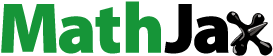
Abstract
Delivery of therapeutic molecules into the deeper ocular compartments is mainly hampered by short precorneal residence and limited transmembrane permeability of topically administered drugs. Hence, the current study was undertaken to fabricate the ion-sensitive in situ gels of natamycin (NT) bilosomes (NB) for efficient ocular delivery. The effect of cholesterol and sodium taurocholate proportion on the properties of the bilosomes were studied and the formulation with better physicochemical properties was optimized and utilized to derive the in situ gelling system (IG). The impact of type/composition of gelling agent on the formation and characteristics of the hydrogel was investigated. The hydrogel formed from IG with 0.3% w/v gellan gum showed optimal viscoelastic and adhesive characteristics. The ocular safety and cytocompatibility of NB and its IG was confirmed by corneal histology and in vitro cytotoxicity evaluation. A 6- to 9-fold enhancement in the transcorneal flux of NB demonstrated efficient ocular penetration of bilosomes. Moreover, the superior mean dose normalized NT levels in the ocular tissues of rabbits treated with optimized NB and IG illustrated the effectiveness of bilosomes loaded ion-sensitive in situ hydrogels as a potential platform for the improved and prolonged ocular pharmacotherapy.
Introduction
Despite advancement in the field of pharmaceutical technology, effective topical ocular drug delivery is still a complicated task for formulation scientists due to the complex anatomical, physiological and formulation constraints [Citation1–3]. Beside various protective ocular barriers, short pre-corneal residence and poor corneal permeability are the key factors contributing to low ocular bioavailability (< 5%) of the therapeutics instilled as conventional ophthalmic dosage forms [Citation2–5]. The increased lacrimation, reflux blinking and nasolacrimal drainage accounts for the rapid elimination of the drugs from the cul-de-sac [Citation2,Citation3]. Various formulations like ocular ointments, hydrogels, polymeric or ion-exchange resin-based topical ocular inserts and in situ gel systems have been reported to improve the residence of the drugs in the pre-corneal space [Citation6–9]. In situ hydrogels have better patient compliance unlike ocular ointments and inserts which causes blurred vision and grittiness influenced distressed blinking [Citation8,Citation10,Citation11]. The in situ gel systems are solutions that undergo phase transition to form viscoelastic hydrogel in the ocular milieu in response to the temperature, pH, electrolytes or ions and photo radiation [Citation8,Citation10,Citation11]. Due to the abundance of electrolytes in tear fluid, the ion or electrolyte sensitive in situ hydrogel formation is triggered at lower polymer concentrations with respect to that induced by other stimuli [Citation12,Citation13].
The distinct anatomical features of the cornea, with hydrophilic stroma sandwiched between lipophilic epithelium and endothelium, restricts the penetration of hydrophilic and lipophilic drugs into the deeper ocular tissues [Citation2–4,Citation14]. Numerous lipid/polymeric nano-formulations such as solid lipid nanoparticles (SLN), nanostructured lipid carriers (NLC), polymeric nanoparticles (PN), micelles and phospholipid or non-ionic surfactant-based vesicles have shown success in enhancing the transcorneal drug permeability [Citation16–20]. The combination of in situ gel systems and nanocarriers offers improved tear/corneal surface contact time and corneal permeability of the drugs [Citation21]. Several pH and/or temperature triggered in situ gel forming systems of SLN, polymeric nanoparticles, nanoemulsions, liposomes and niosomes have been successfully investigated as suitable ocular drug delivery platforms [Citation21–25].
In recent years, bilosomes, the ultra-deformable and flexible vesicles, have emerged as potential carriers for improved oral and transdermal delivery of small and large molecules [Citation26,Citation27]. The inclusion of the amphiphilic bile salt in the vesicle bilayer allows bilosomes to penetrate through the intercellular lipid layers of intact biological membranes, such as skin and intestine [Citation26,Citation27]. Considering this advantage, a recent study investigated the suitability of bilosomes for improved ex vivo corneal permeability of a lipophilic molecule [Citation28]. To the best of our knowledge, bilosomes in ion sensitive in situ hydrogel-forming systems have not been investigated till date.
In this article, we report the fabrication and evaluation of ion-sensitive in situ hydrogels of natamycin (NT)-loaded bilosomes (NB) for improved ocular pharmacotherapy. NT, a macrolide polyene antifungal agent, is the only commercially available ophthalmic product (Natacyn® (NT 5% w/v suspension)) approved by US-FDA for treating fungal keratitis and other fungal infections [Citation29]. Due to poor aqueous solubility, limited corneal permeability and low precorneal residence time of NT, current therapy requires multiple dosing (a drop every 1–2 h) for several weeks (4–6 weeks) to attain and/or maintain optimal concentrations at the desired site [Citation29,Citation30]. Hence, there is a need to develop a delivery system, with improved NT corneal permeability and prolonged drug levels in the ocular sites, for improved patient compliance. A few literature reports described improved NT levels in the tear and prolonged in vitro corneal drug permeability from mucoadhesive nanoparticles and niosomes thermosensitive in situ gels, respectively [Citation25,Citation31]. However, there are no reports on the ocular disposition of NT or any other drug from topically instilled bilosomes in situ gels.
Thus, for the first time, the ocular disposition of ion-sensitive in situ hydrogel-forming system of NB is evaluated in the New Zealand male albino rabbits.
Materials and methods
NT was procured from Cayman Chemical (Ann Arbor, MI). Sodium taurocholate (ST) was obtained from Biosynth International, Inc. (Itasca, IL). Sorbitan monostearate (Span 60 (S60)) and MTT reagent (methylthiazolyldiphenyl-tetrazolium bromide) were received from Sigma Aldrich (St. Louis, MO. Cholesterol (CH) was procured from Alfa Aesar (Ward Hill, MA). Gelan gum (G) and xanthum gum (X) were purchased from MP Biomedicals, LLC (Santa Ana, CA). DMEM/F-12 (Dulbecco's modified Eagle medium/nutrient mixture F-12) was procured from Mediatech, Inc. (A Corning Subsidiary Manassas, VA). Glycerin, Amicon® Ultracentrifugal filter devices with regenerated cellulose membrane (molecular weight cut off 100 kDa), high-performance liquid chromatography (HPLC)-grade solvents, and other chemicals (analytical grade) were supplied by Fisher Scientific (Hampton, NH). Whole eyes of male albino New Zealand rabbits were obtained from Pel-Freez Biologicals (Rogers, AR). Male albino New Zealand rabbits were procured from Harlan Labs (Indianapolis, IN).
