Abstract
Dendrimers, commonly referred to as polymeric trees, offer endless opportunities for biotechnological and biomedical applications. By controlling the type, length, and molecular weight of the core, branches and end groups, respectively, the chemical functionality and topology of dendrimeric archetypes can be customized which further can be applied to achieve required solubility, biodegradability, diagnosis and other applications. Given the physicochemical variability of the dendrimers and their hybrids, this review attempts to discuss a full spectrum of recent advances and strides made by these “perfectly designed structures”. An extensive biotech/biomimicry application profiling of dendrimers is provided with focus on complex archetypical designs such as protein biomimicry (angiogenic inhibitors, regenerative hydroxyapatite and collagen) and biotechnology applications. In terms of biotechnological advances, dendrimers have provided distinctive advantages in the fields of biocatalysis, microbicides, artificial lights, mitochondrial function modulation, vaccines, tissue regeneration and repair, antigen carriers and even biosensors. In addition, this review provides overview of the extensive chemo-functionalization opportunities available with dendrimers which makes them a perfect candidate for forming drug conjugates, protein hybrids, bio mimics, lipidic derivatives, metal deposits and nanoconjugates thereby making them the most multifunctional platforms for diverse biotechnological applications.
Introduction
The term “dendrimer” was coined by the polymer chemist Donald Tomalia from the Greek “dendra” for tree and “meros” for part at Dow Chemical in Midland, Michigan [Citation1]. Afterwards in 1978, at the University of Bonn, Fritz Vögtle’s group synthesized dendrimers for the very first time when they tinkered with multi-functional branches [Citation2]. The dendrimer synthesis could be carried out through two established methods – divergent synthesis or convergent synthesis – and the choice of method is dependent on the end use application as explained in the following section (). Almost a decade ago, a Nature Biotechnology report by Vivian Marx remarking dendrimers as “yet to materialize into commercial success”, but still the research and interest in them is continuously growing [Citation3]. A decade later now, the dendrimers have not only “branched out” but also “rooted themselves deep into” the biomedical arena. Last few years have seen significant advancements in area related to polymeric carrier based drug delivery. Dendrimers are one of the key architectural class offering unique physicochemical and biological properties. The key structural features are their intriguing regions (the multifunctional surface, the tailored sanctuary within the branches, and the encapsulated core); the characteristic of property modification ability; and their potential applications in very diverse areas [Citation4]. These nanoscopic macromolecules offer ample opportunities owing to their low polydispersity index, characteristic intrinsic viscosity and glass transition temperature (both reaches a maxima and then declines owing to the absence of entanglement at higher molecular weight/generation) and uniform molecular weight (which increases with an increase in generation) [Citation5].
Figure 1. (a) Basis of two different approaches of synthesis of dendrimers. (b) Representation of amphiphilic dendrimer having liquid membrane at the interface between water and an immiscible organic solvent.
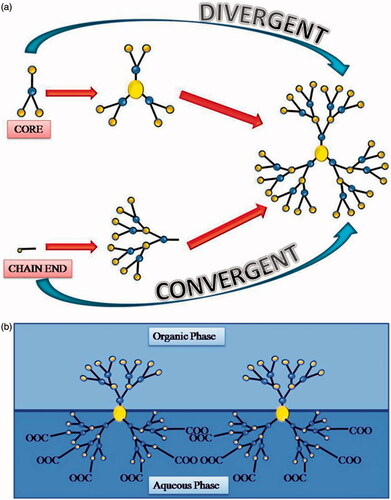
The major focus of the review is to summarize the possible strategies used to prepare dendrimers, their functionalization approach, post preparation characterization; and a detailed illustration of the various application arenas.
Approaches for synthesis of dendrimers
The synthesis of perfect dendrimers is imperative of the fact of difficulties in purification between perfect and imperfect dendrimers owing to their negligible size differences. Actually, dendrimers are assembled through Michael addition reactions which are outward extension reaction from a multifunctional core and further size and branches optimization. There are various index properties, such as solubility, thermal stability, which varies on functionality in each of the constituent parts of dendrimers along with addition of compounds for particular applications. Trailing generations is a phenomenon described for the condition of some branches remaining shorter than the others. Prevention of trailing generations at each step of the reaction is required so as to nullify their presence as impurities which could create negative impacts on the architecture and functionality [Citation6]. The two most commonly used approaches for dendrimer synthesis are explained in the subsections below.
Divergent dendrimer synthesis
In the divergent approach, the systematic addition of monomers starts from the central core, leads molecule formation till periphery by using two repetitive basic steps, i.e. coupling of individual monomers followed by monomers’ end-group transformation for creating new reactive surface for further coupling. The repetition of basic steps several times lead to the formation of varying generations of dendrimers with each new generation having started from earlier generations. During the start of developmental years of dendrimers, the synthetic production of PPI and PAMAM were based on divergent strategy. This approach was highly advantageous for commercial level production due to high yield linked with low purity. Divergent synthesis may produce impurities by missing the repeating unit of next side reactions with either the intramolecular/intermolecular cyclization or the retro-Michael addition [Citation7].
Convergent dendrimer synthesis
In convergent approach, the synthesis takes place from outer surface towards the inner core through one is to one joining of monomers. Such steps result in dendritic segments leading to increase in sizes with synthesis progression with the core introduced at the last level of synthesis. Generally, there are few reactive sites found during in the proliferation stage and these results to a faster reaction rate with enhanced yields. The separation of products from reactants during purification is facilitated by comparatively larger molecular difference between reactant molecules and the products. As a result of this approach, mixed structural features dendrimers are produced due to different segments coupling generating dendrimers with differential morphologies, better purification; better monodispersity and more symmetry. The dendrimers differential morphologies open up the interesting field on one side by combining numerous active sites in one dendrimer which creates a multifunctional macromolecule, but may offer structural hindrance in between dendron as they try to get attached to the core [Citation8].
Although the synthesis of dendrimers mostly involves two-step chemical reactions but if we talk about economy, different types of dendrimers show variable cost. The cationic poly(amidoamine) (PAMAM) dendrimers have been reported to have comparatively higher production as compared to disulphide cross-linked low generation PAMAM dendrimers [Citation9]. Overall, dendrimers have higher production cost [Citation10].
Functionalization of dendrimers
The creation of a macromolecule with multifunctional architecture by the process of incorporating multiple active sites (at either the core or periphery or even both) is known as functionalization of dendrimers or structurally controlled dendrimers. These dendrimers possess at least certain highly defined nanoscale features that are called as Critical Nanoscale Design Parameters (CNDPs). Out of these features, major of them are size, shape, rigidity, architecture, surface chemistry, flexibility and elemental composition. CNDP may control a wide range of new emerging properties which are critical for industrial and commercial applications [Citation11].
Xiangyang et al. have produced dendrimer coordinated nanoparticles where a metal ion complexation takes place with a dendrimer moiety, such as internal tertiary amines and surface-based functional groups with coordination bonding patterns or electrostatic strength-based interactions. This was carried out using poly(amidoamine) (PAMAM) dendrimers as templates or stabilizers. An effective loading of FeS nanoparticles (NPs) was observed using dendrimer-coated silica particles’ approach. Moreover, the production and manipulation of FeS NPs on medium porous silica micro-particles lead to their environmental remediation applications [Citation12]. In another study, Hamilton et al. utilized G5 hydroxyl-terminated PAMAM dendrimer for carrying out hydrophobic modification by conjugation reaction with dodecyl chloroformate and cholesteryl formate. It was observed that on increasing the amount of dodecyl modification, there was proportional increase in dendrimer’s toxicity. On the other hand, increasing cholesteryl modification did not have any toxicity changes [Citation13].
