Abstract
Zinc oxide (ZnO) nanoparticles can exhibit toxic effect on cells and tissues, which may be involved in the excessive generation of reactive oxygen species (ROS) and the consequent mitochondria-mediated apoptotic pathway. Nevertheless, the detailed mechanism remains unclear. In this study, we explored the effects of ZnO nanoparticles on the expressions of cytochrome c, ATP level, mitochondrial membrane potential, ROS, apoptosis, total antioxidant enzyme activities and apoptotic-related protein levels in murine photoreceptor cells as well as the changes of proteomic profiling. Moreover, we also performed the bioinformatics analysis for the differentially expressed proteins. Our results show that ZnO nanoparticles induce the release of cytochorme c, decrease the intracellular ATP level, collapse the mitochondrial membrane potential, elevate the ROS level, inhibit total antioxidant enzyme activities and increase the Bax and Caspase 3 levels whereas it decrease the Bcl-2 expression, leading to cell death. Proteomic analysis reveals the differentially expressed proteins are involved in cytochrome c oxidase activity and oxidative phosphorylation. Protein-protein interaction analysis confirms the differentially expressed proteins are closely associated with the clusters related to apoptotic signaling pathway and oxidative phosphorylation-associated proteins. Our results indicate that mitochondria play a central role in ZnO nanoparticle-induced murine photoreceptor cell death.
Introduction
Nanomaterials demonstrate novel physicochemical properties which depend on their interaction with biological substrates and processes and could thus show great potential in cell imaging [Citation1], biosensor [Citation2], gene therapy [Citation3] and drug delivery [Citation4] because of their nanoscale effects. Zinc oxide (ZnO) nanoparticles are attractive materials because they are regarded as a bio-safe material that possesses photo-oxidizing and photocatalysis influences on chemical and biological species [Citation5]. Currently, ZnO nanoparticles have been applied in diet additive [Citation6], cosmetic [Citation7], antidiabetes [Citation8] and anticancer fields [Citation9,Citation10]. However, investigations of cytotoxic effects demonstrates that ZnO nanoparticles also damage normal cells including human lens epithelial cells [Citation11], lung epithelial cells [Citation12], retinal ganglion cells [Citation13] and murine photoreceptor cells [Citation14]. The mechanisms of these damages are associated with calcium ion (Ca2+), autophagy, apoptosis, transforming growth factor-β (TGF-β) and matrix metalloproteinases (MMP) signaling pathways. Nevertheless, the underlying mechanism is not well understood.
Mitochondria are small ubiquitous organelles of eukaryotic cells that are usually responsible for alterations in substrate availability and energy utilization to maintain cellular ATP supplies. At the same time, the mitochondrial respiratory chain is also a major source for the generation of reactive oxygen species (ROS) [Citation15]. As a result, mitochondrial dysfunction is detrimental to target cells because the decreased ATP level and excessive ROS generation will further exacerbate oxidative stress [Citation16]. The increased oxidative stress can reduce mitochondrial membrane potential (ΔΨm), induce chromosome c release from mitochondria to cytoplasm [Citation17,Citation18], initiating mitochondria-dependent apoptotic pathway and finally leading to cell death.
The advent of high-throughput proteome analysis has enabled the identification and quantitative determination of protein amounts in complex extracts. Thus, proteomic analysis provides us a new insight into bioinformatics from biological processes to the specific signaling pathways. It will support definite and effective access to better understand the physiological and pathological mechanism of living organisms [Citation19]. It is reported that ZnO nanoparticles can affect the protein profiling of the hematological and the immune systems in juvenile common carp after dietary exposure to ZnO nanoparticles [Citation20]. Meanwhile, using a proteomics technique, it is found that exposure to ZnO nanoparticles can induce rat lung inflammation, which is mainly related to stimulus, transport, inflammatory and immune responses [Citation21]. These findings confirm that proteomics greatly facilitates the understanding of gene function in the post-genomic era and contributes to the understanding of the knowledge of proteins, processes and signaling pathways [Citation22].
In order to evaluate the biological function of proteins and their modifications for understanding signaling mechanisms, we analyzed the alterations of proteomic profiling in ZnO nanoparticle-treated murine photoreceptor cells, performed the bioinformatic analysis, and validated the expressions of several proteins. We focused on the effect of ZnO nanoparticles on mitochondrial functions and our investigations facilitate the understanding of ZnO nanoparticle-induced cytotoxic mechanisms.
