Abstract
Pancreatic tissue engineering as a therapeutic option for restoring and maintenance of damaged pancreas function has a special focus to using synthetic Scaffolds. This study was designed to evaluate pancreatic differentiation of human induced pluripotent stem cells (hiPSCs) on poly-L-lactic acid and polyvinyl alcohol (PLLA/PVA) scaffolds as 3 D matrix. During differentiation process, morphology of cells gradually changed and iPSCs derived insulin producing cells (iPSCs-IPCs) formed spherical shaped cell aggregation that was the typical shape of islets of pancreas. The highly efficient differentiation of iPSCs into a relatively homogeneous population of IPCs was shown by immunostaining. Real-time reverse transcription polymerase chain reaction (RT-PCR) results demonstrated that iPSCs-IPCs expressed pancreas-specific transcription factors (Pdx1, insulin, glucagon and Ngn3). The expressions of these transcription factors in PLLA/PVA scaffold were significantly higher than 2 D groups. Furthermore, we showed that concentration of insulin and C-peptide in PLLA/PVA scaffold and/or 2 D culture in response to various concentrations of glucose increased but the difference between them were not significant. Altogether the current results demonstrated that PLLA/PVA scaffold could provide the microenvironment that promotes the pancreatic differentiation of iPSCs, up-regulate pancreatic-specific transcription factors and improved metabolic activity.
Introduction
Diabetes mellitus is a group of metabolic disorders in which the blood sugar levels are higher than normal levels. According to statistics provided by the American Diabetes Association diabetes is seventh leading cause of death in US And unfortunately its prevalence is increasing [Citation1,Citation2]. Diabetes is one of the main reasons of hypoglycemia, hypertension, dyslipidemia, kidney failure, blindness and eye problem and in advanced cases it leads to amputation [Citation3]. For these reasons, as well as the close relationship between diabetes and cardiovascular disease and stroke, the American Diabetes Association has introduced new guidelines for dealing with diabetes [Citation2]. Type I diabetes mellitus (T1DM) consists of 5–10% of diabetes that results from autoimmune destruction of pancreatic beta-cells and pancreas’s failure to produce enough insulin. Currently, daily injection of insulin is the best treatment option for patients with this type of diabetes, it is not only painful for patients but also imposes a high cost on the individual and the society. Although the cause of the disease is not well known, it seems that different genetic and environmental factors are involved in the onset of the disease. Over the past two decades, many researchers have tried to use the pancreatic islet transplantation as an alternative treatment for insulin injection; but this option is limited due to shortage of islets cells donor [Citation4].
Recently, many studies have sought to use stem cells to produce pancreatic beta cells and focus on the production and expansion of these alternative sources [Citation5–8]. Meanwhile, induced pluripotent stem cells (iPSCs), due to their high potential of self-renewability and differentiation as well as low immunogenicity, are an appropriate cell source for use in alternative therapies [Citation9–11]. iPSCs can be cultured in vitro and by using growth f and transcription factors that recapitulate development of pancreatic cells, it can be differentiated to insulin-producing beta cells (IPCs). In recent years, numerous studies have tried to use cell-based therapy for T1DM [Citation12,Citation13]. To obtain the cells needed for cell therapy; these studies have used various methods, such as gene transfer and the use of differentiation medium for the differentiation of stem cells into beta cells [Citation5,Citation11]. These strategies are expensive and time consuming. In order to circumvent these problems, new approach must be developed. Several studies have shown that the use of 3 D environments can be a way to overcome the limitations of current beta cell production protocols [Citation14–16]. Recent advances in tissue engineering have enabled the regulation of cells through the scaffolds made of biocompatible and biodegradable polymers. In the field of biomaterials, these materials quickly adapt to the human body and do not stimulate immune response. These scaffolds provide a 3 D environment that enhances the differentiation of stem cell types into pancreatic beta cells. It seems that these three-dimensional scaffolds, by mimicking in vivo environment, support the cell-extracellular matrix (ECM) interactions as well as the cell-cell contacts [Citation14,Citation17–19]. In the present study, we intend to use a flexible, hydrophilic, biodegradable and biocompatible polymer as a suitable substrate for differentiating induced pluripotent stem cells into pancreatic beta cells. Poly-L-lactic acid and polyvinyl alcohol (PLLA/PVA)-based scaffold may display biological properties and provide suitable synthetic ECM for the promotion beta cells differentiation and islet organization [Citation20–22]. Therefore, the current study aims to evaluate the effect of the PLLA/PVA scaffold on iPSCs differentiation into IPCs.
