn-glycero-3-phosphocholine (DSPC), dimethyldioctadecylammonium bromide (DDA), 1,2-dioleoyl-3-trimethylammonium-propane (DOTAP), β-(N-[N′,N′-dimethylaminoethane]-carbamoyl) (DC-Chol), and trehalose 6,6′-dibehenate (TDB) were purchased from Avanti Polar Lipids, Inc. (Alabaster, AL). Cholesterol and all organic solvents were purchased from Sigma-Aldrich (Dorset, UK), and the organic solvents were of either analytical or chromatographic grade. Influenza antigen A/Jiangsu (H3N2) was kindly supplied by the Chinese Centre for Disease Control and Prevention (Beijing, China). The organic QDs (QD655 ITK-carboxyl), fetal bovine serum (FBS) and RPMI 1640 medium were purchased from Life Technologies (Carlsbad, CA).
Preparation and characterization of liposomes
The liposomes were prepared with the previously described lipid-film hydration method [Citation19,Citation28]. Briefly, 4.46 μmol of total lipids (DSPC:Chol in 1:1molar ratio, DDA:TDB in 8:1 molar ratio) were dissolved in chloroform:methanol (9:1, by volume). After the thin lipid films formed, the organic solvent was removed by rotary evaporation and then flushing with N2. The lipid films were hydrated in Tris buffer (10 mM, pH 7.4) for 20 min at 60 °C. The H3N2 antigen was added after the liposomes had formed, at a final concentration of 75 μg/ml. The antigen encapsulation efficiency (EE) was determined by measuring the non-associated antigen. The non-associated antigen was separated from the liposomes by high-speed centrifugation, and the quantity of non-associated antigen was measured with a Pierce BCA Protein Assay Kit (Thermo Fisher Scientific, Waltham, MA). EE was then calculated with the equation: EE = ([total antigen − non-associated antigen]/total antigen) × 100%. The particle size and the zeta potential of the liposomes were measured with Nicomp™ 380 ZLS Particle Sizer (Santa Barbara, CA). Transmission electron microscopy (TEM) measurements were made with an H-7650 instrument (Hitachi, Tokyo, Japan) at an accelerating voltage of 80 kV.
QD-labelled liposomes were prepared to investigate the effect of liposome uptake on DCs by adding 0.01 mol% QDs (ratio of total lipid moles) to the thin lipid film before it was hydrated. Any free QDs and QD aggregates were removed by centrifugation.
Preparation of bone-marrow-derived DCs (BMDCs)
Mouse BMDCs were prepared as previously described [Citation29], with minor modifications. Bone-marrow cells were separated from the femurs and tibias of C57BL/6 mice (6–8 weeks old) from the Jinfeng Laboratory Animal Jinan Co., Ltd (Jinan, China). After several rounds of centrifugation, washing, and counting, the remaining cells were cultured in RPMI 1640 medium containing 10% heat-inactivated foetal bovine serum, 100 U/ml penicillin, and 100 μg/ml− streptomycin, which was supplemented with granulocyte macrophage colony-stimulating factor (40 ng/ml) and interleukin 4 (40 ng/ml), at 37 °C in 5% CO2 for 6 d to generate immature BMDCs. The ratio of CD11c+ cells was determined with flow cytometry (Bio-Rad, Hercules, CA), and the cells (final proportion of CD11c+ cells >70%) were used for subsequent in vitro experiments. Cell numbers were adjusted to about 1 × 106 cells/ml.
In vitro cellular uptake assay
Mouse BMDCs were cocultured with various QD-labelled liposome formulations (with the same fluorescence intensity) for 2 h. After 2 h, an aliquot of the cells was washed three times with phosphate-buffered saline (PBS) and fixed with 4% paraformaldehyde for 5 min. The cells were then washed twice with PBS. The nuclei were stained by incubation with 4′,6-diamidino-2-phenylindole (DAPI) for 10 min. For a qualitative study, the cells were washed three times with PBS and observed with confocal laser scanning microscopy (Nikon Corporation, Tokyo, Japan) with the imaging software NIS-Element AR 3.0 (SPSS, Chicago, IL). Another aliquot of the cells was washed three times with PBS and examined immediately with flow cytometry in a quantitative study. The cell numbers were adjusted to about 1 × 106 cells/ml.