Quantification of natamycin
The NT content in all the samples was determined with HPLC-UV (ultraviolet) method, as reported earlier, with minor modifications [Citation32]. In brief, a mobile phase of acetonitrile and 0.02 M potassium dihydrogen phosphate (30:70% v/v) mixture (pH 5.5) was pumped through a phenomenex Luna® C18(2) 100 Å column (4.6 mm × 250 mm; 5 µ particle size), at a flow rate of 1.0 ml/min, by Water 600 controller pump in HPLC system. A 20 µL sample was injected by Waters 717 plus Autosampler into the mobile phase and the column eluent was monitored at λmax (detection wavelength) of 304 nm using Waters 2487 Dual λ Absorbance detector. The absorbance peaks were recorded with the help of Agilent 3395 Integrator.
Preparation of natamycin bilosomes and in situ gel formulations
The film hydration technique was employed to prepare the natamycin bilosomes (NB) [Citation23,Citation33]. Briefly, in 20 ml of organic solvent mixture of chloroform, ethanol and methanol (7:1:2), accurately weighed quantities of lipid mixture (210 or 230 µM of S60 and CH taken in 2:1, 1:1 and 1:2 molar ratios) and drug were dissolved in a round bottom (RB) flask. The RB flask was then mounted onto a rotary flash evaporator (Laborota 4000, Heidolph, Germany) operated at 200 rpm for 30 min at 45 ± 5 °C to evaporate the organic solvent, under vacuum, leaving a thin film of lipid on the wall of the flask. The lipid film was dried in vacuum oven at 40 °C for 2 h and then hydrated with the deionized water (8–10 ml) containing ST (20 or 40 µM) and glycerin, maintained at 60 ± 5 °C, to form NB with vigorous shaking. Further, the NB was sonicated, for size reduction, with probe sonicator (Vibra-Cell™ Ultrasonic, Sonics and Material INC., CT) for 5 min with pulse on for 20 s and off for 40 s at 30% amplitude.
The NB in situ gel NBG or NBX formulations were obtained by adding 8 ml of NB 2 to 2 ml of G or X dissolved in deionized water (60 ± 5 °C). The formulations were allowed to come to room temperature and stored in screw-capped glass vials until further evaluation. The composition of the NB and NBG or NBX formulations are presented in .
Table 1. Composition and physicochemical properties of natamycin bilosomes (mean ± SD; n = 3).
Particle size, zeta potential and polydispersity index measurement
The mean particle size (PS) and polydispersity index (PDI) of the NB, NBG and NBX formulations were determined by dynamic light scattering using Zetasizer Nano ZS Zen3600 (Malvern Instruments, Inc. UK). Samples were suitably diluted with filtered double-distilled water (0.2 μm nylon filter) and analysis was performed at 25 °C and 173° angle of detection using a helium-neon laser of 633 nm, in disposable clear cells. Zeta potential (ZP) measurements were also carried out at 25 °C using the laser Doppler velocimetry function of the same instrument.
Drug content and entrapment efficiency
The NT content was determined by extracting the NT from the formulations using methanol and 190-proof alcohol (70:30%v/v) then centrifuged (AccuSpin 17 R centrifuge, Fisher Scientific, Hampton, NH) at 13,000 rpm for 20 min. The supernatant was separated, appropriately diluted and quantified for the drug content by HPLC.
The percentage drug entrapment efficiency (% EE) was determined by estimating the free NT concentration in the dispersion medium using ultra-filtration technique. In brief, 500 μL of each formulation was added to the sample reservoir of the Amicon Ultra-centrifugal filter device (Molecular weight cutoff of 100 kilo Daltons) (Fisher Scientific) and centrifuged at 5000 rpm for 10 min (AccuSpin 17 R centrifuge, Fisher Scientific). The NT content in the aqueous filtrate was evaluated by HPLC. The % EE was calculated from the EquationEquation (1)(1)
(1) . The experiment was carried out in triplicate.
(1)
(1)
where Wi = total drug content, and Wf = amount of free drug in aqueous phase.
In vitro gelation behavior of NB in situ gels
The gelling capacity of bilosomes in situ gel formulations (NBG or NBX) was visually evaluated by determining the gel formation time (GT) and residence time (GRT) of intact gel without dissolution or breaking [Citation25,Citation34]. Briefly, 50 µL of NBG or NBX formulation was dropped into a glass vial with 2 ml of freshly prepared simulated tear fluid (STF) and maintained at 34 °C in a shaking water bath (Precision™, Fisher Scientific) operated at 100 rpm for 12 h (Supplementary Table 1). The time taken for the transformation of sol-to-gel by NBG or NBX formulations was recorded as the gel formation time (GT). Each vial was visually inspected every hour and the time required for the gel to dissolve or break was recorded as the intact gel residence time (GRT).
Rheological studies
The viscosity of the NB 2, NBG and NBX formulations were measured on Brookfield cone and plate viscometer (LVDV-II + Pro Viscometer, Middleboro, MA). Briefly, 500 µL of each formulation was placed in the cup plate, and the gap between cone and plate was adjusted. The samples in the cup were maintained at 25 °C using a circulating water bath. A CP-40 Cone/Spindle was operated at 0.1, 1 and 10 rpm and the viscosity was recorded from Rheocalc software. To determine viscosity of the hydrogels, 500 µL of mixture of NBG/NBX formulation/s and STF (50:7) was placed in cup plate and similar procedure was repeated to record viscosity (n = 3) [Citation23].
Texture analysis
The adhesive strength of hydrogels obtained from NBX and NBG formulations were evaluated with TA.XT2i texture analyzer (Texture Technologies Corp. NY) set to the compression mode [Citation35]. The hydrogels were placed in soft matter holder (TA-275) positioned below the acrylic cylindrical probe (1-in. diameter (TA-3)) which was then lowered into the product (1 mm distance from the surface) at a test-speed of 0.5 mm/s with 3 g trigger force. An acquisition rate of 500 points/sec was selected and the texture analysis was conducted at room temperature (n = 3).
Transmission electron microscopy
The morphology of bilosomes in the NB 2 formulation was evaluated by transmission electron microscopy (TEM) (JEOL JEM1200EX II electron microscope) as per the published protocol with minor modifications [Citation36]. Briefly, NB 2 was centrifuged for 1 h at 25,000 rpm and 15 °C (SORVALL, WX Ultra Series Centrifuge, Waltham, MA) to separate the bilosomes pellet which was then resuspended in 5 ml deionized water and recentrifuged (as mentioned earlier). This step is repeated three times to wash out any soluble impurities. The resuspended bilosomes was centrifuged for 5 min at 2000 rpm (15 °C) (Eppendorf AG centrifuge, Hamburg, Germany), to seperate the aggregates/insoluble impurities, and the clean vesicular suspension was then diluted 1:100 with deionized water. Two microlitres of the diluted suspension was placed on 400 mesh copper grids covered with Formvar film (Electron Microscopy Sciences EMS, Hatfield, PA). The grids were allowed to dry over night in a desiccator and then examined by TEM.
Fourier transform infrared spectroscopy (FTIR)
The infrared spectra of drug, sodium taurocholate, cholesterol, span 60 and NBG 2 formulation samples were recorded using Cary 660 series FTIR (Agilent Technologies, Santa Clara, CA) to understand the chemical compatibility between the drug and excipients.