Characterization of dendrimers
Generally, dendrimers are characterized for using analytical techniques both from molecular chemistry (step by step synthesis approach) prospective as well as polymer chemistry (repetitive structures that are made up of monomers) prospective. Dendrimers are characterized for their chemical composition, morphology, shape, and homogeneity, synthesis, conjugation, reaction rates, molecular weight, structural defects and purity of dendrimers. The detailed information can be obtained from the review, such as Augustus et al. [Citation14]. The brief description of the methods used for characterization has been provided hereunder.
a) Spectroscopic techniques
Spectroscopic analytical methods measure the radiation produced and absorbed by atomic or molecular species.
Ultraviolet–visible spectroscopy: The technique validates surface modification and synthesis of dendrimers by measuring the characteristic absorption maxima or a shift in its value.
Infra-red spectroscopy: It provides information regarding chemical transformation at dendrimer surface.
Nuclear magnetic spectroscopy: It helps in molecular structure and dynamics determination.
Fluorescence spectroscopy: It provides information about interactions between dendrimers and chemical additives by analysing sample fluorescence.
X-ray diffraction: It provides precise chemical composition, shape and size of dendrimers.
Mass spectrometry: It is used to determine the purity of different types of dendrimers including PAMAM and phosphorus dendrimers.
b) Microscopic techniques
Scanning electron microscopy: It is used to study dendrimeric surface topography.
Transmission electron microscopy: It is used to determine size, size distribution and shapes.
c) Size exclusion chromatography: It is size based separation technique and can be utilized for the categorization of dendrimers based on their size.
d) Electroanalytical techniques
Electron paramagnetic resonance: It is used to study compounds having one or more unpaired electrons.
Electrophoresis: It helps in determining purity and homogeneity of different types of dendrimers having good aqueous solubility.
Electrochemistry: It is employed to identify oxidation–reduction process.
e) Scattering techniques:
Small angle X-ray scattering: It helps in determining the radius of gyration (Rg).
Small angle neutron scattering: It gives accurate information about the internal structure of dendrimers.
f) Rheological and physical properties:
Intrinsic viscosity: It determines dendrimer’s morphology.
Differential scanning calorimetry: It is used for detecting glass transition temperature and physical ageing.
Dielectric spectroscopy: It provides insight into the processes involved in molecular dynamics.
Applications of dendrimers
The areas where dendrimers find their applicability is very wide. As far as their biomimicry and biotechnological applications are concerned, the relevant reports published have been arranged in chronological order in . All these applications are summarized in the form of a schematic digital image in .
Table 1. Applications of dendrimers in various fields (chronological order) with special reference to biotechnology and biomimicry.
Dendrimers in biomimicry
Dendrimers as proteo-mimicking platforms
It is crucial to note that because of the high molecular weight of this macromolecule and branched artificial architecture, dendrimers serve as an excellent choice for biomimicry of proteins. Dendrimers like PAMAM dendrimer G2 matches the width (2.4 nm) of DNA duplexes, ammonia core PAMAM dendrimer G3 matches with insulin (3 nm), PAMAM dendrimer (with ammonia core) G4 matches with Cytochrome C (4 nm), PAMAM dendrimer (with ammonia core) G5 with haemoglobin (5.5 nm) in size and shape.
Shcharbin et al.’ study revealed five binding sites to cationic dendrimers [Citation15]. Also, 4 G PAMAM dendrimer interaction with human and bovine serum albumin (HAS and BSA) showed that serum albumins and PAMAM G4 dendrimer forms the complex with stoichiometry of 4–6:1 for G4: HSA and 4–5:1 for G4: BSA molar ratio. They even proposed that dendrimers do not significantly affect the protein secondary structure [Citation15].
Kojima and Fukushima prepared thermosensitive elastin-mimetic dendrimers (ELP-den) by conjugating elastin-like peptides having repeats of valine-proline-glycine-valine-glycine to dendrimers. This preparation was used as vehicle for gold nanoparticles (AuNPs) to be used in photo-thermal therapy. It was observed that the complex exhibited both thermosensitive and photo-thermal properties with efficient photocytotoxicity. This led to the conclusion that ELP-den could serve as an efficient carrier for AuNPs in photothermal therapy [Citation16].
Mimicking dendrimers in anti-angiogenic/angiogenic effect
Angiogenesis (growth of new vessel from already existing vessels) is the key process in tumour growth which is governed by vascular endothelial growth factor (VEGF) and basic fibroblast growth factor (b-FGF) [Citation17]. Angiostatin and endostatin have anti-angiogenesis property. Endostatin is an endogenous angiogenic inhibitor is made up of compact globular protein. It has a positive-charge and 15 arginine residues at the surface. In the extracellular matrix, heparin and heparan sulphate proteoglycan (HSPG) are present. Endostatin binds with them to inhibit angiogenesis [Citation17]. To mimic this mechanism, Kasai et al. designed novel dendrimers, TX-1943 (8 arginine residues) and TX-1944 (16 arginine residues), with heparin binding properties. Due to this, these novel dendrimers were capable to show potent anti-angiogenic activity both in vitro (in chorioallantoic membrane assay) and in vivo [Citation17]. Although polycationic dendrimers are known to possess anti-angiogenic effects, Abdel-Sayed et al. observed two dendrimers (G3KL and G3RL) to have angiogenic effects which in turn were beneficial for wound dressing applications. These dendrimers were found to destroy multi-drug resistant Pseudomonas aeruginosa (referred to as antimicrobial peptide dendrimers) and showed angiogenic effect by initiating neovascularization in deep burn-wounds healing process. Furthermore, when combined with biological bandages prepared from progenitor skin cells, these dendrimers were found to significantly improve wound healing in deep burns. This interesting study concluded that by controlling the topology and sequencing in dendrimers, their interference with the “electrostatic complexation of heparin with partner proteins” can be modulated and hence the angiogenic properties [Citation18]. Further research is warranted in this area to better understand the mechanism and modulation of anti-angiogenic/angiogenic effect of dendrimers.
Biomimetic regeneration of hydroxyapatite
The biomimicry concept has also been applied to the hardest mineralized tissue in the human body, dental enamel (96% inorganic materials; 4% organic materials; and water by weight). To mimic the biomineralization process of dental enamel, amelogenin (an extracellular matrix protein) has been utilized. During the bio-mineralization process of enamel, amelogenin is essential for the managing the nanorod-like hierarchical structure and hydroxyapatite [Citation19,Citation20].
Chen et al. researched on biomimetic regeneration of hydroxyapatite. Their investigation used artificial proteins (dendrimers) to mimic protein-crystal interaction during bio-mineralization. They reported that PAMAM dendrimers when attached with carboxylic acid at the end can be absorbed to enamel crystals [Citation19]. Wu et al. synthesized carboxyl-terminated poly(amidoamine) (PAMAM-COOH), alendronate (ALN) conjugate (ALN-PAMAM-COOH). By utilizing the organic matrices, biomineralization process was mimicked for developing enamel and to boost the binding strength at the remineralization interface (). Overall, promising results for this biomaterial (ALN-PAMAM-COOH) was reported to be used as an in-situ enamel remineralization [Citation21]. Huysal and Senel synthesized Hydroxyapatite nanoparticles modified PAMAM dendrons with siloxane core. This was explored for both bio-composite material and bone tissue engineering. Initially, five different generations of the siloxane-cored PAMAM dendrons were synthesized followed by the synthesis of hydroxyapatite nanoparticles by hydrothermal crystallization method [Citation22].