Maturation assay
A 24-well culture plate was used to coculture the mouse BMDCs (106 cells per well) with the different formulations (H3N2, neutral DSPC/Chol liposomes, cationic DOTAP/DC-Chol or DDA/TDB liposomes). After incubation for 24 and 48 h, the cells were stained with fluorescein isothiocyanate (FITC)-labelled anti-mouse CD80 monoclonal antibody, phycoerythrin (PE)-labelled anti-mouse CD86 monoclonal antibody, and PE-labelled anti-mouse MHCII monoclonal antibody (BD Biosciences, Franklin Lakes, NJ). Cell fluorescence was measured with flow cytometry.
Immunization procedures
Five groups of six female C57BL/6 mice (6 − 8 weeks of age) were immunized intranasally with 50 μl of DSPC/Chol liposomes (1.785 mg of lipid) containing 25 μg of H3N2, 50 μl of DOTAP/DC-Chol liposomes (0.471 mg of lipid) containing 25 μg of H3N2, 50 μl of DDA/TDB liposomes (0.294 mg of lipid) containing 25 μg of H3N2, or 50 μl of H3N2 alone (25 μg). The control groups received 50 μl of PBS. Each mouse was immunized twice, with a 2-week interval between immunizations. Two weeks after the final vaccination, blood samples were taken. The serum samples were separated by centrifugation at 1200 × g for 30 min at room temperature.
To analyze the induced antigen-specific IgA in the nasal washes, nasal wash samples were collected. Immediately thereafter, the mice were killed by cervical dislocation, as previously described [Citation30].
Analysis of specific anti-H3N2 antibodies
The H3N2-specific IgG, IgA, IgG2a, IgG1, and IgG2b titres in the collected samples were determined with ELISA. The wells of a MaxiSorp™ 96-well ELISA plate (Agilent Technologies, Palo Alto, CA) were each coated with 100 μl of H3N2 (2 μg/ml) in 0.1 M carbonate buffer (pH 9.6) and incubated at 4 °C overnight. The plates were washed with 0.02 M PBS containing 0.05% Tween 20 (PBST) and blocked with 200 μl of 2% BSA in PBST. The sera and nasal wash samples were serially diluted two-fold and added to the plates, which were incubated for 1 h at 37 °C. After the plates were washed three times, the wells were treated with horseradish peroxidase (HRP)-conjugated goat anti-mouse IgG IgG2a, IgG1, IgG2b, and IgA antibodies (Bio-Rad, Hercules, CA) for 1 h at 37 °C to detect of the bound anti-mouse IgG IgG2a, IgG1, IgG2b, and IgA. The plates were developed with the TMB substrate. The reactions were stopped with the addition of 2 M H2SO4. After 20 min, the optical density (OD) of the wells was measured with a microplate reader (Bio-Rad, Hercules, CA) at 450 nm. The antibody titre was defined as the reciprocal of the highest dilution in which the OD was two-fold higher than the mean OD of the control samples.
Statistical analysis
Data were analyzed with Student’s t test or analysis of variance (ANOVA). p Values < .05 were deemed statistically significant. Values are reported as means ± standard deviations (SD), and error bars are used to represent SD.
Results
Physicochemical characteristics of liposomal adjuvants
The liposomes were prepared with the thin-film hydration method and H3N2 was entrapped within each liposomal formulation. The physicochemical properties of the liposomes prepared in this study were evaluated, and are shown in and . The particle sizes of DSPC/Chol liposomes, DOTAP/DC-Chol liposomes, and DDA/TDB liposomes were 480.9 ± 21.3, 339.0 ± 3.9, and 1920.0 ± 70.0 nm, respectively. A polydispersity analysis of the liposomes showed that the DDA/TDB liposomes had a quite narrow size distribution. The liposome zeta potentials indicated a more cationic charge on the surfaces of the DDA/TDB liposomes than on those of the DSPC/Chol liposomes or DOTAP/DC-Chol liposomes. As shown in , the H3N2 encapsulation efficiency of the DDA/TDB liposomes was greater than those of the DSPC/Chol and DOTAP/DC-Chol liposomes. This may be attributable to the greater amount of antigen on the surfaces of the DDA/TDB liposomes, increasing the size of the liposomes.