Materials and methods
Expansion of induced pluripotent stem cells
Human iPSC lines were provided by Prof. Masoud Soleimani of Stem Cells Technology Research Center that previously characterized and reported this cell line [Citation11]. These cells were cultured and expanded on a mouse embryonic fibroblast (MEF) feeder layer inactivated with mitomycin-C (Sigma,St. Louis, MO) in iPSCs medium (Dulbecco's Modified Eagle Medium: Nutrient Mixture F-12 (DMEM/F12) supplemented with 20% knockout serum replacement, 0.1 mmol/l nonessential amino acids, 2 mM L-glutamine, 0.1 mM β-mercaptoethanol and 10 ng/ml of recombinant human basic fibroblast growth factor (bFGF), purchased from Invitrogen, Carlsbad, CA) in a 5% CO2 and 95% humidity. Every 4–6 days, human iPSC colonies were passaged enzymatically using 0.1% collagenase type IV (Invitrogen) and were transferred onto inactivated MEF for expansion.
Scaffold fabrication
Amount 0.8 g of PLLA polymer was dissolved in 9 ml of chloroform and 1 ml of dimethylformamide and 1.2 g of PVA polymer were dissolved in 10 ml of hot water. Then, these solutions were loaded into two 10 ml plastic syringes and were placed across the collector at fixed distance of 18 cm. Electrospinning was done at flow rate of 1 ml/h, under a positive voltage of 23 KV between needle and collector.
Surface modification
After scaffold fabrication, to optimize the hydrophilicity, surface plasma treatment of PLLA/PVA scaffolds was performed under optimized conditions of 40 kHz low frequency at 30 w with a cylindrical quartz reactor (Diener Electronics, Germany) by introducing pure oxygen into the reaction chamber at 0.4 mbar pressure and later the glow discharge was applied for 5 min.
Scanning electron microscopy
Cellular and cell-free PLLA/PVA scaffolds were fixed in 2.5% glutaraldehyde (45 min). The fixed samples were rinsed two times with phosphate buffered saline (PBS) for 5 min; after were then dehydrated in ethanol series (50, 60, 70, 80, 95 and 100% v/v) for 10 min each and dried. The dried scaffolds were mounted onto aluminum sample holders with Araldite TM glue and were also
coated with palladium gold in a sputter-coater (Hitachi, Tokyo, Japan). Finally, the samples were observed using a scanning electron microscope (SEM; Hitachi S-4500, Japan) at an accelerating voltage of 5 kV.
Cell seeding on scaffolds
In order to sterilization, the nanofibrous scaffolds were punched to the desired size and sterilized under ultraviolet light for 30 min. Later the scaffolds were rinsed with PBS and were put overnight in the DMEM medium supplemented with 10% serum at 37 °C. For embryoid body(EB) differentiation, iPSCs colonies were detached enzymatically using 0.1% collagenase type IV (Invitrogen) and were transferred to ultra-low adherent culture plate in EB medium (iPSC medium without bFGF) for five days. Formed EBs plated were coated on PLLA/PVA scaffolds at a density of 5 × 104 cells per scaffold in MEF-conditioned medium for two days.
Experimental design
Three groups including two experimental and two control groups were considered. The hiPSCs were cultured in tissue culture plate (TCP) under iPSCs medium as the 2 D control group. The seeded hiPSCs onto the PLLA/PVA scaffolds were cultured in iPSCs media and were used as the 3 D control group. The cultured hiPSCs in IPC differentiation media were used as the 2 D experimental group. The seeded hiPSCs onto the PLLA/PVA scaffolds were cultured in IPC differentiation media and were applied as the 3 D experimental group. A three-step differentiation protocol was used to induce hiPSCs into IPC. At Step 1: the hiPSCs were cultured (37 °C, 5% CO2) in the medium I containing serum-free high glucose DMEM (25 mmol/L) containing 0.5 mmol/L beta-mercaptoethanol (Invitrogen) and 50 ng/ml activin A (Sigma) for seven days. Then, in step 2: the hiPSCs were treated by the differentiation medium II containing 1% nonessential amino acids (Invitrogen), 20 ng/ml epidermal growth factor (EGF; Sigma), 20 ng/ml basic fibroblast growth factor (bFGF; Sigma), 2 mmol/L Lglutamine (Sigma), 2% B27 (Invitrogen) and 10 ng/ml exendine-4 (Sigma) for seven days. Finally, in Step 3: the hiPSCs were cultured for an additional seven days in differentiation medium III containing 10 ng/ml betacellulin (Sigma), 2% B27, 10 mmol/L nicotinamide (Sigma) and 10 ng/ml exendine-4. During differentiation process, the differential media was replaced with fresh medium every two days.