Figure 3. Schematic demonstration of the specific adsorption of ALNePAMAMeCOOH on the surface of tooth enamel and the subsequent in situ remineralization of HA reproduced with permission from Elsevier B.V. Ltd.© 2013 [Citation21].
![Figure 3. Schematic demonstration of the specific adsorption of ALNePAMAMeCOOH on the surface of tooth enamel and the subsequent in situ remineralization of HA reproduced with permission from Elsevier B.V. Ltd.© 2013 [Citation21].](/cms/asset/7a297dea-b63e-446d-b227-619fd911b73a/ianb_a_1438451_f0003_c.jpg)
Collagen-mimetic dendrimers
Collagen is the most abundant, essential and fundamental component of extracellular matrixes of mammals’ connective tissue (comprised of one-third fraction of the total body proteins). In other words, the major component of connective tissues like skin, hair, cartilage, bone and tendonitis collagen. To supplement collagen in human body, human dead bodies and bovine sources are utilized which may induce allergic reactions. There is a need for crosslinking as collagen has weak mechanical properties and is rapidly biodegraded. Structurally, collagen consist of triple helical peptides compose of Gly-Xaa-Yaa trimer repeats. Many synthetic collagen mimetic dendrimers have been developed as synthetic and artificial collagen analogous [Citation23]. To mimic collagen, Kinberger et al. synthesized dendrimers with trimesic acid (TMA) core. The sequence for this collagen-mimetic dendrimer was Gly-Pro-Nleu and Gly-Nleu-Pro. The triple-helical structure of the synthesized dendrimer was confirmed using optical rotation and circular dichroism techniques. Further, report revealed that Gly-Nleu-Pro sequence was having comparatively more thermal stability than the equivalent structures formed from the Gly-Pro-Nleu sequence [Citation23].
In the same direction, to mimic collagen, Gly-Pro-Nleu sequence was utilized on a G1 PAMAM core. The collagen-PAMAM conjugates were synthesized to prepare collagen mimetic dendrimers with improved functionality as compared to the TMA-based structures discussed above. Further, the consequences of different dendritic structural design effect were studied for collagen mimetic melting transitions by synthesizing them. Reports revealed that complexion of the synthesized PAMAM collagen-mimetic conjugates with metal cofactors, such as Cu2+ and Ni2+ resulted in extraordinary biological properties [Citation24]. Zhao et al. investigated the effects of molar masses, substrates, polypeptide structures, and solvent polarity on the supramolecular assembly of oligoethyleneglycolated (OEGylated) collagen mimetic polypeptides (CMPs). They synthesized CMPs pendanted with dendritic or linear OEG using ether linkage and observed that CMPs with dendritic OEGs showed characteristic thermos-responsive properties. It was proposed that a periphery having solvent-favourable dendrons and a backbone having solvent-unfavourable polypeptide may enhance the supramolecular assembly of the prepared OEGylated CMPs that aid in formation of long fibres [Citation25].
Biotechnological applications of dendritic platforms
Major application of dendrimers has been found in the field of biotechnology and modern medicine systems. Their multiple tunable characteristics on the basis of multiple replaceable groups and their alterable carrier capacity make them suitable for profound application in these fields. Among many possibilities this molecule finds application in catalysing a reaction, as microbicide, light harvesting hub, MRI contrast agent, mitochondrial protectant, vaccine, tissue generator and cell repair. These aspects are further discussed in detail hereunder [Citation26].
Catalytic reactors
The nanoscopic cavities/catalytic pockets formed of core amino acids sequences armed with either focal core or specific functional groups for specialized transformation may serve as an excellent reactor site for reaction at molecular level. With the objective to mimic pyruvate oxidase using dendrimers, Habicher et al. synthesized catalytic dendrophanes consisting of thiazolio-cyclophane attached with four G2 poly(ether amide) dendrons. The construct gave rise to ester-oxidation from aldehyde by flavin as anchor cum cofactor. However, the reaction kinetics was hundred times slower in comparison with the thiazolio-cyclophane system without dendritic branches [Citation27].
In order to study dendrimeric core for catalytic activity modification Liu and Breslow covalently bonded pyridoxamine reagent at the PAMAM core with increasing generation stages which resulted in better reactivity (three orders of magnitude) for transamination with pyruvate and phenylpyruvate in aqueous media. The dendrimer amine backbone and hydrophobic substrate binding are the major factors in acid–base catalysis which may be responsible for this improvement [Citation28]. Albiter et al. encapsulated the dendrimers in platinum nanoparticles and dispersed them on homemade sol–gel silica support having large surface area. The catalyst was activated using hydrogen treatment [Citation29]. With an aim to have catalytic properties; convergent approach was utilized to form single-walled carbon nanotube-polyamidoamine dendrimers hybrids (SWCNT-PAMAM) [Citation30]. In this study, a direct reaction of PAMAM dendrimers (Cystamine-based 2.5 and 3.0 G) with pristine SWCNTs was performed in toluene under reflux. Small palladium nanoparticles were formed by the immobilization and reduction of [PdCl4]2−. It was followed by the formation of nanoparticles which were homogenously dispersed along the length of the nanotube. In Suzuki and Heck reactions, the formulation was found to function as a proficient catalyst. The important quality is that this hybrid has the ability to recycle up to 6 times after recycling [Citation30].
Antiseptic and microbicidal activity
The outer membrane of gram-negative bacteria consists of lipopolysaccharides (LPS) which are endotoxins and play a vital role in septic shock, a major cause of mortality in severely ill patient and to combat sexually transmitted diseases and HIV. Cromer et al. demonstrated that in vitro binding of PAMAM dendrimer with surface amines sub-stoichiometrically derivatized with alkyl groups binds to LPS with high affinity which ultimately results into neutralization of LPS based inflammatory response and provides protection from endotoxic shock in murine model [Citation31].
In order to make carbon nanotubes (CNTs) water soluble and biocompatible, they first have to be functionalized. Neelgund et al. deposited in situ CdS and Ag2S quantum dots on PAMAM dendrimers and converted it into an anti-bacterial complex by in situ deposition of CdS and Ag2S quantum dots. The complex was further grafted onto MWCNTs (multiwalled carbon nanotubes). The f-MWCNTs-CdS and f-MWCNTs-Ag2S were checked for antibacterial activity against E. coli, P. aeruginosa and S. aureus and it was concluded that germicidal activity of MWCNTs was increased via PAMAM grafting [Citation32].
For controlling the sexual life of women and as a safeguard against sexually transmitted infections and HIV, numerous researchers have been carried out over the past two decades for the development of safe and effective microbicides. In this direction, Chonco et al. synthesized Second generation water-soluble anionic Carbosilane dendrimer S16 for the treatment of HIV infection. For cytotoxicity evaluation, study was carried out on the genital tract of female rabbit. It was observed that the synthesized dendrimer quickly blocked activated peripheral blood mononuclear cells infection. On carrying out toxicity analysis 2G-S16 was found to be highly biocompatible and no cellular and local inflammation were observed. The blocking effect of 2G-S16 for HIV transmission in epithelial monolayer suggests that dendrimer can be explored at high level as anti-HIV or anti-viral agent [Citation26].
Carbosilane dendrimers have shown promising results as anti-viral agents against HIV infection. Vacas-Cordoba et al. developed two sulphated (G3-S16) and naphthyl sulphonated (G2-NF16) carbosilane dendrimers. Both were found to inhibit HIV-1 infection at fusion stage. They impeded viral binding at the cell surface and hindered membrane fusion by blocking gp120-CD4 interaction. The study demonstrated for the first time that dendrimers could successfully inhibit cell-to-cell transmission of HIV and formation of infectious synapse. Thus, it was concluded that carbosilane dendrimers could have antiviral effect by blocking HIV infection in the initial steps [Citation33].