Figure 2. TEM of various liposomal formulations: (a) DSPC/Chol liposome, (b) DOTAP/DC-Chol liposome, and (c) DDA/TDB liposome influenza vaccines.
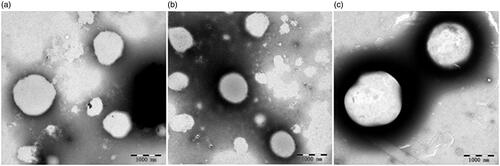
Table 1. Physicochemical characteristics of various liposomal formulations.
Uptake of liposomal adjuvants by DCs and flow cytometry
Because the uptake and presentation of antigen by DCs is very important, we used BMDCs to estimate the uptake of the different liposomes in vitro. First, we used CLSM to visualize the uptake of the different liposomal formulations by BMDCs after their incubation with QD-labelled liposomes, in a qualitative study. To better compare the red fluorescence intensity of the QD among the BMDCs treated with the three types of liposomes, the images were taken with the same imaging parameters (sensitivity, gain, and offset), while maintaining the laser power constant throughout the cell imaging process. As shown in , the QD fluorescent signals were observed after 2 h of coculture with the DDA/TDB liposomes. The liposomes were labelled with QDs (red) and the nuclei of the BMDCs were labelled with DAPI (blue). The red fluorescence intensity in the mouse BMDCs containing the cationic DDA/TDB liposomes () was higher (more red staining of the cytoplasm) than the intensity of those containing DOTAP/DC-Chol or DSPC/Chol liposomes ().
Figure 3. Confocal laser scanning microscopy (CLSM) images using BMDCs cells after 2 h incubation with the PBS as the control group (a), DSPC/Chol liposome (b), DOTAP/DC-Chol liposome (c), and DDA-TDB liposome (d). Column (I): DAPI channels showing the blue fluorescence from DAPI stained nuclei, (II) quantum dots showing the red fluorescence from liposomes distributed in cytoplasm, and (III): merged channels of quantum dots and DAPI. Scale bars equal 40 μm.
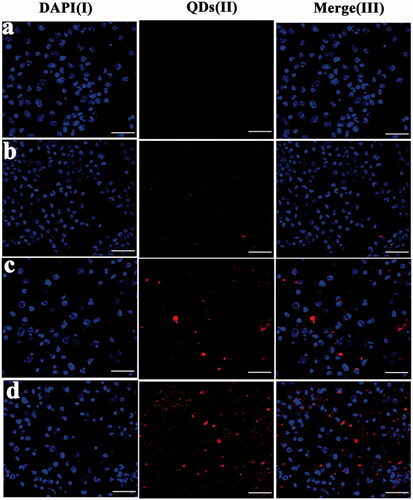
Flow cytometry was used to evaluate the uptake of the different liposomal formulations by BMDCs in a quantitative study. The flow cytometric results in show that 32.73 ± 2.6% of mouse BMDCs treated with DDA/TDB liposomes were positive for QDs, whereas only 12.23 ± 1.0% and 4.9 ± 1.8% of the cells treated with DOTAP/DC-Chol and DSPC/Chol liposomes were positive for QDs. These results demonstrate that liposomes uptake by BMDCs was significantly greater for DDA/TDB liposomes.
Figure 4. BMDCs were cultured with various QDs-labelled liposome formulations for 2 h. The uptake of QDs-labelled liposome was measured using flowcytometry. (A) Percentages of intracellular uptake of test formulations. (B) Flow cytometry pictures are representatives of the control group (a), DSPC/Chol liposome (b), DOTAP/DC-Chol liposome (c), and DDA-TDB liposome (d). (n = 3) *p < .05, **p < .01.