Stability studies
The NB 2 and NBG 2 formulations were taken in glass vials, wrapped in aluminum foil and stored at 4 °C and 25 °C for 60 days. Their physical and chemical stability, on storage, were evaluated by withdrawing the samples at definite time intervals (0, 30 and 60 days) and analyzing the particle size, PDI, zeta potential, % EE and drug content as described earlier.
In vitro cytotoxicity
In vitro cytotoxicity of NB 2 and NBG 2 formulations were evaluated by the methyl thiazolyl tetrazolium (MTT) assay. Normal saline and triton X 100 served as negative and positive controls for the experiment respectively. The assay was carried out in 96-well plates (Costar 3596, Corning Inc., Corning, NY). Briefly, 100 μl of the human corneal limbal epithelial (HCLE) cells were plated at a concentration of 15 × 104 cell/mL and incubated in an incubator at 37 °C with 5% CO2 for 24 h in culture medium (DMEM/F-12 50:50, with 10% calf serum, and 1% penicillin/streptomycin). Then, 100 μl of the diluted formulations, negative control (normal saline), NB 2, NBG 2 and positive control (triton X 100)) (i.e. 50 μl formulation +50 μl of DMEM/F-12 50:50 medium) was added to each well. After 24-h incubation, the formulations were removed, and the cells were washed twice with the culture medium to remove all traces of the formulations. Hundred microlitres of MTT reagent (1 mg/ml in culture medium without serum) was added to each well and the plates were incubated at 37 °C for 4 h. During the incubation, the active enzymes (mitochondrial reductase) of the viable cells converted the yellow MTT dye into purple formazan crystals. After incubation, the medium was removed and 100 μl DMSO was added to each well to dissolve the formazan crystals and the intensity of color was assessed at 570 nm with µ-Quant universal microplate spectrophotometer (Bio-Tek Instruments, Inc. Winooski, VT).
In vitro corneal permeation studies
In vitro transcorneal permeation studies were performed in the corneas separated from rabbit whole eyes shipped overnight in Hanks balanced salt solution (over wet ice) from Pel-Freez Biologicals. They were used immediately upon receipt. To confirm the secure mounting between the donor and receiver chambers of diffusion cell during the study period, corneas were excised with some scleral portion. They were placed on Valia-Chien cells (PermeGear, Inc®), maintained at 34 °C, with the endothelial side facing receiver chamber while the epithelial side facing the donor chamber. 200 µL of control (Natacyn®), NB 2 and NBG 2 formulations were added to the individual donor chambers. 50 µL of DPBS was placed in the donor chamber holding 200 µL of NBG 2 formulation to ensure the hydrogel formation. The Natacyn® suspension was diluted to obtain 0.1% w/v NT, equivalent to that in NB 2 and NBG 2 formulations. The receiver chamber was filled with 5 ml of DPBS (pH 7.4) with 2.5% w/v of RMβCD (random methylated β cyclodextrin) medium. At predetermined time points, an aliquot of 600 µL was withdrawn from the receiver chamber and replenished with equal volume of fresh medium to maintain the constant volume, until 3 h. Samples were stored at −20 °C until further analysis by HPLC.
Data analysis
The transcorneal permeation parameters across rabbit cornea such as the cumulative amount of drug permeated (Mn), steady-state flux (J) and the apparent permeability coefficient (Papp) were calculated as per the earlier reports [Citation37]. The cumulative amount of NT permeated across the cornea was determined using the equation EquationEquation (2)(2)
(2) .
(2)
(2)
where: n indicates sampling time point (n = 1, 2, 3….8 corresponding to 15, 30, 45….180 min respectively); Vr and VS represents the volume of the medium in the receiver chamber (mL) and the volume of the sample collected at the nth time point (mL); and Cr(n) specifies the concentration of the drug in the receiver chamber medium at nth time point (µg/mL).
The rate of NT transported through the rabbit cornea was computed from the slope of the cumulative amount of NT permeated versus time (t) plot. The steady-state flux (SSF) (J) of natamycin was calculated according to the following equation (EquationEquation (3)(3)
(3) ) [Citation16]:
(3)
(3)
where M and A designates the cumulative amount of drug transported and the surface area of the cornea utilized in the experiment (0.636 cm2).
The transcorneal permeability of NT was determined from the ratio of steady-state flux (J) and amount of drug taken in the donor chamber (Cd) (EquationEquation (4)(4)
(4) ) [Citation16,Citation37].
(4)
(4)
Further, the enhancement ratio (ER) was determined from the ratio of flux (J) of natamycin from bilosomes (NB 2) or bilosome in situ gel (NBG 2) formulation and control formulation.
Corneal histology studies
The corneal histological evaluation was performed on the corneas utilized in the in vitro transcorneal permeation studies. At the end of the study, corneas were fixed with 4% paraformaldehyde for 24 h, embedded in paraffin wax and were sliced into 5-μm cross sections using a microtome (American Optical® 820 Rotary Microtome). The tissue sections were mounted on a slide and dried overnight in an oven. The slide was washed with xylene to remove the paraffin and the tissue was hydrated by washing with alcohol and water. The tissue was stained with nuclear dye Gill III hematoxylin (StatLab medical) by rinsing for 10 min and later counterstained with eosin. Finally, these slides were washed in running water, alcohol and xylene. A cover slip was placed on the tissue section, examined under Nikon Eclipse 800 microscope and images were captured using PictureFrame 3.0 software (Optronics) to observe morphological changes in cornea, if any.
Ocular distribution study
The ocular distribution study was carried out in conscious male New Zealand albino rabbits. Prior to the study, the animals were allowed free access to food and water. The experiment was performed according to the approved protocols of the University of Mississippi Institutional Animal Care and Use committee and Association for Research in Vision and Ophthalmology (ARVO) statement. The rabbits were divided into three groups based on the formulation they were treated with and represented as control (Natacyn®), NB 2 and NBG 2 groups. Fifty microlitres of Natacyn® or NB 2 or NBG 2 formulation, with the help of micropippette, was topically administered in the cul-de-sac of the right eye of each rabbit in the corresponding group. At the end of 2-h and 6-h post-treatment, rabbits (n = 4) were anesthetized with intramuscular injection of ketamine and xylazine combination and euthanized with an excess amount of pentobarbital injected through a marginal ear vein. The treated eye was washed thoroughly with ice cold IPBS and enucleated immediately. The ocular tissues were carefully separated, weighed and stored at −80 °C until analysis.