Artificial light harvesting and gleaning
Light gleaning or photosynthesis is an everyday process ultimately providing us with energy. In the process antennas (chromophores) absorb photons and transfer them to reaction centre where light is converted to useful chemical energy by supramolecular biosystem. Dendrimer is a molecule of multiple decorations. On replacing end groups with chromophores, it can prove as a mimic artist for light harvesting hub. The role of dendrimer in light gleaning was for the first time studied by Devadoss et al. where they showed that phenylene ethylene dendrimer showed strong UV absorption between 250 and 350 nm and could further emit at range of 350–450 nm by core chromophores thus acting as light harvesting unit [Citation34]. Adding more detail to this concept Paulo and Costa reported electron-transfer by dendrimer. In their study, zinc meso-tetrakis (Nmethylpyridinium-4-yl) porphyrin (ZnTMPyP4+) was complexed with poly (amidoamine) dendrimers (PAMAMs) and electron transfer was triggered via photoexcitation of complex. They also showed the effect of air in the process, which could be concluded from the following result. Photo-induced electron-transfer between the 1:1 associated complex of ZnTMPyP4 + porphyrin and PAMAM dendrimers could only induce a weak fluorescence quenching effect in air-equilibrated aqueous solutions. The process triggered a sequence of photoreduction reactions under deaerated conditions that degraded ZnTMPyP4 + successively first to chlorine and further to tetrahydroporphyrin forms. Methyl viologen (Mv2+) was the inhibitor of this process as it acted as an electron acceptor for the radical ion ZnTMPyP3 + formed during primary electron-transfer step. Laser flash photolysis is the technique of choice providing insight on electron-transfer reactions between the triplet state of ZnTMPyP4+ and methyl viologen [Citation35]. Recently, Yang et al. prepared light-harvesting dendrimer zinc-phthalocyanines chromophores labelled-single-wall carbon nanotubes (SWNTs) nanoparticles. Photo-induced intramolecular electron transfer was reported from phthalocyanines (donors) to SWNTs (acceptors) with an increase in the exchange rates and efficacies of electron transfer with decrease in length of spacer linker (either ethylenediamine or hexamethylenediamine). It was suggested that these nanoconjugates may be applied further for biological labelling and drug delivery applications [Citation36].
Contrast agent for magnetic resonance imaging
Paramagnetic metal ions have been utilized before magnetic resonance imaging (MRI) to lower the relaxation time of nearby water protons and enhance the intensity of the signal without using the harmful ionizing radiations. Such metal ions are actually known as contrast agent for MRI. The low molecular weight contrast agents have short circulation time and they cannot distinguish normal and diseased tissues very efficiently. To overcome such limitations, Kobayashi et al. formulated six small dendrimer-based MRI contrast agents and investigated their whole-body retention, pharmacokinetics and dynamic MRI. It was observed that diaminobutane (DAB) dendrimer-based agents showed better clearance than PAMAM dendrimer-based agents and smaller dendrimer conjugates excreted more rapidly as compared to larger ones. With DAB-G2 & -G3 and PAMAM-G2 showing rapid excretion, it was suggested that these conjugates could be used for future clinical applications [Citation37].
Before treatment of metastatic tumours, the diagnosis of metastasis is crucial. Instead of accumulating in cancer cells, MRI contrast agents may accumulate in Kupffer cells and hepatocytes of liver. This impedes MRI of liver metastases. In an attempt to avoid accumulation of contrast agent in Kupffer cells and hepatocytes, fourth generation biodegradable dendritic contrast agents were synthesized by Zhou et al. The dendritic contrast agent avoided its uptake by Kupffer cells and hepatocytes and showed enhanced permeability and retention effect. It also showed minimal long-term retention of gadolinium (III) in tissues and organs. Thus, it was suggested that DCA could serve as a potential candidate as contrast agent for metastatic tumour imaging [Citation38].
Dendrimers in apoptosis
One of the greatest concerns associated with nano-materials is their potential to adversely affect human health. Lee et al. studied genes expression in human lung cells when exposed to PAMAM and found out that there is downregulation of gene expression in mitochondria. They further showed that PAMAM downregulated mitochondrial DNA encoded genes involved in maintenance of mitochondrial membrane potential in human lung cells (WI-26 VA4). They explained that dendrimer co-localized with mitochondria caused the release of cytochrome C. This resulted into disruption of membrane potential and occurrence of cell death in presence of dendrimer. This property of dendrimer establishes them as cytotoxicity inducer but still other endothelial cells need to be tested before arriving at a final conclusion. It is further commented that this property of dendrimer can be utilized for rational killing of specific cancer cells in vivo through specific receptors or any other specific targeting mechanism [Citation39].
With the objective to study the toxic impact of dendrimers on human health and the environment, Bodewein et al. tested G3.0, 3.5, 4.0, 4.5 and 5.0 PAMAM dendrimers and G3.0, 4.0 and 5.0 PPI dendrimers on zebrafish embryos and human cancer cell lines. It was observed that the toxicity of cationic PAMAM and PPI dendrimers in zebrafish embryo increased over time; however, there was no increase in toxicity with increase in generations. Apoptosis was induced in zebrafish embryos with cationic dendrimers, while little or no toxicity was observed in cells and fish embryos with anionic PAMAM dendrimers. HepG2 and DU145 cells showed lower toxicity with cationic dendrimers than zebrafish embryos [Citation40].
Chronic lymphocytic leukaemia (CLL) is associated with a failure in apoptosis pathway and increased proliferation. Although, a large number of anti-leukemic drugs are available, CLL is still incurable. With the objective to study the effect of PPI dendrimers in CLL, Franiak-Pietryga et al. treated normal and CLL cells with PPI dendrimers having peripheral amino groups and coated up to 30% with maltotriose (PPI-G4-OS-Mal-III). It was observed that the dendrimer complex, which acts as a drug itself, acts by inducting apoptotic mechanism. The results showed that PPI-G4-OS-Mal-III significantly influenced CLL cells viability without significantly harming normal lymphocytes. It also had a significant effect on protein and gene expression in CLL cells. It was thus suggested that dendrimers may be an effective option for CLL therapy as the effect of PPI-G4-OS-Mal-III was found to be stronger than anti-leucocyte drug fludarabine, in vitro [Citation41].
Modulating mitochondrial function and protection
Mitochondria are known to play a vital role in insulin resistance and diabetes pathogenesis [Citation42]. In diabetes, there is a decrease in efficiency of mitochondrial respiration especially in tissues like brain and heart cells that are highly dependent on aerobic metabolism. This decreased efficiency is due to disturbance in mitochondrial functioning, reduction in electron transport chain, which in turn lowers the ATP output. Reduced ATP formation decreased oxidative capacity and increased reactive oxygen species (ROS) production are common characteristics of diabetic heart [Citation42].
Labieniec and Gabryelak reported the role of dendrimer in modulating mitochondrial functions. The study demonstrated that tested dendrimer of G3.5 protected mitochondria against depolarization induced by Ca2 + ions overloading. They investigated membrane potential and Ca2 + ions uptake of mitochondria exposing to G3.5 at concentration of 10, 30 and 50 μm. Results revealed that reduction in transmembrane potential occurred above concentration of 10 μm the mechanism of which could be either hindrance of externally added Ca2 + ions influx to mitochondria, or by acceleration of their efflux from mitochondria [Citation43].
In another study, Siewiera and Labieniec-Watala came with puzzling results when in vitro data suggested that PAMAM G3 can be employed in mitochondrial protection against MG (Methyl glyoxal)-induced impaired respiratory activity. However, in vivo experimentation did not support the in vitro results. Further study revealed that G3 dendrimer activity could be toxic with higher mortality ranges. The author’s view supports experimentation with animal models as in vitro studies does not completely relate with live animal models, and the outcome of the two could be anomalous [Citation42].