Materials and methods
ZnO nanoparticles
ZnO nanoparticles (purity: 99.9%) were provided by Shanghai Fortunebio-tech Co., Ltd (Shanghai, China) and was characterized by a field emission scanning electron microscope (SU8020, Hitachi, Japan). The particle dispersionand the zeta potential were determined using a Malvern Zetasizer (Nano ZS90, Malvern Instruments Ltd., Malvern, UK). Prior to determination, particles were diluted with Dulbecco’s modified Eagle’s medium (DMEM; Thermo Fisher Scientific Beijing, China) containing 1.0 g/L glucose, 10% fetal bovine serum (HyClone, South Logan, UT) and then were sonicated on ice to prevent aggregation.
Cell culture
The murine photoreceptor cell line (661 W) was kindly supplied by Dr Muayyad R. AlUbaidi (University of Oklahoma Health Sciences Center, Oklahoma city, OK,). Cells were cultured in DMEM in a water-saturated incubator supplemented with 5% carbon di oxide (CO2) at 37 °C.
Effects of ZnO nanoparticles on 661 W cells
Cytochrome c release
To measure the cytochrome c release, 5 × 105 cells per well were seeded in a 6-well plate (final volume, 2 mL/well) and were incubated with 0, 31.25, 62.5and 125.0 μmol/L of ZnO nanoparticles for 6 h, respectively. At the indicated time point, cell morphology was first observed by a light field microscope (Olympus IX 71, Tokyo, Japan). The supernatant of each sample was collected and the content of cytochrome c released from cells was determined by a Mouse Cytochrome-c (Cyt-C) ELISA Kit (CSB-E08532m) according to the manufacturer’s instructions (CUSABIO, Biotech Co., Ltd., Wuhan, China). The experiment was repeated three times in triplicate wells.
Determination of adenosine 5′-triphosphate (ATP) level
ATP content was determined using an enhanced ATP assay kit (Beyotime, Shanghai, China) according to the manufacturer’s instructions. Briefly, cells were treated with different concentrations (i.e. 0, 31.25, 62.5 and 125.0 μmol/L) of ZnO nanoparticles for 6 h and were lysed with 100 μL of ATP-releasing reagent in the assay kit, followed by sonication on the ice for 10 min, centrifuged at 12,000 g at 4 °C for 10 min. Subsequently, 20 μL of the supernatant was transferred into a 96-well plate and then 100 μL of ATP detection solution was added to each well. Chemiluminescence was monitored using a microplate reader (Envision, Perkin Elmer, Waltham, MA).
Measurement of mitochondrial membrane potential(ΔΨm)
In this study, changes of ΔΨm were explored using a JC-1 mitochondrial membrane potential assay kit (Beyotime Biotechnology Co., Ltd., Shanghai, China). Briefly, cells were treated with ZnO nanoparticles as described above and then the alterations of ΔΨm were monitored using a JC-1 mitochondrial membrane potential assay kit. ΔΨm was measured using a BD Verse flow cytometer (BD Biosciences, Santa Clara, CA).
Determination of reactive oxygen species (ROS)
To explore the effect of ZnO nanoparticles on cells, the levels of intracellular ROS were determined using 2', 7'-dichlorofluorescin diacetate (DCFH-DA, Invitrogen, Carlsbad, CA) by flow cytometry. In brief, cells were incubated with 0, 31.25, 62.5 and 125.0 µmol/L ZnO nanoparticles for 6 h and then were incubated with 10 µmol/L of DCFH-DA solution in the dark at 37 °C for 30 min, washed with PBS (pH 7.4, 20 mmol/L) twice. Finally, cells were monitored using flow cytometry.
Assay of total antioxidant enzyme activities
After ZnO nanoparticle treatment for 6 h, cells treated with 0, 31.25, 62.5and 125.0 μmol/L ZnO nanoparticles were collected, respectively. Every sample was homogenized in cold saline for determining the activity of total antioxidant enzymes. In this study, total superoxide dismutase activities (including copper-zinc super oxide dismutase and manganese super oxide dismutase) were measured using total superoxide dismutase (T-SOD) assay kit (Nanjing Jiancheng Bioengineering Institute, Nanjing, China). Activities of T-SOD are expressed as units per milligrams of protein (U/mg protein).