Cell proliferation assay
To evaluate the proliferation ability and viability of iPSCs on PLLA/PVA nanofibrous scaffold 3-(4,5-dimethylthiazol-2-yl)-2,5-diphenyltetrazolium bromide (MTT) assay was used for four days during the culturing procedure. Briefly, sterilized nanofibers were placed in a 48-well cell culture plate, seeded at a cell density of 4 × 103 cells/cm2 and were incubated at 37 °C, 5% CO2. At 24, 48 and 72 h after seeding hiPSCs, 50 µL MTT solution with a final concentration of 5 mg/ml in DMEM without fetal bovine serum (FBS) was added to each well. After 3 h incubation, the supernatant was discarded and the formazan crystals were solubilized with dimethylsulfoxide (DMSO). Plates were shaken for 10 min to dissolve the precipitate. The sample absorbance was quantified by absorbance measurement at 570 nm using a BioTek Synergy HT micro-plate reader (BioTek Instruments Inc., Winooski, VT). The results were obtained as mean values of three repeats in 2 D and 3 D culture groups.
RNA extraction and quantitative real-time polymerase chain reaction (PCR)
The hiPSC’s were seeded in a six-well plate at 2.0 × 105 cells/well and were grown until the cell density reached 80%. Total RNA was isolated from differentiated hiPSCs and control cells at the end of the induction process with the RNeasy Mini Kit (Qiagen, Germany) following the manufacturer’s protocol. Later, 1 μg of total RNA was reverse transcribed by the random hexamer primer to complementary DNA (cDNA) using a M-MuLV Reverse Transcriptase kit (Fermentas, Ontario, Canada) at 42 °C for 60 min and 70 °C for 5 min in a total reaction volume of 15 μl. Quantitative real-time PCR was performed using a SYBR premix ExTaq (Takara, Korea) according to the manufacturer’s protocol and with the ABI light cycler (ABI step one) in a total reaction volume of 10 μl in 96-well optical reaction plates. The primer sequences were designed using AlleleID software (Primer Biosoft, Palo Alto, CA), which is illustrated in . All reactions were performed at 95 °C for 2 min, followed by 40 amplification cycles in triplicate of 30 s at 94 °C for denaturation, 30 s at 60 °C for annealing and final extension at 72 °C for 30 s. The data were normalized against the Ct value of the housekeeping gene β2M. The relative quantification (ΔΔCt) method was applied to analyze the data.
Table 1. Specifications of primer sequences used for quantitative real-time PCR.
Immunofluorescence assay
To evaluate pancreatic differentiation, the differentiated cells in both 2 D and 3 D groups were fixed with 4% (w/v) paraformaldehyde (Sigma) in PBS at 4 °C for 30 min in different stages of differentiation process. All samples were permeabilized with 0.2% triton X-100 (Sigma) for 10 min and blocked with 10% goat serum (Sigma) for 1 h at room temperature. For definitive endoderm stage, primary antibody against human PDX1 (1:2000; #ab47267) and for maturation stage primary antibody against insulin (1:200; #ab181547) were diluted with 1% bovine serum albumin (BSA) in PBS. All samples were incubated overnight with these primary antibodies at 4 °C. Later these samples were rinsed twice with PBS and were subsequently incubated with fluorescein isothiocyanate-labeled secondary antibodies for 90 min at room temperature in darkness. After washing with PBS, the nuclei were stained with 0.1 μg/ml DAPI (Sigma) for 5 min. Images were captured under a phase contrast fluorescent microscope (Nikon, Tokyo, Japan).
Insulin and C-peptide release as well as insulin content assay
The differentiated hiPSCs in both 2 D and 3 D groups were washed twice with PBS and were pre-incubated in freshly prepared Krebs-Ringer bicarbonate (KRB) buffer containing 25 mM NaHCO3, 5 mM KCl, 2.5 mM CaCl2, 1.1 mM MgCl2, 120 mM NaCl and 0.1% BSA (all from Sigma) in glucose-free for 2 h. Then, differentiated cells were incubated for two hours in KRB buffer with different concentrations of glucose. The supernatant were collected at stored at −70 °C until analyzation. To evaluate insulin and C-peptide secretion, the ultrasensitive ELISA kit (#10–1132-01, #10–1141- 01, Mecodia, Uppsala, Sweden) was used according to the manufacturer’s protocols. Total protein concentration was determined by the bicinchoninic acid (BCA) protein assay system and 50 μg of protein was used for detection of intracellular insulin in each well of ELISA kit.