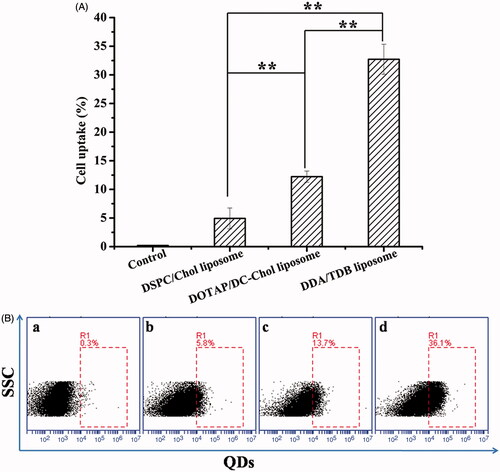
Effects of liposomal adjuvants on the maturation of DCs in vitro
To determine the effects of the different liposomal formulations on DCs maturation, we evaluated the increases in MHCII, CD80, and CD86 levels on the DCs 24 and 48 h after stimulation with the liposome formulations. As shown in , after coincubation for 24 h, the BMDCs treated with H3N2 alone or H3N2 combined with the various liposomes showed no difference in the expression of CD80, MHC-II, or CD86. However, after coincubation for 48 h, the BMDCs treated with H3N2 encapsulated in the DDA/TDB liposomes displayed significantly higher levels of the CD80, MHC-II, and CD86 markers on their surfaces than those treated with H3N2 alone or complexed with the DOTAP/DC-Chol or DSPC/Chol liposomes. This result indicates that the effective uptake of the antigen by DCs and the maturation of the DCs were both closely associated with the presence of the DDA/TDB liposomes, which induced subsequent immune responses.
Figure 5. BMDCs were cultured with medium (control), antigen, or different liposome formulations for 48 h. The expression of CD80, CD86, and MHCII was measured using flow cytometry (a–c). *Significant difference (p < .05) between cells receiving various liposome vaccines and those H3N2 alone; #significant difference (p < .05) between cells receiving DDA-TBD liposomes and those receiving DSPC-Chol liposomes or DOTAP-DC-Chol liposomes (n = 3).
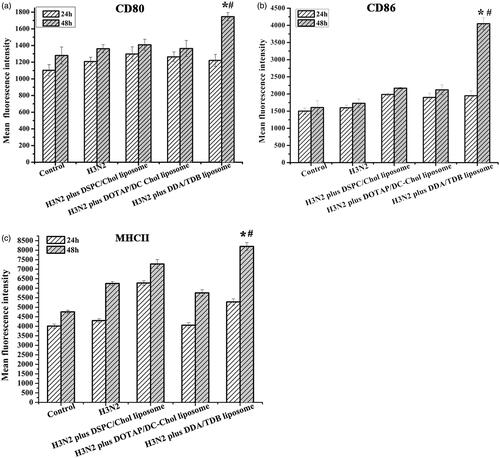
Antibody titre analysis
We investigated whether the intranasal administration of DDA/TDB liposomes as an adjuvant induced both mucosal and systemic H3N2-specific antibody responses in mice by testing the nasal washes collected 2 weeks after the mice were immunized with H3N2 alone or complexed with the different adjuvant formulations (DSPC/Chol liposomes, DOTAP/DC-Chol liposomes, or DDA/TDB liposomes). As shown in , a significant anti-H3N2 secretory IgA (s-IgA) antibody response was elicited by the intranasal administration of the DDA/TDB liposomes. It was significantly higher than the responses induced in the DOTAP/DC-Chol or DSPC/Chol liposome group (p < .05). The intranasal delivery of H3N2 alone did not improve the H3N2-specific IgG response compared with that induced by the delivery of the liposomal formulations (). However, the levels of H3N2-specific IgG antibodies were significantly higher after vaccination with the H3N2-complexed DDA/TDB liposomes than after vaccination with the H3N2-complexed DOTAP/DC-Chol or DSPC/Chol liposomes. These data suggest that the efficient delivery of the antigen to DCs induced potent mucosal and humoural immune responses.
Figure 6. Effect of liposomes on the H3N2-specific antibody response. C57BL/6 mice were given various liposome formulations. One week after the final administration, the anti-H3N2 IgG and anti-H3N2 IgA level in nasal washes were detected by ELISA assay. The antibody titre was defined as the reciprocal of the highest dilution that had an OD value that was two times higher than the mean OD of control mice. *Significant difference (p < .05) between mice receiving various liposome vaccines and those H3N2 alone; #significant difference (p < .05) between mice receiving DDA-TBD liposomes and those receiving DSPC-Chol liposomes or DOTAP-DC-Chol liposomes. Mean ± SD (n = 6).