NT extraction from ocular tissue matrix
Protein precipitation technique was employed for the extraction of NT from the ocular tissue matrix. Briefly, tissues such as cornea, sclera, iris ciliary (IC), retina-choroid (RC) were taken in separate Eppendorf tubes and cut into small pieces followed by the addition of 1 ml ice-cold methanol with 0.1% w/v formic acid to precipitate tissue proteins. The proteins in the aqueous humor (AH) (200 µL) and vitreous humor (VH) (500 µL) were precipitated by adding 200 µL and 500 µL of ice-cold acetonitrile with 0.1% w/v formic acid, respectively. All the Eppendorf tubes were vortexed for 30 s, allowed to stand at room temperature for 15 min and kept under sonication in bath sonicator (Fisher Scientific) for 10 min to extract the drug into solvent. These samples were centrifuged at 13,000 rpm for 30 min, the supernatant was collected in the separate vials and stored at −80 °C until the analysis. The NT content in the supernatant solutions were estimated by injecting an aliquot of 100 µL into the HPLC operated with the chromatographic conditions described earlier [Citation32]. The column eluent was monitored at λmax (detection wavelength) of 304 nm using Waters 2487 Dual λ absorbance detector, and the sensitivity was set at 0.05 AUFS at room temperature. The NT was quantified using standard calibration plots constructed with the concentration range of interest in various ocular tissues. The limit of detection and quantification in various ocular tissues were 10 and 20 ng/mL, respectively. The standard curves in all the ocular tissues were linear with a coefficient of determination (r2) ≥ 0.95.
Statistical analysis
The data obtained were subjected to one-way analysis of variance (ANOVA) and the statistical significance of difference between formulations was calculated by Tukey’s post hoc HSD (version 5.00; GraphPad Software, San Diego California). A “p” value less than .05 was set to represent the statistically significant difference.
Results and discussion
Physicochemical properties of NB
NB were successfully fabricated by thin-film hydration technique coupled with probe sonication. The physicochemical properties of bilosomes such as particle size (PS), polydispersity index (PDI), zeta potential (ZP) and drug entrapment (% EE) are dependent on the proportion of S60-to-CH and the bile salt (ST) [Citation28,Citation38]. Hence, in the present study, the influence of cholesterol and the bile salt on these parameters were investigated by varying the S60-to-CH molar ratio and ST quantity, respectively, keeping overall lipid content 250 µM. Thus, the loading capacity (ratio of entrapped drug and total lipid weight) of the NT in bilosomes in all formulations was 8.8%. The NT content in all the NB formulations (pH 6.2–7.1) was in the range of 90.1 ± 7.2% to 97.9 ± 4.1% (). The NB formulations showed PS ranging from 235.03 ± 05.29 nm to 380.43 ± 27.94 nm with a PDI of 0.20 ± 0.01 to 0.43 ± 0.23, ZP of −45.41 ± 4.54 mV to −69.03 ± 1.57 mV and % EE of 70.12 ± 3.66% to 93.75 ± 4.32% ().
Table 2. Composition and characterization of NB in situ hydrogel formulations (mean ± SD; n = 3).
Effect of cholesterol
The PS and % EE of NT in bilosomes appeared to be dependent on the CH composition. It was noticed that the PS of bilosomes decreased as the CH content increased in the formulations (NB 1 to NB 2 or NB 4 to NB 5) (). Generally, CH interacts with the hydrophobic tails of the lipids in the vesicle bilayer and controls the packing and rigidity of the vesicles [Citation39]. Thus, the decrease in PS can be attributed to the increased interactions of CH and S60 leading to close packing of the bilayers in the bilosomes. The % EE increased proportionately with the CH concentration in the formulations NB 1 to NB 2 or NB 4 to NB 5 suggesting effective intercalation of NT within the bilayer with increased hydrophobic interactions and reduced leakage of drug from rigid bilosomes () [Citation39,Citation40]. Interestingly, further increase in the CH concentration in the formulations (NB 3 or NB 6) resulted in higher PS and lower % EE (). This can be attributed to the perturbation of bilayer structure by the accommodation of excess cholesterol and thereby leading to the expulsion of drug from the vesicles [Citation38,Citation40].
Effect of bile salt
Consistent with the earlier reports, the PS, PDI, ZP and % EE of the bilosomes were influenced by the amount of bile salt [Citation26,Citation28,Citation41]. With a steroid-like structure, ST, a salt of taurocholic acid (bile acid), resides in the bilayer of the bilosomes imparting negative ZP due to its anionic nature [Citation28,Citation41]. The PS, PDI and ZP of the formulations with 40 µM (21 mg) ST (NB 4–NB 6) were higher compared to the bilosomes with 20 µM (10 mg) ST (NB 1–NB 3), at similar molar ratio of S60 and CH (). These results corroborate with earlier literature reports which demonstrated the increased PS and PDI because of the repulsion between the bilayers of the vesicles due to the greater negative charge (ZP) at higher concentration of ST [Citation42]. Moreover, the accommodation of extra ST in the bilayers can increase the bulkiness of bilosomes resulting in increased particle size and the excess of amount of ST forms micelles in the dispersion medium thereby resulting in increased PDI [Citation43,Citation44]. Strikingly, the %EE of NT in the bilosomes, with same molar ratio of S60 and CH, decreased with the increased ST quantity (). This drop in %EE, at higher concentration of ST, is attributed to the leakage of the entrapped drug from the bilosomes owing to the fluidization of vesicle bilayer by bile salt micelles [Citation28,Citation44].
The NB 2 formulation showed uniformly distributed bilosomes (235.0 ± 05.2 nm) in the colloidal dispersion (PDI: 0.20 ± 0.01) with negative ZP (−47.0 ± 1.5 mV), and higher % EE (93.7 ± 4.3%). Additionally, TEM image revealed layered nanoparticles confirming the vesicular structure of the bilosomes in the NB 2 formulation (). Hence, this formulation was optimized and used in further investigations and for the preparation of in situ gel formulations.
Evaluation of NB in situ gel formulations
The NB in situ hydrogel systems (NBG/NBX) were derived by introducing the NB 2 formulation into the solution comprising gellan gum/xanthum gum, anionic polysaccharide polymers, as ion sensitive gelling agents. The physiochemical properties of bilosomes in the NBG and NBX formulations were similar to NB 2 preparation confirming no interactions of gelling agents with bilosomes (Supplementary Table 3). Several literature reports have successfully demonstrated the formulation of nanoparticles or nanoemulsion loaded in situ gels using these gelling agents as they crosslink in the presence of electrolytes to form hydrogel matrix with higher pore size facilitating the movement of nanoparticles through the gel [Citation12,Citation21–25]. The tested concentrations of gelling agents were below the limits specified in the inactive ingredients database from US FDA [Citation45]. Owing to the thickening property of gellan gum and xanthum gum, it is essential to measure the viscosity of the in situ gel preparations as it determines the free flow of the formulation from the container ensuring the ease of administration. A viscosity of 25–50 cP is most common for ophthalmic solutions [Citation46]. The formation, residence time, viscoelastic behavior and adhesive property of the hydrogels are critical parameters in deciding the pre-corneal residence and shear thinning pseudoplastic behavior for comfort in the eye while blinking [Citation13,Citation21,Citation23]. However, these parameters depend on the kind of gelling agent. Hence, this study focused on the influence of the two most commonly used ion sensitive gelling agents on the critical properties of in situ gel formulations.