Statistical analysis
All experiments were performed at least in triplicate independently and the obtained data were reported as a mean ± standard deviation (SD). Results were analyzed by one-way analysis of variance (ANOVA) and Bonferroni’s post-hoc test was used for comparison of 3 D and 2 D groups by Graphpad Prism 6 software (Graphpad Software Inc., La Jolla, CA). A value of p < .05 was considered statistically significant.
Results
Morphological changes of hiPSCs differentiation into IPCs
To generate IPCs from hiPSCs, we used a previously reported stepwise differentiation protocol that followed human pancreatic beta cells developmental principles with some modifications, such as obtaining IPCs in a short time of differentiation. Under the inverted and scanning electron microscope, the induction of hiPSCs differentiation into IPCs and cell morphologies were recorded at end of differentiation stages ( and ). SEM images established that the nanofibrous scaffold was very porous and contained numerous interconnected pores (). After the cells were cultured in plates and on the nanofibrous scaffolds and following sequential addition of differentiation medium I, II and III for 21 days, hiPSCs converted to sheets of cells and occupied the pores of the PLLA/PVA scaffolds in both experimental and control 3 D culture. In the control group, hiPSCs took an elongated and flat shape (); while the differentiated cells in the experimental group were cluster- shaped in structure which is typical morphological feature of pancreatic islet (). Finally, these cluster-shaped structures were used for further evaluation to test the efficiency of differentiation protocol in different groups.
Figure 1. Morphological changes in 2 D culture. (a) hiPSCs in DMEM (control group) and (b) hiPSCs in IPC differentiation media. Scale bars are 100 µm.
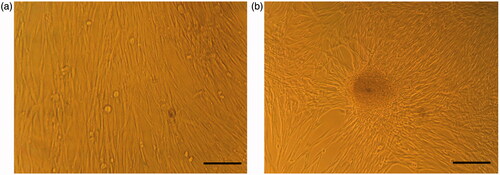
Figure 2. Morphological changes in 3 D culture. (a) Unseeded scaffold. Interconnected pores are observed. (b) hiPSCs-seeded scaffold in DMEM (control group). The cells show spindle-shape morphology. (c) hiPSCs-seeded scaffold in IPC differentiation media. The cells form spherical clusters of round cells. Scale bars are 10 and 30 µm, respectively.
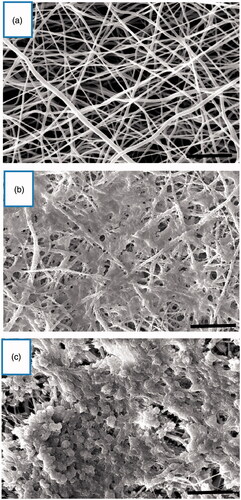
Cell viability and cell proliferation assessment
MTT tests indicated biocompatibility of PLLA/PVA scaffold and its effectiveness in supporting cell proliferation. As shown in , the viability and proliferation rate of the hiPSCs on scaffold were significantly higher than the 2 D culture group. Cell viability on PLLA/PVA nanofibrous scaffolds indicated significantly increased viability of cells when compared with the sample of 2 D culture on the fifth and seventh days; however, on the first and third days of the culture, the proliferation of scaffold did not increase in comparison with 2 D culture group.
Gene expression pattern of pancreatic endocrine genes in derived IPCs
To indicate whether the hiPSCs had derived into IPCs, real time reverse transcription polymerase chain reaction (RT-PCR) analysis was performed to evaluate endocrine genes expression profiles for pancreatic cell differentiation markers. Since genes expression pattern analysis displayed no statistically significant differences between the two controls, both of them were considered as a single group. As shown in , the expression of Pdx1, insulin, glucagon, and Glut2 genes in undifferentiated hiPSCs, as a control group, was low. After the hiPSCs differentiated into IPCs, real time RT-PCR analysis indicated that the expression pattern of these genes was significantly increased in the 2 D culture of hiPSCs-derived IPCs in comparison with undifferentiated hiPSCs. High expression of the mentioned genes was observed in the cells cultured and differentiated on the PLLA/PVA scaffold. HiPSCs-derived IPCs showed a significant increase in Pdx1, Ngn3, glucagon and insulin gene expression when they were cultured in PLLA/PVA scaffolds in comparison to the conventional monolayer culture. Overall, these data showed that the presence of a 3 D scaffold along with the induction media could lead to effective differentiation of hiPSCs to IPCs and PLLA/PVA nanofibrous scaffolds as a synthetic polymer can improve IPCs differentiation.