With the objective of developing mitochondrial targeted second-generation drug delivery systems, Paleos et al. decorated the external surface of dendritic polymer with triphenylphosphonium cation. These functionalized nanoparticles were able to successfully accumulate within the mitochondria in vitro. It was thus suggested that DDSs can be used for effective drug delivery for mitochondrial diseases and predicted the emergence of a new medicine called mitochondrial medicine [Citation4].
Dendritic vaccines
For vaccination, genetic immunization has been reported as quite promising strategy owing to its potent cell-mediated immune response and the antibody response against the corresponding antigen. Various delivery systems like plasmids, cationic liposomes, nanoparticles and dendrimers have been tested for gene delivery and genetic immunization methods. Dendrimers offer certain characteristic properties such as spherical shape, cationic charge, reactive 3D structure, high degree of readily accessible terminal functional groups, nano size, customized molecular weight, possibility to carry a guest molecule; ability to evade reactive endothelial cells (RES) of the body [Citation1].
Dendrosomes, in addition to be novel, spherical and vesicular may overcome the haemolytic toxicity and offer higher transfection efficacy and in vivo acceptability [Citation1]. Dutta et al. used reverse phase evaporation for preparing dendrosome formulation (DF3: possessing optimum vehicle size, entrapment efficiency and zeta potential) from poly(propyleneimine) dendrimers (PPI 5.0). In vivo immunization with 10 μg of plasmid DNA (pDNA) administered intramuscularly or its dendrimeric or dendrosomal formulation showed that DF3 evoked high-immune response in terms of total IgG and its subclasses. The above work showed superiority of dendrimeric complexes over PPI–DNA complex and pDNA for genetic immunization against hepatitis B [Citation1].
Stability of peptides in vivo and enhanced peptide immunogenicity is major issues in peptide-based vaccines. Ganda et al. synthesized G4 hydroxyl-terminated PAMAM dendrimers conjugated with peptide mimic of chlamydial glycolipid antigen – Peptide 4 to study its anti-microbial feasibility against Chlamydia trachomatis. The conjugate was found to enhance Chlamydia-specific antibodies in serum, provided significant protection against C. trachomatis, and reduced tissue damage in the genital tract (). The results suggested that hydroxyl-terminated PAMAM dendrimers are promising platform for peptide vaccines delivery [Citation44].
Figure 4. Immunostaining analysis of cells infected in vitro by different Chlamydia species. When Chlamydia-specific antibodies bind to chlamydial organisms and matrix material inside inclusions (I) within infected cells, the secondary anti-IgG antibody FITC conjugate has shown green colour. Uninfected cells do not contain inclusions. Sera from mice immunized with: PBS, Pep4 + Al(OH)3; G4OH-Pep4, or G4OH + Pep4 were evaluated for the presence of chlamydia-specific IgG Ab. Pictures represent HEp-2 cells infected with C. trachomatis and incubated with immune mouse sera at the same dilutions. Cell nuclei (N) and Chlamydia inclusions (I) are indicated. Animals evaluated per group: C. trachomatis (n = 7–8) and C. muridarum, C. abortus and C. pecorum (n = 2). 6–9 inclusions were analysed per slide. Images were photographed under identical conditions reproduced with permission from Elsevier B.V. Ltd.© 2017 [Citation44].
![Figure 4. Immunostaining analysis of cells infected in vitro by different Chlamydia species. When Chlamydia-specific antibodies bind to chlamydial organisms and matrix material inside inclusions (I) within infected cells, the secondary anti-IgG antibody FITC conjugate has shown green colour. Uninfected cells do not contain inclusions. Sera from mice immunized with: PBS, Pep4 + Al(OH)3; G4OH-Pep4, or G4OH + Pep4 were evaluated for the presence of chlamydia-specific IgG Ab. Pictures represent HEp-2 cells infected with C. trachomatis and incubated with immune mouse sera at the same dilutions. Cell nuclei (N) and Chlamydia inclusions (I) are indicated. Animals evaluated per group: C. trachomatis (n = 7–8) and C. muridarum, C. abortus and C. pecorum (n = 2). 6–9 inclusions were analysed per slide. Images were photographed under identical conditions reproduced with permission from Elsevier B.V. Ltd.© 2017 [Citation44].](/cms/asset/0c78da9a-5282-405b-95a7-1c0ffeecbb6a/ianb_a_1438451_f0004_c.jpg)
Tissue regeneration and stem cell therapy
There has been a great progress in the field of tissue engineering over the past few decades. This field aims at regenerating native tissue by supplementing the body’s natural healing process or creating entire organs for transplantation. The first step in the tissue construction is the selecting an appropriate scaffold material. The goal of tissue engineering applications is to encapsulate cells that would regenerate native extracellular matrix and finally replace the scaffold altogether. Therefore, the rate of biodegradation of the scaffold must complement the biosynthesis of new extracellular matrix.
Reports reveal that corneal tissue can be regenerated using poly(propylene)imine octaamine dendrimer. The in vitro studies revealed the incorporated peptide enhanced adhesion and proliferation of corneal epithelial cell culture. There was also an enhancement of nerve cell density and neurite extension. The study demonstrated a novel strategy to obtain polymer-based tissue engineering scaffolds [Citation45]. For bone tissue engineering, the researchers used dexamethasone carboxymethyl chitosan/PAMAM dendrimer and were able to modify the cellular microenvironment which resulted in increased alkaline phosphatase level and modified mineralization characteristics. In the scaffold, the increased ectopic early osteogenic differentiation of rat bone marrow stromal cells; and calcium deposition resulted in higher mineralization and better scaffold constructs exposure to the dexamethasone carboxymethyl chitosan/PAMAM dendrimer environment [Citation45].
In stem cell therapy, targeting therapeutic cells to damaged tissues remains a great challenge. Liu et al. coated cell surfaces with dendrimer nanocarriers modified with adhesion molecules. These coated bone marrow cells efficiently reached grafted corneas and cutaneous wound tissues and were found to be advantageous over conventional BMC transplantation for neovascularization and wound healing. It was suggested that this platform could be used for targeted systemic delivery of almost all therapeutic cell types [Citation46]. Abdel-Sayed et al. reported the effectiveness of dendrimers in treating burn wound exudates. An in vitro scratch assay with keratinocytes revealed the cell migration owing to the presence of antimicrobial dendrimers. Actually, the “biological bandage” invigorated keratinocyte relocation. This resulted in comparatively quicker (within 72 h) in vitro healing of wound [Citation18].
Dendrimers in cell repair
The nanotechnology has emerged as a vital tool that can re-order matter at subatomic level. The dendrimers molecules offer a promising approach for cellular level repairing of cell/tissue/organs. Repairing is actually working structure by structure viz., starting from cell to cell repair; progressing to tissue to tissue; followed by organ to organ; and finally, a healthy body. Avenues for new medical approach are “dendrimers” which offer their customized terminal groups’ chemistry; control on cell proliferation rates and biodegradation profiles owing to systematic variation of generation, size, concentration and molecular weight. The prime focus of tissue engineering is the synthesis of scaffolds which are nothing but biocompatible porous materials which can support the growth; direct the differentiation of cells; diffuse nutrients to and waste product from the cells; and monitor the material-biological interactions. The studies on most of the tissue engineering applications are based on the regeneration of the extracellular matrix of encapsulated cells and ultimately replace the scaffold on the whole.