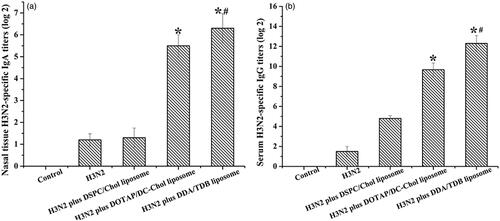
The IgG1, IgG2a, and IgG2b responses induced in this study are shown in . By day 28, a significantly (p < .05) higher IgG1 antibody response was produced with the DDA/TDB formulation than in mice immunized with the free antigen or the other liposomal formulations (). Furthermore, the mice immunized with the cationic DDA/TDB liposomes produced better IgG2b responses than the mice immunized with the free antigen (). However, the IgG2a responses induced by the various liposome formulations did not differ significantly.
Figure 7. Specific anti-H3N2 serum antibody levels serum collected from mice immunized with liposome influenza vaccine. Samples were collected at weeks 4. *Significant difference (p < .05) between mice receiving various liposome vaccines and those H3N2 alone; #significant difference (p < .05) between mice receiving DDA-TBD liposomes and those receiving DSPC-Chol liposomes or DOTAP-DC-Chol liposomes. Mean ± SD (n = 6).
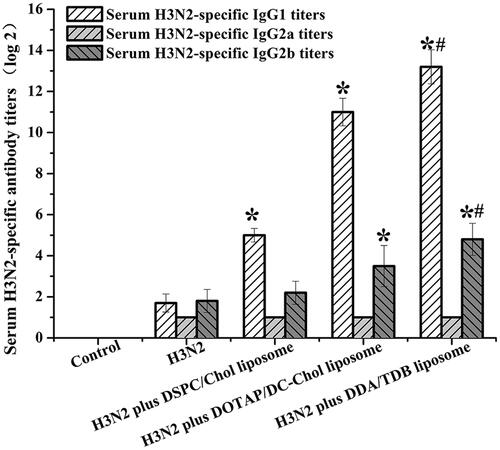
Discussion
Vaccination is one of the most potent approaches to the prevention of fatal infectious diseases. In this context, it is essential to induce both mucosal and systemic immune responses against the original infection and subsequent infections. Therefore, the development of safe and effective adjuvants for vaccine therapies is a major goal of immunological research. In this study, we investigated the effects of cationic DDA/TDB liposomes on DCs in vitro and as a mucosal vaccine adjuvant for the influenza antigen when administered via the intranasal route in vivo.
Physicochemical factors, such as the positive charge on the liposomes, play crucial roles in their usefulness [Citation31]. Our results showed that the zeta potentials of the DDA/TDB and DOTAP/DC-Chol liposomal formulations were approximately 50 mV, whereas the zeta potential of the DSPC/Chol liposomal formulation was approximately −4 mV (). The surficial charge has a significant effect on the ability of formulations to cross mucus, and cationic formulations display enhanced deposition and antigen penetration in the tracheobronchial region. This may be more important in inducing mucosal immune responses than the epithelial permeation capacity of the antigen alone [Citation11,Citation32,Citation33]. The particle sizes of the formulations differed, and the effect of particle size on the immune responses induced warrants further study.
Upon the administration of a vaccine, the adjuvant and antigen must be taken up by APCs, which then present the antigen to the T cells to stimulate immune activation [Citation34]. Among the APCs, DCs play crucial roles in antigen uptake and the subsequent priming of naïve T cells [Citation23,Citation35,Citation36]. To investigate the capacity of liposomes to enhance their uptake by DCs, BMDCs were treated with the various liposome formulations. The BMDCs took up the cationic DDA/TDB and DOTAP/DC-Chol liposomes more effectively than the neutral DSPC/Chol liposomes after coculture for 2 h ( and ), suggesting that the positive charge on the surfaces of liposomes promote their uptake. The BMDCs also took up the cationic DDA/TDB liposomes more effectively than the DOTAP/DC-Chol liposomes. The toxicity of the DOTAP/DC-Chol liposomes may limit their uptake, and TDB may activate APCs via a specific intracellular signalling system. Other studies have suggested that the uptake of cationic DDA liposomes by APCs, to deliver the associated antigen to cells, is facilitated by electrostatic interactions immediately upon the liposome contacting the cell surface, which activates antigen uptake and presentation. This may be a crucial factor behind the adjuvant properties of cationic DDA liposomes [Citation19,Citation33]. Low doses of the DDA/TDB liposomes easily delivered the antigen to DCs. Therefore, cationic DDA/TDB liposomes have greater potential utility in inducing more-potent immune responses than conventional liposomes.