Figure 4. Relative gene expression in end stage derived IPCs. The expression levels of pancreatic transcription factors such as Pdx1, Ngn3, insulin, glucagon and Glut2 were analyzed in each group of differentiation into IPCs. Gene transcripts of 3 D group are compared with the 2 D group. Relative levels of gene expression were normalized to the human β2M. The values in each graph are represented as mean ± SD. *p < .05, **p < .01 and ****p < .0001.
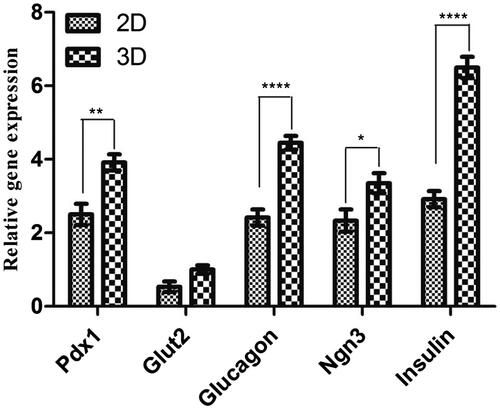
Immunofluorescence staining
To confirm pancreatic differentiation of hiPSCs into IPCs, expression pancreatic-specific markers (insulin and Pdx1) was examined using the immunostaining assay (). Immunocytochemistry did not show any Pdx1 and insulin genes expression in 2 D and 3 D control groups (data not shown). In the 2 D experimental culture group, weak or moderate expression of Pdx1 and insulin was observed in the nuclei and cytoplasm, respectively, in differentiated IPCs on day 21. In 3 D experimental culture group, strong immune-reactivity of both markers was observed in the nuclei and cytoplasm of differentiated IPCs on day 21. Qunter-staining of the nucleus (blue) was performed by 4',6-diamidino-2-phenylindole (DAPI). These results are presented in .
Figure 5. Immunocytochemical analysis in end stage derived IPCs. Immunofluorescence analysis detected nuclei localization of PDX1 and cytoplasmic localization of insulin in differentiated IPCs at day 14 in the 3D group (a) and 2 D group (b). Counter-staining of nucleus was performed by DAPI. Images were obtained by a fluorescence microscope. Scale bars are 100 µm.
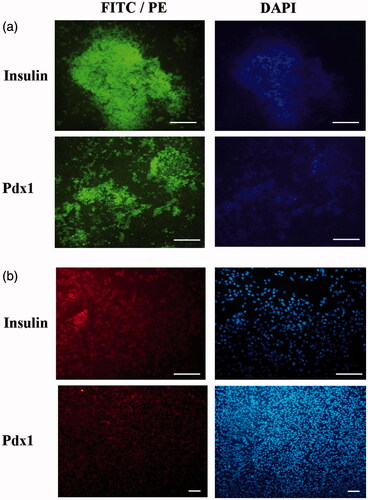
Insulin and C-peptide release in response to glucose stimulation
In order to evaluate the pancreatic-specific functions of differentiated cells, C-peptide and insulin secretion of hiPC-IPCs in response to various concentrations of glucose were performed by utilizing an ELISA kit. The cultured hiPSCs in control groups indicated very low levels of insulin and C-peptide in the presence low or high of glucose concentrations. Both derived IPCs mediated through the protocol with 2 D and 3 D did not have a significant response to concentrations of glucose stimulation from 5.5–15 mM compared to each other (p > .05). However, in differentiated IPCs with 3 D and 2 D, insulin secretions were increased by a concentration of 25 mM glucose which was secreted at 9.76 ± 0.75 and 7.9 ± 0.4 ng/106 cells (n = 6) per 60 min and the amounts of C-peptide were 37.5 ± 2.45 and 28.5 ± 3.51 pmol/l (n = 6), respectively. Thus, our results in showed that differentiated IPCs with 3 D in concentration of 25 mM glucose stimulation could significantly produce more C-peptide and insulin compared to differentiate IPCs with 2 D (p < .05). Moreover, the insulin content in the differentiated IPCs was measured. The values for insulin in 5.5, 15 and 25 mM glucose concentrations in 2 D group were 6.25 ± 3.25, 6 ± 2.32 and 15.88 ± 3.6 ng/mg, respectively and the values in 5.5, 15 and 25 Mm glucose for 3 D group were 7.2 ± 1.2, 10.75 ± 3.4, 22.7 ± 5.2 ng/mg, respectively. Thus, the obtained results showed that maturated IPCs in 3 D scaffold group could produce significant amount of insulin as compared to 2 D group in the 15 and 25 mM. These results are shown in .