Star polycaprolactone-hydroxyapatite films were fabricated and synthesized by several researchers. These films were used as a biodegradable matrix for bone tissue engineering. They fabricated an HA-composite for in vitro bone regeneration using a hybrid scaffold by conjugating poly(caprolactone) (PCL) chains to poly(L-lysine) dendritic core. The outcome of these scaffolds was evaluated in the cell line MG-63. They found the performance of dendrimer-PCL HA hybrid to be much better than linear PCL, thereby suggesting dendritic architecture to be advantageous over linear polymers for in vitro bone cell growth and adhesion over 24 h [Citation47].
It has been reported that using G1 PAMAM dendrimer as a model dendrimer and incorporating it into laboratory developed cholecyst-derived extracellular matrix may provide with a novel intact extracellular matrix derived from the perimuscular subseosal connective tissue of porcine cholecysts. Using the EDC/NHS cross-linking system, incorporation of varied feed concentrations of PAMAM dendrimer in CEM was observed resulting in covalent binding of PAMAM on CEM. Diverse degrees of cross-linking increased amine functional groups useful in tethering bioactive agents, improved stability of CEM to enzymatic degradation and maintained tensile strength; however, there was an increase in flexibility of scaffold. In vitro preservation property of DENCEM to support cells was observed [Citation47,Citation48].
If we consider the disease osteoarthritis, there is need for efficient medical treatment. Actually, the major limitation in this direction is the inefficient delivery of pharmacologic agents in the joints. To solve this, Hu et al. developed PEG-PAMAM nanocarrier for the delivery of kartogenin (a small hydrophobic molecule which promotes the selective chondrogenesis) to look up its effectiveness in the cytoplasm for induction of chondrogenic differentiation of mesenchymal cells. Chondrogenesis process is the formation of cartilage from condensed mesenchyme tissue. This synthesized conjugate improved chondrogenesis efficacy. The conjugate stayed in the joint cavity of osteoarthritis and healthy rates for longer time. The study suggested PEG-PAMAM dendrimer to be a potential carrier for intra-articular (IA) drug delivery for treating osteoarthritis [Citation49].
Dendrimers as antigen carriers
Continuous research has been going on to invent a vaccine for human immunodeficiency syndrome (AIDS), since any combination of drugs has not been able to cause a complete cure of AIDS yet [Citation50]. Dendrimers may enhance the efficacy of vaccines by carrying antigens and immune-stimulatory additives. By generating such “multimeric antigen conjugates” by saving the host from immunogenic and inflammatory adverse reactions [Citation51].
Acute and post-infection complications, such as rheumatic heart disease and rheumatic fever have been associated with Streptococcus pyogenes infection. It is commonly known as group A Streptococcus. Rheumatic heart disease is a global health burden with Australia’s indigenous population reported having one of the highest incidences of rheumatic heart disease globally. Previously, from the C-terminal region of M protein, peptide (J14) vaccine has been recognized. However, lack of adjuvants and carriers suitable for human use hamper the development of such peptide-based vaccines. Polyacrylate dendritic polymer-based synthetic peptide subunit vaccine candidate was developed by Zaman et al. Induction of J14-specific IgG and in vitro opsonization of GAS was observed upon administering the nanoparticulate system intranasally without any added adjuvant, thereby suggesting the self-adjuvanting potential of polyacrylate nanoparticle-based concept for peptide vaccine delivery system. It was concluded that the platform may provide promising opportunities for the treatment of systemic GAS infection [Citation52]. Mesa-Antunez et al. synthesized a novel fluorescent dendrimeric antigen (DeAn) based on a dendron with amoxicilloyl terminal groups using all-aliphatic polyamide dendrimer (Bis- aminoalkyl-polyamide dendrimers [BAPAD]) were used as a synthetic antigen. Results revealed these dendrons to be non-toxic and they could be easily internalized by dendritic cells. It was concluded that BAPAD dendrons could serve as efficient candidates for synthetic scaffolds for such applications [Citation53]. Thus, dendrimers have emerged as one of the promising technique for specific delivery of antigens. The dendrimers will release the antigens as controlled drug delivery vehicle thus making them more specific and potent in their activity [Citation54].
Dendrimers as modified electrodes/biosensors
Suitable biorecognition receptors (like antibodies, enzymes, DNA or ligand proteins) makes transducers to sense analyte molecule in given sample. It is made possible owing to the formation of detectable signals by the interaction between the analyte with the biorecognition receptor. To monitor this signal, various techniques have been utilized. These techniques include electrochemical detection, optical detection, piezoelectric detection or electrochemiluminescence resonance energy transfer. The interesting approaches are affinity-based biosensor and those based on some biochemical reactions [Citation55,Citation56]. Zhu et al. introduced new impedimetric DNA biosensor using PAMAM dendrimer. Its preparation included PAMAM G2 covalently bonded to multi-walled carbon nanotube (MWNT) electronic transducers as the tether for probe DNA’s surface confinement. By acting as tether for probe DNA, G2-PAMAM dendrimer provided a large number of amino groups that increased the surface binding of probe DNA. This increased the sensitivity of the impedimetric biosensor for the target DNA. This impedimetric DNA biosensor provided excellent analytical properties and is potentially useful for practical applications [Citation57]. Cytochrome P450 3A4 (CYP3A4) is an enzyme responsible for the inactivation of a large number of drugs. Müller et al. constructed a CYP3A4-containing biosensor in which sub-nanometre gold particle-modified G4 PAMAM dendrimers were adsorbed in alternate layers with the enzyme. This biosensor was then used for the detection of caffeine [Citation58].
For biochemical reaction based biosensors, PAMAM dendrimer layer have been utilized to increase the electrode surface area and increase the sensitivity. Uygun et al. developed polyhydroxylated-fullerene-PAMAM composite which reduced the overall analysis time, with a better sensitivity and wide range of deflections as compared to already existing ELISA method [Citation55]. Similarly, for sensing hydrogen peroxide, the co-polymers of pyrrole PAMAM dendrimer have been utilized. Using divergent technique, different generations of pyrrole-PAMAM dendrimers were synthesized with branched amine periphery and focal pyrrole group. Further, the conjugate is covalently attached to the electrode surface and followed by the horseradish peroxidase immobilization so as to form hydrogen peroxide sensing conducting films [Citation56]. Additionally, amperometric method based glucose sensor was synthesized using pyrrole-PAMAM dendrimer and ferrocenyl dendrimer to immobilize glucose oxidase. Actually, pyrrole-containing PAMAM encapsulated Pt nanoparticles (Pt-PAMAM) were electropolymerized with immobilized glucose oxidase in pyrrole-PAMAM dendrimer and covalent modification of gold electrode with 3-mercaptopropionic acid, ferrocenyl PAMAM dendrimers and immobilizes glucose oxidase [Citation56].
Zhu et al. introduced new impedimetric DNA biosensor using PAMAM dendrimer. Its preparation included PAMAM G2 covalently bonded to multi-walled carbon nanotube (MWNT) electronic transducers as the tether for probe DNA’s surface confinement. By acting as tether for probe DNA, G2-PAMAM dendrimer provided a large number of amino groups that increased the surface binding of probe DNA. This increased the sensitivity of the impedimetric biosensor for the target DNA. This impedimetric DNA biosensor provided excellent analytical properties and is potentially useful for practical applications [Citation57]. Cytochrome P450 3A4 (CYP3A4) is an enzyme responsible for the inactivation of a large number of drugs. Müller et al. constructed a CYP3A4-containing biosensor in which sub-nanometer gold particle-modified G4 PAMAM dendrimers were adsorbed in alternate layers with the enzyme. This biosensor was then used for the detection of caffeine [Citation58]. In a recent study, nanofilms with 4th generation pyridylphenylene dendrimers have been synthesized using proteins. These nanofilms were found to be stable, 230–710 nm thick. Such nanofilms have been reported to serve as promising tools for developing new stable biosensors [Citation59].