Impact of type of gelling agent
The evaluation parameters of the NBG and NBX formulations are represented in the . The viscosity of the formulations (NBG or NBX) increased with the concentration of gellan or xanthan gum confirming their thickening property (). At any given concentration of gelling agent, the viscosity of the NBG formulations were lower compared to that observed in NBX formulations (). These results were in accordance with the previous reports explaining the higher viscosity of the xanthan gum due to the formation of helical structures via hydrogen bonding resulting in the immobilization of water [Citation47]. Additionally, the increased intermolecular accumulation of polymer chains because of the high molecular weight of xanthan gum (∼ 2000 K Daltons) could be the reason for high viscosity of NBX systems [Citation48]. Conversely, the lower viscosity of the gellan gum formulations (NBG) can be attributed to the formation of weak double helices associated with the vander Waal forces [Citation23]. The NBG formulations immediately (< 5 s) underwent in situ phase transition and formed hydrogels, while the NBX formulations slowly (≥20 s) formed in situ hydrogels in the STF suggesting the slow or poor interaction of electrolytes with the xanthan gum compared to the gellan gum [Citation23] (). The NB hydrogels were opaque, initially, and appeared translucent as the time progress due to the release of vesicles from the gel matrix (Supplementary Figure 2). The intact gel residence time and the viscosity of the hydrogels increased with the increasing proportion of the gelling agent (). However, at a given concertation of the gelling agent, these properties were higher for the hydrogels formed from NBG formulations compared to that from NBX preparations (). Similar findings have been reported in the literature where higher viscosities/stiffness of the gellan gum based in situ gels was due to the cross-linking of the polymer chain by the mono/divalent cation mediated aggregates of double helices [Citation49]. In contrast, the lower viscosity/intactness of the hydrogel formed from the NBX formulations can be attributed to the presence of the trisaccharide side chain in the branched structure of xanthan gum which avoids the cation triggered helix formation leading to low or no cross linking of the polymer [Citation12]. The adhesiveness of the in situ hydrogel is an important factor that determines its contact with the corneal or scleral surface [Citation24]. In this study, the work adhesion of hydrogels was measured to understand their adhesive characteristics. The work of adhesion increased with respect to the concentration of gel-forming agent in NBX but similar trend in NBG formulations was seen up to the gellan gum concentration of 0.3% w/v. This might be due to the formation of stiff gel with poor shear thinning behavior, at higher proportion of gellan gum (0.4%), which could lead to discomfort in the eye [Citation23]. At a given concentration of gelling agent, it was noticed that the work of adhesion of hydrogels from NBG formulations was high over that from NBX formulations (). These findings agree with the measured viscosities of the hydrogels and could be attributed to the structure related differences in cross-linking of the polymer chains of gellan and xanthan gum [Citation23,Citation47].
The NBG 2 formulation showed acceptable viscosity (37.54 ± 2.41 cp), immediate transition into a hydrogel in the STF which remained intact for 6–8 h with a viscosity of 514.9 ± 3.87 cP and superior adhesive property (1.39 ± 0.006 g/sec) was selected as suitable NB in situ gel system and investigated further. Layered nanoparticles seen in the STF with hydrogel suggested that the in situ gels system did not alter morphometric properties of bilosomes (Supplementary Figure 1).
Fourier transmission infrared spectroscopy (FTIR)
The FTIR spectra of NT, NBG 2 formulation and other excipients are portrayed in . The existence of signature functional group stretching of NT at 3260 cm−1 (NH+3 deformation), 1713 cm−1 (-C = O), 1567 cm−1 (-CH = CH-) and 1266 cm−1 (-C-O-C-) in the FTIR spectra of NBG 2 formulation suggested no drug-excipient chemical interactions. However, the slight shift in the IR bands of NT in NBG 2 spectra could be due to the transformation in NT physical state, as confirmed from the differential scanning calorimetry (DSC) study (Supplementary Figure 2) ().
In vitro cytotoxicity
The cytotoxicity of NB 2 and NBG 2 formulations in HCLE cells is presented in . About 100.5 ± 4.5 and 110.7 ± 5.6% cell viability was noticed for NB 2 and NBG 2 groups, respectively, which were not statistically different from negeative control (saline) (). These results suggest that both optimized formulations are well tolerated by corneal epithelial cells and can be explored as a suitable vehicle for topical ocular drug delivery. Similar literature reports discussed no ocular cytotoxicity with the vesicular systems containing nonionic surfactants (S60) and/or ST [Citation28,Citation38]. In addition, a study outlined no corneal cytotoxicity with the liposomes containing the sodium taurocholate, whereas formulations containing sodium deoxycholate were toxic to HCE cells [Citation41]. The validity of experimental conditions was confirmed by the positive control (Triton X 100 1%), which showed lower % cell viability (6.9 ± 2.5%) ().
Corneal transport studies
The saturation solubility of NT was in the following order: DPBS (2.5% w/v RMβCD) > DPBS ≥ IPBS > water (Supplementary Table 2). Hence, to maintain sink, DPBS (2.5% w/v RMβCD) was utilized as the release medium in the in vitro corneal transport studies. The transcorneal permeability parameters of NT from control, NB 2 and NBG 2 formulations are detailed in . A significant improvement in the transcorneal flux of drug was observed from NB 2 and NBG 2 over that from control suspension (p < .05) (). An ER of 9.01 ± 5.67 and 6.37 ± 2.81-fold transcorneal flux of NT from NB 2 and NBG 2 formulations, respectively, indicated their potential to improve the corneal transport of NT (). Furthermore, a significantly higher apparent permeability coefficient (Papp) of NT from NB 2 and NBG 2, compared to control, could be attributed to the improved permeability of bilosomes (p < .05) (). These findings verify the previous literature reports, which described the penetration of bilosomes through intact cornea owing to the fluidization of corneal lipids [Citation28,Citation41]. In addition, the superior flexibility of the vesicles with bile salt enables them to permeate across the intact biological membranes [Citation26–28]. The lower transcornal flux and Papp of NT from NBG 2, compared to NB 2, were consistent with the earlier reports which suggested the slower release of drug loaded nanoparticles or vesicles from the in situ hydrogel matrix (p < .05) () [Citation22,Citation25].
Table 3. In vitro transcorneal permeability parameters of natamycin from bilosomes and bilosomes in situ gel formulations (mean ± SD; n = 3).
Table 4. The amount of natamycin in the ocular tissues of rabbits at the end of 2 h and 6 h post-topical administration with control, NB 2 and NBG 2 formulations (mean ± SEM; n = 4).