Figure 6. In vitro insulin and C-peptide response assay in end stage derived IPCs. (a and b) insulin secretion and C-peptide release changes in two groups of IPCs and control group in response to various concentrations of glucose from 5.5 to 25 mM. (c) Intracellular insulin content in each concentration of glucose that was normalized with total cellular protein. Values are expressed as mean ± SD (n = 6). *p < .05, **p < .01 and ***p < .001.
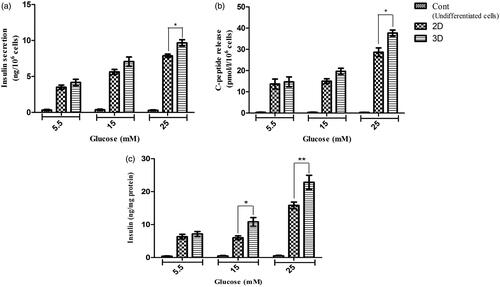
Discussion
In recent years, with increasing knowledge about mechanisms of pancreas development and the emergence of iPSCs technology, researchers were encouraged to use these cells for the production of beta cells. Due to the unlimited self-renewing capacity and less immunogenicity of iPSCs, they have become the preferred choice to generate various tissues such as bone [Citation23], cartilage [Citation24], neural [Citation25], liver [Citation19], etc. In this regard, scientists around the world are looking for ways to use iPSCs to produce IPCs and treat T1DM. Since iPSCs can be generated from the reprogramming of the patient's somatic cells, they can be an appropriate option for cell therapy in a diabetic's autologous transplantation. Therefore, several studies have reported that iPSCs can effectively differentiate into IPCs [Citation10,Citation11]. Meanwhile, the greatest success has been with protocols that have used 3 D environments with a step-wise differentiation medium [Citation14,Citation17,Citation26]. Compared to 2 D culture strategies, 3D environments can optimize the differentiation of iPSCs towards higher functional IPCs. In 3 D culture, cell aggregation enhance islet like morphology, differentiation and functionality of iPSCs-IPCs.
In this study, we used PLLA/PVA scaffold as substrate for differentiation of iPSCs into IPCs. To investigate the effect of this scaffold on iPSCs during pancreatic differentiation, we seeded the iPSCs in PLLA/PVA scaffold and tissue culture plates (TCPs) with same pancreatic induction medium and further assessed cell morphology and function. The invert and electron microscope images showed, in both groups, during differentiation process, morphology of cells gradually changed and finally hiPSC-IPCs converted spherical-shaped cell that was the typical shape of islets of Langerhans. The milestone of our study was 3 D morphology of iPSCs-IPCs in PLLA/PVA scaffold at day 21 that was completely similar to spherical shaped islets of Langerhans. This morphology was not seen in many same studies conducted during the same periods [Citation14,Citation27]. Our immunofluorescence analysis revealed the expression of insulin and Pdx1 in iPSCs-IPCs cultured on PLLA/PVA scaffold qualitatively were higher than 2 D culture. These results suggest that this scaffold could support homogenous and efficient differentiation iPSCs into IPCs. The induction of these expressions of pancreatic markers during differentiating iPSCs on PLLA/PVA scaffold, were also confirmed by our real time PCR analysis. When we focused on the expression of pancreas-specific transcription factors, we observed the difference between 2 D and 3 D groups was statistically significant in pancreatic specific markers in mRNA and protein level. The expression of Pdx1, insulin, glucagon, and Ngn3 genes in PLLA/PVA scaffold were significantly higher than 2 D cultures. Glut-2 gene as a glucose transporter and beta cell maturity marker, although was expressed in both groups, had no statistically significant difference between them. The results of response to the different concentration of glucose did not show any significant difference in term of insulin and C-peptide releasing which can be due to the lack of significant expression of Glut-2 gene between the two groups.