Conclusions
It is evident from the above discussion that there is hardly a biomedical and/or biotechnological intervention where dendrimers have not yet been explored. The unique branched structure of dendrimers provides the much needed “large reactive surface area” giving the biomimicry the optimum-co-maximum, functional physicochemical characteristics. The future of the dendritic archetypes lies in conjugated single component systems, such as dendrimers conjugated vaccines and biosensors. Given the above-mentioned surface area, the number of moles of bioactive that can be conjugated to the dendrimer molecule is huge and may provide a cost-effective biotechnology intervention. Such bioactive functionalization may also improve the biocompatibility, biomineralization and tissue regeneration properties of the dendrimers. However, the biodegradability of the dendrimers still remains a major concern with only few studies reporting completely biodegradable dendritic variants. To address this issue to some extent, recent research studies have explored the possibility of using biodegradable linkers to formulate the dendrimer-bioactive conjugate opening new era of possibilities in this dendrimer-based biomimicry and biotechnology.
Disclosure statement
No potential conflict of interest was reported by the authors.
References
- Dutta T, Garg M, Jain NK. Poly(propyleneimine) dendrimer and dendrosome mediated genetic immunization against hepatitis B. Vaccine. 2008;26:3389–3394.
- Abbasi E, Aval SF, Akbarzadeh A, et al. Dendrimers: synthesis, applications, and properties. Nanoscale Res Lett. 2014;9:247.
- Marx V. Poised to branch out. Nat Biotechnol. 2008;26:729–732.
- Paleos CM, Tsiourvas D, Sideratou Z. Triphenylphosphonium decorated liposomes and dendritic polymers: prospective second generation drug delivery systems for targeting mitochondria. Mol Pharmaceutics. 2016;13:2233–2241.
- Hawker CJ, Farrington PJ, Mackay ME, et al. Molecular ball bearings: the unusual melt viscosity behavior of dendritic macromolecules. J Am Chem Soc. 1995;117:4409–4410.
- Serenko O, Strashnov P, Kapustin G, et al. Adsorption properties of pyridylphenylene dendrimers. RSC Adv. 2017;7:7870–7875.
- Boas U, Christensen JB, Heegaard PM. Dendrimers in medicine and biotechnology. Royal Society of Chemistry; 2006. p. 62–70.
- Gupta V, Nayak S. Dendrimers: a review on synthetic approaches. J Appl Pharm Sci. 2015;5:117–122.
- Liu H, Wang H, Yang W, et al. Disulfide cross-linked low generation dendrimers with high gene transfection efficacy, low cytotoxicity, and low cost. J Am Chem Soc. 2012;134:17680–17687.
- Hashempour Alamdari N, Alaei-Beirami M, Sadat Shandiz SA, et al. Gd3+-Asparagine-Anionic linear globular dendrimer second-generation G2 complexes: novel nanobiohybrid theranostics. Contrast Media Mol Imaging. 2017;2017:19.
- Hecht S. Functionalizing the interior of dendrimers: synthetic challenges and applications. Angew Chem Int Ed Engl. 2003;40:74–91.
- Xiangyang S, Kai S, Lajos PB, et al. Synthesis, characterization, and manipulation of dendrimer-stabilized iron sulfide nanoparticles. Nanotechnology. 2006;17:4554.
- Hamilton P, Jacobs D, Rapp B, et al. Surface hydrophobic modification of fifth-generation hydroxyl-terminated poly(amidoamine) dendrimers and its effect on biocompatibility and rheology. Materials (Basel). 2009;2:883–902.
- Augustus EN, Allen ET, Nimibofa A, et al. A review of synthesis, characterization and applications of functionalized dendrimers. Am J Polym Sci. 2017;7:8–14.
- Shcharbin D, Janicka M, Wasiak M, et al. Serum albumins have five sites for binding of cationic dendrimers. Biochim Biophys Acta. 2007;1774:946–951.
- Kojima C, Fukushima D. Applications of gold nanoparticle-loaded thermosensitive elastin-mimetic dendrimer to photothermal therapy. J Photopol Sci Technol. 2016;29:519–523.
- Kasai S, Nagasawa H, Shimamura M, et al. Design and synthesis of antiangiogenic/heparin-binding arginine dendrimer mimicking the surface of endostatin. Bioorg Med Chem Lett. 2002;12:951–954.
- Abdel-Sayed P, Kaeppeli A, Siriwardena T, et al. Anti-microbial dendrimers against multidrug-resistant P. aeruginosa enhance the angiogenic effect of biological burn-wound bandages. Sci Rep. 2016;6:22020.
- Chen H, Banaszak Holl M, Orr BG, et al. Interaction of dendrimers (artificial proteins) with biological hydroxyapatite crystals. J Dent Res. 2003;82:443–448.
- Palmer LC, Newcomb CJ, Kaltz SR, et al. Biomimetic systems for hydroxyapatite mineralization inspired by bone and enamel. Chem Rev. 2008;108:4754–4783.
- Wu D, Yang J, Li J, et al. Hydroxyapatite-anchored dendrimer for in situ remineralization of human tooth enamel. Biomaterials. 2013;34:5036–5047.
- Huysal M, Senel M. Dendrimer functional hydroxyapatite nanoparticles generated by functionalization with siloxane-cored PAMAM dendrons. J Colloid Interface Sci. 2017;500:105–112.
- Kinberger GA, Cai W, Goodman M. Collagen mimetic dendrimers. J Am Chem Soc. 2002;124:15162–15163.
- Kinberger GA, Taulane JP, Goodman M. The design, synthesis, and characterization of a PAMAM-based triple helical collagen mimetic dendrimer. Tetrahedron. 2006;62:5280–5286.
- Zhao X, Sun H, Zhang X, et al. OEGylated collagen mimetic polypeptides with enhanced supramolecular assembly. Polymer. 2016;99(C):281–291.
- Chonco L, Pion M, Vacas E, et al. Carbosilane dendrimer nanotechnology outlines of the broad HIV blocker profile. J Control Release. 2012;161:949–958.
- Habicher T, Diederich F, Gramlich V. Catalytic dendrophanes as enzyme mimics: synthesis, binding properties, micropolarity effect, and catalytic activity of dendritic thiazolio-cyclophanes. HCA. 1999;82:1066–1095.
- Liu L, Breslow R. Dendrimeric pyridoxamine enzyme mimics. J Am Chem Soc. 2003;125:12110–12111.
- Albiter MA, Morales R, Zaera F. Dendrimer-based synthesis of Pt catalysts for hydrocarbon conversion. Appl Catal A. 2011;391:386–393.
- Giacalone F, Campisciano V, Calabrese C, et al. Single-Walled carbon nanotube-polyamidoamine dendrimer hybrids for heterogeneous catalysis. ACS Nano. 2016;10:4627–4636.
- Cromer JR, Wood SJ, Miller KA, et al. Functionalized dendrimers as endotoxin sponges. Bioorg Med Chem Lett. 2005;15:1295–1298.
- Neelgund GM, Oki A, Luo Z. Antimicrobial activity of CdS and Ag2S quantum dots immobilized on poly(amidoamine) grafted carbon nanotubes. Colloids Surf B Biointerfaces. 2012;100:215–221.
- Vacas-Cordoba E, Maly M, De la Mata FJ, et al. Antiviral mechanism of polyanionic carbosilane dendrimers against HIV-1. Int J Nanomedicine. 2016;11:1281–1294.