Corneal histology
Corneal histology studies were carried out to access the corneal tissue architecture after the treatment with NB 2 and NBG 2 formulations. The histology of corneas exposed to DPBS, marketed suspension, NB 2 and NBG 2 formulations in the in vitro transcorneal permeation studies are portrayed in . Normal anatomy of an intact cornea represents epithelial layer (EP) and stroma layer (SL) separated by Bowman’s membrane (BM), and Descemet’s membrane (DM) that separates the stroma from endothelial layer. Any observations like tear or loss, degeneration or disappearance of epithelial layer, separation of Bowman’s membrane from epithelial and stroma layers, vacuoles in the stroma layer confirms the damage of corneal structure. In this study, none of the corneas showed any such signs, except the absence of endothelial layer which was accidentally lost while processing, suggesting no alterations in the corneal tissue architecture during the in vitro transcorneal permeability studies (). Moreover, the corneas exposed to NB 2 and NBG 2 formulations were not structurally different from those exposed to control marketed suspension and DPBS () suggesting the corneal compatibility of NB 2 and NBG 2 bilosome formulations. Similar findings were obtained in the literature which indicated the safety of ST and/or nonionic surfactant based vesicles to ocular sites [Citation23,Citation38,Citation41].
Figure 4. Histology images of rabbit corneas exposed to (A) DPBS, (B) control, (C) natamycin bilosomes (NB 2) and (D) natamycin bilosomes in situ gel (NBG 2) formulations. 1 and 2 indicates the 10 × and 100 × magnifications respectively. EP: epithelial layer; BM: Bowman's membrane; SL: stromal layer; DM: Descemet's membrane.
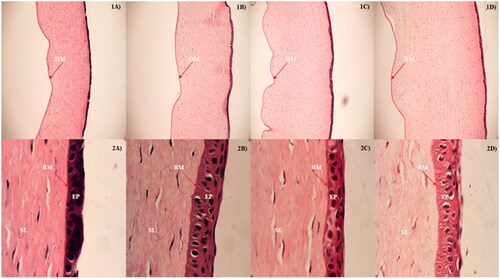
Ocular disposition studies
Since the performance of pharmaceutical product depends on the drug and other excipients in it, undiluted Natacyn suspension (5% w/v natamycin) was used, to exclude the underestimation of the product efficacy, as a control formulation in the ocular distribution studies. The amount of drug observed in the ocular tissues of the rabbits at the end of 2 h and 6 h post treatment with control, NB 2 and NBG 2 formulations are given in . To understand the permeability and prolong delivery of NT from control, NB 2 and NBG 2 formulations, the mean dose normalized drug levels in the rabbit ocular tissues were calculated and presented in .
Figure 5. Mean dose normalized natamycin levels in rabbit ocular tissues from control, optimized natamycin bilosomes (NB 2) and bilosomes in situ gel (NBG 2) formulations, at (A) 2 h and (B) 6 h time point (mean ± SEM; n = 4). *– significantly different at p < .05. ND-not detected; Cr-Cornea; AH-Aqueous Humor; IC-Iris Ciliary Body; SC-Sclera; RC-Retina Choroid; VH-Vitreous Humor.
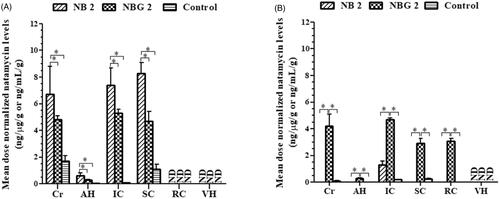
At the end of 2 h, the concentration of NT observed in the cornea and AH of rabbits administered with control at 50-fold high dose were disproportionately higher by ∼13–18 and ∼3–6-fold compared to that treated with NB 2 and NBG 2, respectively (). Hence, the mean dose normalized NT concentration in cornea and AH of rabbits treated with NB 2 and NBG 2 formulations were significantly higher, over that seen in the control group, indicating the improved transcellular penetration of NB’s across the cornea (p < .05) (). These results support the findings from corneal transport studies and could be attributed to the intercalation of bilosomes with corneal lipids and their amphiphilic nature due to the presence of bile salt (ST) driving them to hydrophilic AH [Citation23,Citation50]. In contrast, the lipophilic character might have led the drug, in the cul-de-sac from control suspension (higher amount), to partition into corneal epithelial cells resulting in high corneal drug levels, however, the poor aqueous solubility could have limited the penetration through hydrophilic stroma layer in cornea leading to low-dose normalized AH drug concentration. Unlike cornea, sclera is 10 folds more permeable to hydrophilic drugs, but it still is impervious to lipophilic molecules [Citation51,Citation52]. Thus, the actual amount of NT in rabbit sclera from control group treated with 50 folds high drug dose was disproportionately higher by ∼6- and ∼11-fold higher over that noted in NB 2 and NBG 2 group, respectively, confirming the limited scleral permeation of NT from marketed suspension (). The significantly higher mean dose normalized NT level in sclera of rabbits treated with NB 2 and NBG 2 formulations, compared to that noticed in the control group, exemplifies the ability of bilosomes as a potential vehicle to reduce the scleral barrier characteristics (p < .05) (). The amount of NT in the IC of rabbits from all the treatment groups could be attributed to the permeation of drug into the ciliary body either through cornea-scleral limbus junction [Citation15,Citation16] (). The mean dose normalized IC NT levels were significantly higher in the rabbits treated with NB 2 and NBG 2 formulations, compared to that treated with control suspension, which could be attributed to the transcytosis/endocytosis of bilosomes by cornea epithelial cells into the AH (p < .05) () [Citation23,Citation41]. As expected, the mean dose normalized drug levels in cornea, AH, IC and sclera of rabbits administered with NB 2 formulation was significantly higher compared to that treated with NBG 2 which could be due to the slow release of bilosomes from the in situ hydrogel matrix [Citation28,Citation31] (p < .05) (). The drug levels in the RC and the VH of rabbits from all the treatment groups were below the detection limit ( & ).
At the end of 6 h, no detectable drug levels were noticed in any of the ocular tissues, except sclera, in the rabbits treated with NB 2 indicating the clearance of the formulation from the precorneal space ( and ). Surprisingly, the cornea, the IC and the sclera of rabbits from the control group showed some drug levels that could be attributed to depo formation of drug in the cornea and sclera (). The undetectable drug levels in the AH, the RC and the VH in these rabbits, however, confirmed no or low drug in the cul-de-sac and poor transcorneal/transscleral penetration of NT (). These results agree with a study which reported poor NT levels (< 0.8 µg/mL) in the rabbit tear at 5-h post-topical administration of marketed suspension [Citation31]. In contrast, significantly higher mean dose normalized drug levels in all the ocular tissues, except VH, in the rabbits topically administered with NBG 2 formulation confirmed improved pre-corneal residence time of the in situ hydrogel of NT bilosomes (). As reported earlier, in situ hydrogels bond with glycoprotein moieties of the epithelial cells through hydrophobic interactions/van der Waals forces/ionic/hydrogen bonding and facilitates higher mucoadhesion with the biological membranes ensuring longer residence time [Citation53]. The NT levels noticed in the RC of the rabbits treated with NBG 2 can be attributed to the penetration of bilosomes through conjunctival-scleral pathway (). It has been reported that the topically applied colloidal nanoparticles penetrate through conjunctival-scleral pathway and reach the back of the eye tissues like the RC and/or the VH [Citation16,Citation17]. These results confirmed the ability of ion-sensitive in situ hydrogels of bilosomes as potential system for improved and prolong ocular drug delivery.