The exact cellular and molecular events that PLLA/PVA scaffold exerts on iPSCs during differentiation to IPCs were not investigated in this study. Previous studies have shown that 3 D cultures increase global gene expression, cell differentiation and cell proliferation. It has been stated that in 3 D culture, these cellular behavior may be related to physiological interactions of cultured cells with neighboring cells and extracellular matrix [Citation28–32]. Besides the increase in the expression of genes in mRNA and protein level which are determinant in differentiation of IPCs and production of insulin in 3 D culture (as shown here), Baharvand et al. have shown that the involvement of cellular organelles in protein synthesis also increase [Citation33]. In addition, 3 D culture systems are likely to improved interactions between beta cells with other cells in the islet and could enhance insulin secretory dynamics. Altogether, our results demonstrated that iPSCs cultured on PLLA/PVA scaffolds differentiate into IPCs with proper morphological, molecular and functional characteristics, suggesting PLLA/PVA as an ideal scaffold to provide a proper microenvironment promoting pancreatic differentiation of iPSCs.
Conclusion
In conclusion, we introduced a new approach for the differentiation of hiPSCs to insulin-producing cells by enhancing a differentiation protocol with PLLA/PVA scaffold for the first time. The results of our study show that these IPCs have a beta cell pancreatic characteristic in the mRNA and protein levels and also are seen to respond to glucose stimulation test. However, more research is required to investigate the function of these IPCs in vivo.
Acknowledgements
Authors wish to thank Stem Cell Technology Research Center for the use of their laboratory facilities.
Disclosure statement
No potential conflict of interest was reported by the authors.
References
- Rathmann W, Giani G. Global prevalence of diabetes: estimates for the year 2000 and projections for 2030. Diabetes Care. 2004;27:2568–2569.
- Association AD. 2. Classification and diagnosis of diabetes. Diabetes Care. 2016;39(Supplement 1):S13–S22.
- Chapman J. Chronic calcineurin inhibitor nephrotoxicity—lest we forget. Am J Transplant. 2011;11:693–697.
- Ellis C, Ramzy A, Kieffer TJ. Regenerative medicine and cell-based approaches to restore pancreatic function. Nat Rev Gastroenterol Hepatol. 2017;14:612–628.
- Piran M, Enderami SE, Piran M, et al. Insulin producing cells generation by overexpression of miR-375 in adipose-derived mesenchymal stem cells from diabetic patients. Biologicals. 2017;46:23–28.
- Rajaei B, Shamsara M, Taha MF, et al. Pancreatic endoderm‐derived from diabetic patient‐specific induced pluripotent stem cell generates glucose‐responsive insulin‐secreting cells. J Cell Physiol. 2017;232:2616–2625.
- Aghazadeh Y, Nostro MC. Cell therapy for type 1 diabetes: current and future strategies. Curr Diab Rep. 2017;17:37.
- Bai C, Gao Y, Li Q, et al. Differentiation of chicken umbilical cord mesenchymal stem cells into beta-like pancreatic islet cells. Artif Cells Nanomed Biotechnol. 2015;43:106–111.
- Zhang D, Jiang W, Liu M, et al. Highly efficient differentiation of human ES cells and iPS cells into mature pancreatic insulin-producing cells. Cell Res. 2009;19:429–438.
- Nostro MC, Keller G. Generation of beta cells from human pluripotent stem cells: potential for regenerative medicine. Semin Cell Dev Biol. 2012;23:701–710.
- Enderami SE, Mortazavi Y, Soleimani M, et al. Generation of insulin‐producing cells from human‐induced pluripotent stem cells using a stepwise differentiation protocol optimized with platelet‐rich plasma. J Cell Physiol Suppl. 2017;232:2878–2886.
- Li Y, Zhang R, Qiao H, et al. Generation of insulin-producing cells from PDX-1 gene-modified human mesenchymal stem cells. J Cell Physiol. 2007;211:36–44.
- Okere B, Lucaccioni L, Dominici M, et al. Cell therapies for pancreatic beta-cell replenishment. Ital J Pediatr. 2016;42:62.
- Aloysious N, Nair PD. Enhanced survival and function of islet-like clusters differentiated from adipose stem cells on a three-dimensional natural polymeric scaffold: an in vitro study. Tissue Eng Part A. 2014;20:1508–1522.
- Amer LD, Mahoney MJ, Bryant SJ. Tissue engineering approaches to cell-based type 1 diabetes therapy. Tissue Eng Part B Rev. 2014;20:455–467.
- Enderami SE, Soleimani M, Mortazavi Y, et al. Generation of insulin‐producing cells from human adipose‐derived mesenchymal stem cells on PVA scaffold by optimized differentiation protocol. J Cell Physiol. 2018;233:4327–4337.