We also examined the effect of liposomes on DC maturation. DCs are considered the most efficient APCs, and play crucial roles in both the innate and adaptive immune responses. Aberrant increases in the expression of the CD80, CD86, and MHC molecules tend to be a marker of DC maturation. Many studies have demonstrated that liposomes promote the delivery of antigens to DCs. In this study, BMDCs treated with DDA/TDB liposomes showed higher expression of MHC II, CD80, and CD86 than those treated with DSPC/Chol or DOTAP/DC-Chol liposomes (). The presence of DDA/TDB liposomes in this delivery system was responsible for the maturation of the DCs. According to our in vitro results, DDA/TDB liposomes induce both better DC maturation and better antigen uptake than other liposomes, which may explain the higher antibody titres they induced in this study.
A very prompt immune response occurs in mammals after infection with influenza virus, which can clear the invading virus within 5–7 d [Citation37]. Antibodies directed against the virus can be detected during the first week of infection, with IgA and IgM present in the nasal mucus and blood, respectively [Citation38].
The intranasal immunity, particularly in the NALT, has a great capacity to defend against pathogens that infect the organism via the mucosa [Citation39]. The NALT consists of follicle-associated epithelium containing antigen-sampling M cells, as well as large numbers of APCs, B cells, and T cells, which favour the production of s-IgA [Citation21]. In the present study, the DDA/TDB liposomes enhanced the production of mucosal IgA antibodies after intranasal immunization, thus offering more cross-protection against viral infection than is afforded by the systemic immunity [Citation38].
The DDA/TDB liposomes also potentiated the production of serum IgG, IgG1, and IgG2b antibodies and facilitated a more potent response than the influenza antigen alone or the antigen complexed with the DSPC/Chol or DOTAP/DC-Chol liposomes ( and ). This suggests that DDA/TDB liposomes potentiate a stronger humoural immune response and a Th2-type antibody profile, which may be attributable to their capacity to increase antigen uptake and promote DC maturation. Evidence from in vitro and in vivo systems has suggested that the combination of an effective delivery system (DDA) [Citation33] and a potential immunomodulator (TDB) [Citation40] induces a potent immune response. DDA-based liposomes also form an “antigen depot” at the administration site, which significantly affects the long-term memory response [Citation16]. TDB is a synthetic immunomodulator that signals through the SYK–CARD9 pathway to activate APCs, thereby inducing Th responses [Citation41,Citation42]. These distinct characteristics of DDA/TDB liposomes may be highly relevant to their induction of a potent immune response. In brief, our results suggest that DDA/TDB liposomes are more efficaciously taken up by DCs in vitro than other liposomes, thus causing the DCs to mature and move into the systemic circulation, which induces both mucosal and systemic immune responses, even after intranasal immunization. Therefore, intranasal vaccination with DDA/TDB liposomes could confer a more efficient protective effect than other carriers in combating infectious diseases.
Conclusions
In this study, DDA/TDB liposomes efficiently potentiated their uptake by DCs and DCs maturation in vitro. DDA/TDB liposomes provide needle-free vaccine delivery and produce stronger antigen-specific antibody responses in both the mucosal and systemic circulations after intranasal vaccination than other formulations. Therefore, DDA/TDB liposomes have potential utility as a novel carrier for vaccine delivery and for specific indications, including cancer vaccines.
Disclosure statement
The authors state no conflict of interest and have received no payment in preparation of this manuscript.
Additional information
Funding
References
- Rappuoli R. Reverse vaccinology. Curr Opin Microbiol. 2000;3:445–450.