Stability studies
The physical stability of the optimized formulations at 4 °C and 25 °C are specified in . The PS, PDI, ZP and % EE of NB 2 formulations stored at 25 °C were not statistically different for 30 days but changed significantly by 60 days, with respect to those observed initially (p < .05) (). This could be due to the aggregation of bilosomes with the leakage of drug. Surprisingly, the physicochemical properties of the NBG 2 formulation did not differ upon storage at 25 °C for 60 days which could be attributed to the viscosity limited migration and aggregation of bilosomes (). No variations in the PS, PDI, ZP and % EE of NB 2 and NBG 2 formulations stored at 4 °C for 60 days suggests that both formulations can be stored at refrigerated conditions (4 °C) ().
Table 5. Physical stability of optimized natamycin bilosomes and bilosome in situ gel formulations stored at 4 °C and 25 °C for 60 days (mean ± SD; n = 3).
Conclusions
Efficient and prolonged topical drug delivery to ocular tissues is majorly hampered by poor corneal permeability and low pre-corneal residence of therapeutic molecules. In this context, NT bilosomes (NB) and corresponding in situ gel systems were successfully fabricated and evaluated. The NB formulation with equimolar ratio of vesicular components and low amount of bile salt (ST) resulted in uniformly distributed small size bilosomes with higher drug % EE. The optimized NB loaded in situ gel (NBG) system with 0.3% w/v gellan gum rapidly transformed into hydrogel in the STF, which displayed good viscoelastic and adhesive properties. The cytotoxicity and corneal histology evaluations confirmed compatibility and safety of these formulation on ocular tissues. The in vitro corneal transport studies confirmed the superior permeability characteristics of NT from the optimized formulations, over control suspension. Furthermore, considerably higher mean dose normalized drug levels in the ocular tissues from NBG for 6 h, compared to that from the control suspension, demonstrated the effectiveness of ion-sensitive in situ hydrogels of bilosomes as a potential carrier system for the improved and prolonged topical ocular drug delivery. Nevertheless, the ocular distribution studies of NT from NBs with higher drug loads is necessary to confirm the efficiency of this system as suitable vehicle enhanced ocular drug delivery platforms.
Karthik_et_al._Supplementary_Materials.zip
Download Zip (5.8 MB)Disclosure statement
The authors declare no conflicts of interest in this research.
Additional information
Funding
References
- Rowe-Rendleman CL, Durazo SA, Kompella UB, et al. Drug and gene delivery to the back of the eye: from bench to bedside. Invest Ophthalmol Vis Sci. 2014;55:2714–2730.
- Urtti A. Challenges and obstacles of ocular pharmacokinetics and drug delivery. Adv Drug Deliv Rev. 2006;58:1131–1135.
- Patel A, Cholkar K, Agrahari V, et al. Ocular drug delivery systems: an overview. World J Pharmacol. 2013;2:47–64.
- Ali M, Byrne ME. Challenges and solutions in topical ocular drug-delivery systems. Expert Rev Clin Pharmacol. 2008;1:145–161.
- Huang D, Chen YS, Rupenthal ID. Overcoming ocular drug delivery barriers through the use of physical forces. Adv Drug Deliv Rev. 2017. DOI:https://doi.org/10.1016/j.addr.2017.09.008.
- Adelli GR, Balguri SP, Bhagav P, et al. Diclofenac sodium ion exchange resin complex loaded melt cast films for sustained release ocular delivery. Drug Deliv. 2017;24:370–379.
- Balguri SP, Adelli GR, Tatke A, et al. Melt-cast noninvasive ocular inserts for posterior segment drug delivery. J Pharm Sci. 2017;106:3515–3523.
- Jain D, Kumar V, Singh S, et al. Newer trends in in situ gelling systems for controlled ocular drug delivery. Japlr. 2016;2:1–16.
- Bhardwaj A, Kumar L, Mehta S, et al. Stimuli-sensitive systems–an emerging delivery system for drugs. Artif Cells Nanomed Biotechnol. 2015;43:299–310.
- Imam SS, Bukhari SNA, Ali A. Preparation and evaluation of novel chitosan: gelrite ocular system containing besifloxacin for topical treatment of bacterial conjunctivitis: scintigraphy, ocular irritation and retention assessment. Artif Cells Nanomed Biotechnol. 2017. DOI:https://doi.org/10.1080/21691401.2017.1349779
- Sheshala R, Kok YY, Ng JM, et al. In situ gelling ophthalmic drug delivery system: an overview and its applications. Recent Pat Drug Deliv Formul. 2015;9:237–248.
- Rupenthal ID, Green CR, Alany RG. Comparison of ion-activated in situ gelling systems for ocular drug delivery. Part 1: physicochemical characterisation and in vitro release. Int J Pharm. 2011;411:69–77.
- Kushwaha SK, Saxena P, Rai A. Stimuli sensitive hydrogels for ophthalmic drug delivery: a review. Int J Pharma Investig. 2012;2:54–60.
- Mun EA, Morrison PW, Williams AC, et al. On the barrier properties of the cornea: a microscopy study of the penetration of fluorescently labeled nanoparticles, polymers, and sodium fluorescein. Mol Pharmaceutics. 2014;11:3556–3564.
- Balguri SP, Adelli GR, Janga KY, et al. Ocular disposition of ciprofloxacin from topical, PEGylated nanostructured lipid carriers: effect of molecular weight and density of poly (ethylene) glycol. Int J Pharm. 2017;529:32–43.
- Balguri SP, Adelli GR, Majumdar S. Topical ophthalmic lipid nanoparticle formulations (SLN, NLC) of indomethacin for delivery to the posterior segment ocular tissues. Eur J Pharm Biopharm. 2016;109:224–235.
- Mandal A, Bisht R, Rupenthal ID, et al. Polymeric micelles for ocular drug delivery: from structural frameworks to recent preclinical studies. J Control Release. 2017;248:96–116.
- Ikuta Y, Aoyagi S, Tanaka Y, et al. Creation of nano eye-drops and effective drug delivery to the interior of the eye. Sci Rep. 2017; 7:44229.
- Bardania H, Tarvirdipour S, Dorkoosh F. Liposome-targeted delivery for highly potent drugs. Artif Cells Nanomed Biotechnol. 2017;45:1478–1489.
- Abdelkader H, Ismail S, Hussein A, et al. Conjunctival and corneal tolerability assessment of ocular naltrexone niosomes and their ingredients on the hen's egg chorioallantoic membrane and excised bovine cornea models. Int J Pharm. 2012;432:1–10.