- Hoveizi E, Nabiuni M, Parivar K, et al. Definitive endoderm differentiation of human-induced pluripotent stem cells using signaling molecules and IDE1 in three-dimensional polymer scaffold. J Biomed Mater Res A. 2014;102:4027–4036.
- Ravi M, Paramesh V, Kaviya S, et al. 3D cell culture systems: advantages and applications. J Cell Physiol. 2015;230:16–26.
- Mahmoodinia Maymand M, Soleimanpour-lichaei HR, Ardeshirylajimi A, et al. Improvement of hepatogenic differentiation of iPS cells on an aligned polyethersulfone compared to random nanofibers. Artif Cells Nanomed Biotechnol. 2018 [cited 2017 Jul 11]; [8 p.]. DOI:https://doi.org/10.1080/21691401.2017.1345929
- Fazili A, Gholami S, Zangi BM, et al. In vivo differentiation of mesenchymal stem cells into insulin producing cells on electrospun Poly-L-Lactide acid scaffolds coated with matricaria chamomilla L. Oil. Cell J. 2016;18:310.
- Kanimozhi K, Basha SK, Kumari VS. Processing and characterization of chitosan/PVA and methylcellulose porous scaffolds for tissue engineering. Mater Sci Eng C. 2016;61:484–491.
- Liu Y, Wu N, Wei Q, et al. Wetting behavior of electrospun poly (L‐lactic acid)/poly (vinyl alcohol) composite nonwovens. J Appl Polym Sci. 2008;110:3172–3177.
- Ardeshirylajimi A. Applied induced pluripotent stem cells in combination with biomaterials in bone tissue engineering. J Cell Biochem. 2017;118:3034–3042.
- Mahboudi H, Soleimani M, Enderami S, et al. The effect of nanofibre-based polyethersulfone (PES) scaffold on the chondrogenesis of human induced pluripotent stem cells. Artif Cells Nanomed Biotechnol. 2018 [cited 2017 Nov 6]; [9 p.]. DOI:https://doi.org/10.1080/21691401.2017.1396998
- Lee HK, Velazquez Sanchez C, Chen M, et al. Three dimensional human neuro-spheroid model of Alzheimer's disease based on differentiated induced pluripotent stem cells. PLoS One. 2016;11:e0163072.
- Khorsandi L, Nejad-Dehbashi F, Ahangarpour A, et al. Three-dimensional differentiation of bone marrow-derived mesenchymal stem cells into insulin-producing cells. Tissue Cell. 2015;47:66–72.
- Khorsandi L, Khodadadi A, Nejad-Dehbashi F, et al. Three-dimensional differentiation of adipose-derived mesenchymal stem cells into insulin-producing cells. Cell Tissue Res. 2015;361:745–753.
- Birgersdotter A, Sandberg R, Ernberg I. Gene expression perturbation in vitro—a growing case for three-dimensional (3D) culture systems. Sem Cancer Biol. 2005;15:405–412.
- Islami M, Mortazavi Y, Soleimani M, et al. In vitro expansion of CD 133+ cells derived from umbilical cord blood in poly-l-lactic acid (PLLA) scaffold coated with fibronectin and collagen. Artif Cells Nanomed Biotechnol. 2018 [cited 2017 Aug 6]; [9 p.]. DOI:https://doi.org/10.1080/21691401.2017.1358733
- Birhanu G, Akbari Javar H, Seyedjafari E, et al. An improved surface for enhanced stem cell proliferation and osteogenic differentiation using electrospun composite PLLA/P123 scaffold. Artif Cells Nanomed Biotechnolnb. 2018 [cited 2017 Aug 24]; [8 p.]. DOI:https://doi.org/10.1080/21691401.2017.1367928
- Mousavi SH, Abroun S, Soleimani M, et al. 3-Dimensional nano-fibre scaffold for ex vivo expansion of cord blood haematopoietic stem cells. Artif Cells Nanomed Biotechnol. 2018 [cited 2017 Jul 7]; [9 p.]. DOI:https://doi.org/10.1080/21691401.2017.1337026
- Nadri S, Barati G, Mostafavi H, et al. Differentiation of conjunctiva mesenchymal stem cells into secreting islet beta cells on plasma treated electrospun nanofibrous scaffold. Artif Cells Nanomed Biotechnol. 2018 [cited 2017 Dec 14]; [10 p.]. DOI:https://doi.org/10.1080/21691401.2017.1416391
- Baharvand H, Hashemi SM, Ashtiani SK, et al. Differentiation of human embryonic stem cells into hepatocytes in 2D and 3D culture systems in vitro. Int J Dev Biol. 2004;50:645–652.