- Rappuoli R, Mandl CW, Black S, et al. Vaccines for the twenty-first century society. Nat Rev Immunol. 2011;11:865–872.
- McNeil SE, Rosenkrands I, Agger EM, et al. Subunit vaccines: distearoylphosphatidylcholine-based liposomes entrapping antigen of fer a neutral alternative to dimethyldioctadecylammonium-based cationic liposomes as an adjuvant delivery system. J Pharm Sci. 2011;100:1856–1865.
- Amorij JP, Kersten GF, Saluja V, et al. Towards tailored vaccine delivery: needs, challenges and perspectives. J Control Release. 2012;161:363–376.
- Lindblad EB, Elhay MJ, Silva R, et al. Adjuvant modulation of immune responses to tuberculosis subunit vaccines. Infect Immun. 1997;65:623–629.
- Bhavsar MD, Amiji MM. Development of novel biodegradable polymeric nanoparticles-in-microsphere formulation for local plasmid DNA delivery in the gastrointestinal tract. AAPS PharmSciTech. 2008;9:288–294.
- Schwendener RA. Liposomes as vaccine delivery systems: a review of the recent advances. Ther Adv Vaccines. 2014;2:159–182.
- Kakhi Z, Frisch B, Heurtault B, et al. Liposomal constructs for antitumoral vaccination by the nasal route. Biochimie. 2016;130:14–22.
- Tyagi RK, Garg NK, Jadon R, et al. Elastic liposome-mediated transdermal immunization enhanced the immunogenicity of P. falciparum surface antigen, MSP-119. Vaccine. 2015;33:4630–4638.
- Tada R, Hidaka A, Iwase N, et al. Intranasal immunization with DOTAP cationic liposomes combined with DC-cholesterol induces potent antigen-specific mucosal and systemic immune responses in mice. PLoS One. 2015;10:e0139785.
- Christensen D, Korsholm KS, Andersen P, et al. Cationic liposomes as vaccine adjuvants. Expert Rev Vaccines. 2011;10:513–521.
- Tada R, Muto S, Iwata T, et al. Attachment of class B CpG ODN onto DOTAP/DC-chol liposome in nasal vaccine formulations augments antigen-specific immune responses in mice. BMC Res Notes. 2017;10:68.
- Andersen P. Effective vaccination of mice against Mycobacterium tuberculosis infection with a soluble mixture of secreted mycobacterial proteins. Infect Immunity. 1994;62:2536–2544.
- Lemaire G, Tenu J-P, Petit J-F. Natural and synthetic trehalose diesters as immunomodulators. Med Res Rev. 1986;6:243–274.
- Kirby DJ, Kaur R, Agger EM, et al. Developing solid particulate vaccine adjuvants – surface bound antigen favouring a humoural response, whereas entrapped antigen shows a tendency for cell mediated immunity. Cdd. 2013;10:268–278.
- Henriksen-Lacey M, Bramwell VW, Christensen D, et al. Liposomes based on dimethyldioctadecylammonium promote a depot effect and enhance immunogenicity of soluble antigen. J Control Release. 2010;142:180–186.
- Korsholm KS, Petersen RV, Agger EM, et al. T-helper 1 and T-helper 2 adjuvants induce distinct differences in the magnitude, quality and kinetics of the early inflammatory response at the site of injection. Immunology. 2009;129:75–86.
- Henriksen-Lacey M, Devitt A, Perrie Y. The vesicle size of DDA:TDB liposomal adjuvants plays a role in the cell-mediated immune response but has no significant effect on antibody production. J Control Release. 2011;154:131–137.
- Kaur R, Henriksen-Lacey M, Wilkhu J, et al. Effect of incorporating cholesterol into DDA:TDB liposomal adjuvants on bilayer properties, biodistribution, and immune responses. Mol Pharm. 2014;11:197–207.
- Gebert A, Steinmetz I, Fassbender S, et al. Antigen transport into Peyer's patches: increased uptake by constant numbers of M cells. Am J Pathol. 2004;164:65–72.
- Kim SH, Jang YS. The development of mucosal vaccines for both mucosal and systemic immune induction and the roles played by adjuvants. Clin Exp Vaccine Res. 2017;6:15–21.