- Kumar D, Jain N, Gulati N, et al. Nanoparticles laden in situ gelling system for ocular drug targeting. J Adv Pharm Tech Res. 2013;4:9–17.
- Pandurangan DK, Bodagala P, Palanirajan VK, et al. Formulation and evaluation of voriconazole ophthalmic solid lipid nanoparticles in situ gel. Int J Pharma Investig. 2016;6:56–62.
- Morsi N, Ibrahim M, Refai H, et al. Nanoemulsion-based electrolyte triggered in situ gel for ocular delivery of acetazolamide. Eur J Pharm Sci. 2017;104:302–314.
- Ibrahim MM, Abd-Elgawad AH, Soliman OA, et al. Stability and ocular pharmacokinetics of celecoxib-loaded nanoparticles topical ophthalmic formulations. J Pharm Sci. 2016;105:3691–3701.
- Paradkar MU, Parmar M. Formulation development and evaluation of natamycin niosomal in-situ gel for ophthalmic drug delivery. J Drug Deliv Sci Technol. 2017;39:113–122.
- Shukla A, Mishra V, Kesharwani P. Bilosomes in the context of oral immunization: development, challenges and opportunities. Drug Discov Today. 2016;21:888–899.
- Al-mahallawi AM, Abdelbary AA, Aburahma MH, et al. Investigating the potential of employing bilosomes as a novel vesicular carrier for transdermal delivery oftenoxicam. Int J Pharm. 2015;485:329–340.
- Abdelbary AA, Abd-Elsalam WH, Al-Mahallawi AM. Fabrication of novel ultradeformable bilosomes for enhanced ocular delivery of terconazole: in vitro characterization, ex vivo permeation and in vivo safety assessment. Int J Pharm. 2016;513:688–696.
- Tanure MAG, Cohen EJ, Sudesh S, et al. Spectrum of fungal keratitis at Wills eye hospital, Philadelphia, Pennsylvania. Cornea. 2000;19:307–312.
- Phan CM, Subbaraman LN, Jones L. In vitro drug release of natamycin from β-cyclodextrin and 2-hydroxypropyl-β-cyclodextrin-functionalized contact lens materials. J Biomater Sci Polym Ed. 2014;25:1907–1919.
- Bhatta RS, Chandasana H, Chhonker YS, et al. Mucoadhesive nanoparticles for prolonged ocular delivery of natamycin: in vitro and pharmacokinetics studies. Int J Pharm. 2012;432:105–112.
- Thangabalan B, Kumar PV. Analytical method development and validation of natamycin in eye drop by RP-HPLC. Asian J Pharm Clin Res. 2013;6:134–135.
- Abdelkader H, Ismail S, Kamal A, et al. Design and evaluation of controlled-release niosomes and discomes for naltrexone hydrochloride ocular delivery. J Pharm Sci. 2011;100:1833–1846.
- Nagesh C, Patil M, Chandrashekar S, et al. A novel in situ gel for sustained ophthalmic delivery of ciprofloxacin hydrochloride and dexamethasone- design and characterization. Der Pharmacia Lett. 2012;4:821–827.
- Tai A, Bianchini R, Jachowicz J. Texture analysis of cosmetic/pharmaceutical raw materials and formulations. Int J Cosmet Sci. 2014;36:291–304.
- Ibrahim MM, Abd-Elgawad AE, Soliman OA, et al. Nanoparticle-based topical ophthalmic formulations for sustained celecoxib release. J Pharm Sci. 2013;102:1036–1053.
- Majumdar S, Srirangam R. Solubility, stability, physicochemical characteristics and in vitro ocular tissue permeability of hesperidin: a natural bioflavonoid. Pharm Res. 2009;26:1217–1225.
- Kakkar S, Kaur IP. Spanlastics-a novel nanovesicular carrier system for ocular delivery. Int J Pharm. 2011;413:202–210.
- Zhang J, Xue R, Ong WY, et al. Roles of cholesterol in vesicle fusion and motion. Biophys J. 2009;97:1371–1380.
- Janga KY, Jukanti R, Velpula A, et al. Bioavailability enhancement of zaleplon via proliposomes: role of surface charge. Eur J Pharm Biopharm. 2012;80:347–357.
- Dai Y, Zhou R, Liu L, et al. Liposomes containing bile salts as novel ocular delivery systems for tacrolimus (FK506): in vitro characterization and improved corneal permeation. Int J Nanomed. 2013;8:1921–1933.
- El Zaafarany GM, Awad GA, Holayel SM, et al. Role of edge activators and surface charge in developing ultradeformable vesicles with enhanced skin delivery. Int J Pharm. 2010;397:164–172.
- Salama HA, Mahmoud AA, Kamel AO, et al. Brain delivery of olanzapine by intranasal administration of transfersomal vesicles. J Liposome Res. 2012;22:336–345.
- Niu M, Lu Y, Hovgaard L, et al. Liposomes containing glycocholate as potential oral insulin delivery systems: preparation, in vitro characterization, and improved protection against enzymatic degradation. Int J Nanomed. 2011;6:1155–1166.
- Inactive ingredient search for approved drug products. Fedral Drug Administration IIG database-2017. Available from: https://www.accessdata.fda.gov/scripts/cder/iig/index.cfm
- Uddin MS, Al Mamun A, Kabir MT, et al. Quality control tests for ophthalmic pharmaceuticals: pharmacopoeial standards and specifications. JAMPS. 2017;14:1–17.
- Khouryieh HA, Herald TJ, Aramouni F, et al. Intrinsic viscosity and viscoelastic properties of xanthan/guar mixtures in dilute solutions: effect of salt concentration on the polymer interactions. Food Res Int. 2007;40:883–893.
- Dintzis F, Babcock G, Tobin R. Studies on dilute solutions and dispersion of the polysaccharide from xanthomonas campestris nrrl b-1459. Carbohydr Res. 1970;13:257–267.
- Zignani M, Tabatabay C, Gurny R. Topical semi-solid drug delivery: kinetics and tolerance of ophthalmic hydrogels. Adv Drug Deliv Rev. 1995;16:51–60.
- Shamma RN, Elsayed I. Transfersomal lyophilized gel of buspirone HCl: formulation, evaluation and statistical optimization. J Liposome Res. 2013;23:244–254.
- Al-Halafi AM. Nanocarriers of nanotechnology in retinal diseases. Saudi J Ophthalmol. 2014;28:304–309.
- Barar J, Asadi M, Mortazavi-Tabatabaei SA, et al. Ocular drug delivery; impact of in vitro cell culture models. J Ophthalmic Vis Res. 2009;4:238–252.
- Yu W, do Egito EST, Barratt G, et al. A novel approach to the preparation of injectable emulsions by a spontaneous emulsification process. Int J Pharm. 1993;89:139–146.