- Devadoss C, Bharathi P, Moore JS. Energy transfer in dendritic macromolecules: molecular size effects and the role of an energy gradient. J Am Chem Soc. 1996;118:9635–9644.
- Paulo PMR, Costa SMB. Photoinduced electron-transfer in supramolecular complex of zinc porphyrin with poly(amido amine) dendrimer donor. J Photochem Photobiol A Chem. 2012;234:66–74.
- Yang H, Pan S, Ma D, et al. Light-harvesting dendrimer zinc-phthalocyanines chromophores labeled single-wall carbon nanotube nanoensembles: synthesis and photoinduced electron transfer. J Lumin. 2016;179:588–594.
- Kobayashi H, Kawamoto S, Jo SK, et al. Macromolecular MRI contrast agents with small dendrimers: pharmacokinetic differences between sizes and cores. Bioconjugate Chem. 2003;14:388–394.
- Zhou X, Ye M, Han Y, et al. Enhancing MRI of liver metastases with a zwitterionized biodegradable dendritic contrast agent. Biomater Sci. 2017;5:1588–1595.
- Lee JH, Cha KE, Kim MS, et al. Nanosized polyamidoamine (PAMAM) dendrimer-induced apoptosis mediated by mitochondrial dysfunction. Toxicol Lett. 2009;190:202–207.
- Bodewein L, Schmelter F, Di Fiore S, et al. Differences in toxicity of anionic and cationic PAMAM and PPI dendrimers in zebrafish embryos and cancer cell lines. Toxicol Appl Pharmacol. 2016;305:83–92.
- Franiak-Pietryga I, Ziolkowska E, Ziemba B, et al. Glycodendrimer PPI as a potential drug in chronic lymphocytic leukaemia. The influence of glycodendrimer on apoptosis in in vitro B-CLL cells defined by microarrays. Anticancer Agents Med Chem. 2017;17:102–114.
- Siewiera K, Labieniec-Watala M. Ambiguous effect of dendrimer PAMAM G3 on rat heart respiration in a model of an experimental diabetes - objective causes of laboratory misfortune or unpredictable G3 activity? Int J Pharm. 2012;430:258–265.
- Labieniec M, Gabryelak T. Preliminary biological evaluation of poli(amidoamine) (PAMAM) dendrimer G3.5 on selected parameters of rat liver mitochondria. Mitochondrion. 2008;8:305–312.
- Ganda IS, Zhong Q, Hali M, et al. Dendrimer-conjugated peptide vaccine enhances clearance of Chlamydia trachomatis genital infection. Int J Pharm. 2017;527:79–91.
- Gorain B, Tekade M, Kesharwani P, et al. The use of nanoscaffolds and dendrimers in tissue engineering. Drug Discov Today. 2017;22:652–664.
- Liu ZJ, Daftarian P, Kovalski L, et al. Directing and potentiating stem cell-mediated angiogenesis and tissue repair by cell surface E-Selectin coating. PLoS One. 2016;11:e0154053.
- Noriega-Luna B, GodÃ-nez LA, RodrÃ-guez FJ, et al. Applications of dendrimers in drug delivery agents, diagnosis, therapy, and detection. J Nanomater. 2014;2014:39.
- Chan JC, Burugapalli K, Naik H, et al. Amine functionalization of cholecyst-derived extracellular matrix with generation 1 PAMAM dendrimer. Biomacromolecules. 2008;9:528–536.
- Hu Q, Ding B, Yan X, et al. Polyethylene glycol modified PAMAM dendrimer delivery of kartogenin to induce chondrogenic differentiation of mesenchymal stem cells. Nanomedicine. 2017;13:2189–2198.
- Baigude H, Katsuraya K, Okuyama K, et al. Synthesis of structurally-controlled AIDS vaccine model with glyco-peptide dendrimer scaffolds. Macromol Chem Phys. 2004;205:684–691.
- Kalomiraki M, Thermos K, Chaniotakis NA. Dendrimers as tunable vectors of drug delivery systems and biomedical and ocular applications. Int J Nanomedicine. 2015;11:1.
- Zaman M, Skwarczynski M, Malcolm JM, et al. Self-adjuvanting polyacrylic nanoparticulate delivery system for group a streptococcus (GAS) vaccine. Nanomedicine. 2011;7:168–173.
- Mesa-Antunez P, Collado D, Vida Y, et al. Fluorescent BAPAD dendrimeric antigens are efficiently internalized by human dendritic cells. Polymers (Basel). 2016;8:111.
- Vacas-Cordoba E, Climent N, De La Mata FJ, et al. Dendrimers as nonviral vectors in dendritic cell-based immunotherapies against human immunodeficiency virus: steps toward their clinical evaluation. Nanomedicine. 2014;9:2683–702.
- Uygun ZO, Sahin Ã, Yilmaz M, et al. Fullerene-PAMAM (G5) composite modified impedimetric biosensor to detect Fetuin-A in real blood samples. Anal Biochem. 2017;542:11–15.
- Nigam S, Chandra S, Bahadur D. Dendrimers based electrochemical biosensors. Biomed Res J. 2006;2:21–36.
- Zhu N, Gao H, Xu Q, et al. Sensitive impedimetric DNA biosensor with poly(amidoamine) dendrimer covalently attached onto carbon nanotube electronic transducers as the tether for surface confinement of probe DNA. Biosens Bioelectron. 2010;25:1498–1503.
- Muller M, Agarwal N, Kim J. A cytochrome P450 3A4 biosensor based on generation 4.0 PAMAM dendrimers for the detection of caffeine. Biosensors (Basel). 2016;6:44.
- Stroylova Y, Sorokina S, Stroylov V, et al. Spontaneous formation of nanofilms under interaction of 4th generation pyrydylphenylene dendrimer with proteins. Polymer. 2018;137:186–194.
- Eichman JD, Bielinska AU, Kukowska-Latallo JF, et al. The use of PAMAM dendrimers in the efficient transfer of genetic material into cells. Pharm Sci Technolo Today. 2000;3:232–245.
- Sepulveda-Crespo D, Vacas-Cordoba E, MÃrquez-Miranda V, et al. Effect of several HIV antigens simultaneously loaded with G2-NN16 carbosilane dendrimer in the cell uptake and functionality of human dendritic cells. Bioconjug Chem. 2016;27:2844–2849.
- Zhu A, Bharathi P, White JO, et al. Effect of pressure on the emission efficiencies of a series of phenylacetylene dendrimers. Macromolecules. 2001;34:4606–4609.
- Duan X, McLaughlin C, Griffith M, et al. Biofunctionalization of collagen for improved biological response: scaffolds for corneal tissue engineering. Biomaterials. 2007;28:78–88.
- Oliveira JM, Kotobuki N, Marques AP, et al. Surface engineered carboxymethylchitosan/poly(amidoamine) dendrimer nanoparticles for intracellular targeting. Adv Funct Mater. 2008;18:1840–1853.
- Boduch-Lee KA, Chapman T, Petricca SE, et al. Design and synthesis of hydroxyapatite composites containing an mPEG dendritic poly(l-lysine) star polycaprolactone. Macromolecules. 2004;37:8959–8966.
- Alonso B, Armada PG, Losada J, et al. Amperometric enzyme electrodes for aerobic and anaerobic glucose monitoring prepared by glucose oxidase immobilized in mixed ferrocene-cobaltocenium dendrimers. Biosens Bioelectron. 2004;19:1617–1625.
- Armada MP, Losada J, Zamora M, et al. Electrocatalytical properties of polymethylferrocenyl dendrimers and their applications in biosensing. Bioelectrochemistry. 2006;69:65–73.