- Lycke N. Recent progress in mucosal vaccine development: potential and limitations. Nat Rev Immunol. 2012;12:592–605.
- Jiang PL, Lin HJ, Wang HW, et al. Galactosylated liposome as a dendritic cell-targeted mucosal vaccine for inducing protective anti-tumor immunity. Acta Biomater. 2015;11:356–367.
- Asanuma H, Thompson AH, Iwasaki T, et al. Isolation and characterization of mouse nasal-associated lymphoid tissue. J Immunol Methods. 1997;202:123–131.
- Kuper CF, Koornstra PJ, Hameleers DMH, et al. The role of nasopharyngeal lymphoid tissue. Immunol Today. 1992;13:219–224.
- Zavari-Nematabad A, Alizadeh-Ghodsi M, Hamishehkar H, et al. Development of quantum-dot-encapsulated liposome-based optical nanobiosensor for detection of telomerase activity without target amplification. Anal Bioanal Chem. 2016;409:1301–1310.
- Qu W, Zuo W, Li N, et al. Design of multifunctional liposome-quantum dot hybrid nanocarriers and their biomedical application. J Drug Target. 2017;25:1–12.
- Bangham AD, Standish MM, Watkins JC. Diffusion of univalent ions across the lamellae of swollen phospholipids. J Mol Biol. 1965;13:238–252.
- Inaba K, Inaba M, Romani N, et al. Generation of large numbers of dendritic cells from mouse bone marrow cultures supplemented with granulocyte/macrophage colony-stimulating factor. J Exp Med. 1992;176:1693–1702.
- Wang H-W, Jiang P-L, Lin S-F, et al. Application of galactose-modified liposomes as a potent antigen presenting cell targeted carrier for intranasal immunization. Acta Biomater. 2013;9:5681–5688.
- Perrie Y, Kastner E, Kaur R, et al. A case-study investigating the physicochemical characteristics that dictate the function of a liposomal adjuvant. Hum Vaccin Immunother. 2013;9:1374–1381.
- Ingvarsson PT, Rasmussen IS, Viaene M, et al. The surface charge of liposomal adjuvants is decisive for their interactions with the Calu-3 and A549 airway epithelial cell culture models. Eur J Pharm Biopharm. 2014;87:480–488.
- Korsholm KS, Agger EM, Foged C, et al. The adjuvant mechanism of cationic dimethyldioctadecylammonium liposomes. Immunology. 2007;121:216–226.
- Ma Y, Poisson L, Sanchez-Schmitz G, et al. Assessing the immunopotency of Toll-like receptor agonists in an in vitro tissue-engineered immunological model. Immunology. 2010;130:374–387.
- Morel PA, Butterfiel LH. Dendritic cell control of immune responses. Front Immunol. 2015;6:1–2.
- Swartz MA, Hubbell JA, Reddy ST. Lymphatic drainage function and its immunological implications: from dendritic cell homing to vaccine design. Semin Immunol. 2008;20:147–156.
- Hinshaw VS, Webster RG, Easterday BC, et al. Replication of avian influenza a viruses in mammals. Infect Immun. 1981;34:354–361.
- Doherty PC, Turner SJ, Webby RG, et al. Influenza and the challenge for immunology. Nat Immunol. 2006;7:449–455.
- Tasaniyananda N, Chaisri U, Tungtrongchitr A, et al. Mouse model of cat allergic rhinitis and intranasal liposome-adjuvanted refined Fel d 1 vaccine. PLoS One. 2016;11:e0150463.
- Agger EM, Rosenkrand S, Hansen J, et al. Cationic liposomes formulated with synthetic MycobacterialCordfactor (CAF01) a versatile adjuvant for vaccines with different immunological requirements. PLoS One. 2008;3:e3116.
- Christensen D, Foged C, Rosenkrands I, et al. CAF01 liposomes as a mucosal vaccine adjuvant: in vitro and in vivo investigations. Int J Pharm. 2010;390:19–24.
- Werninghaus K, Babiak A, Gross O, et al. Adjuvanticity of a synthetic cord factor analogue for subunit Mycobacterium tuberculosis vaccination requires FcRgamma-Syk-Card9-dependent innate immune activation. J Exp Med. 2009;206:89–97.