Apoptosis/necrosis analysis
To investigate the effect of ZnO nanoparticles on cell apoptosis/necrosis, cells were treated with various concentrations (0, 31.25, 62.5 and 125.0 µmol/L, respectively) of ZnO nanoparticles for 6 h. To assess the apoptosis and/or necrosis, both floating and adherent cells were collected and washed with phosphate buffered saline (PBS) two times, resuspended in 400 µL of Annexin V-fluorescein isothiocyanate (FITC) binding buffer (Bipec Biopharm, Cambridge, MA) at a concentration of 1 × 106 cells/mL. After adding 5 µL of Annexin V-FITC, cells were gently vortexed and incubated at 4–8 °C for 15 min in the dark, and then 10 µL propidium iodide (PI) was added into the cell suspension and then each sample was analyzed by flow cytometry.
Proteomic analysis
Cell culture and sample preparation
In order to investigate the proteomic profiling alterations of target cells exposure to ZnO nanoparticles, 5 × 105 cells per well were seeded in six-well plates and were cultured overnight, then they were treated with various concentrations (i.e. 0, 31.25, 62.5 and 125.0 μmol/L) of ZnO nanoparticles for 6 h. At the indicated time point, cells were harvested, rinsed with PBS solution two times, sonicated on ice for 15 min, centrifuged at 15,000 g for 20 min and later the protein pellet was collected . Finally, 200 μg proteins of pellet from each sample were digested using a filter-aided sample preparation (FASP) method [Citation23] for Liquid chromatography–mass spectrometry (LC-MS)/ mass spectrometry (MS) analysis.
LC-MS/MS analysis and database search
LC-MS/MS analysis was performed as previously described [Citation24]. The datasets were obtained from three LC-MS/MS runs with three independent cell samples. For each resulting set of spectra, SEQUEST was used for peptide sequence assignment. Briefly, every sample was analyzed by Q-Exactive (Thermo Fisher Scientific, Waltham, MA) on a Thermo Scientific Easy-nLC 1000 system. The flow rate was set as 250 nL per min and the column temperature was set as 25 °C. After determination, database search was performed using Proteome Discoverer 1.3 software (Thermo Fisher Scientific Waltham, MA,) with SEQUEST search engine against Swiss-Prot protein sequence database. A two-tailed p values < .05 and a 1.5-fold alteration was regarded as the differentially expressed proteins. To obtain convincible data, three independent experiments of LC-MS/MS analysis were repeated. Every sample in each experiment was determined three times.
Validation of caspase 3, B cell lymphoma gene 2 (Bcl-2), BCL-2-associated protein X (Bax)expressions
To assess the influence of ZnO nanoparticles on cell apoptosis and/or necrosis, we selected three proteins that were identified by mass spectrometry, i.e. caspase 3, Bcl-2, Bax, to validate their expressions using ELISA technique. Briefly, cells (5 × 105 cells per well) were incubated with different concentrations (0, 31.25, 62.5 and 125.0 µmol/L, respectively) of ZnO nanoparticles for 6 h. After harvest, cells were then sonicated on ice for 15 min, followed by centrifugation at 5000 × g for 10 min to collect the supernatants. Measurements of caspase 3, Bax and Bcl-2 protein levels were respectively determined by mouse caspase 3 ELISA kit, mouse Bax ELISA kit and mouse Bcl-2 ELISA kit (all purchased from CUSABIO Biotech Co., Ltd., Wuhan, China).
Moreover, using quantitative PCR (Q-PCR) technique, we also determined the alterations of the three gene (i.e. caspase 3, Bax and Bcl-2) expressions after cells treated with different concentrations of ZnO nanoparticles for 6 h. Briefly, cells were collected and total RNA was extracted, followed by the synthesis of cDNA. Quantitative PCR was performed with cDNA using a SYBR Green I Master kit (Roche, 4707516001, Switzerland) on a LightCycler 480 II sequence detection system (Roche Applied Science, Indianapolis, IN). The primer sequences of caspase 3, Bax, Bcl-2 and GAPDH were as follows: caspase 3 forward: 5′-AGATACCGGTGGAGGCTGACTTC-3′, caspase 3 reverse: 5′-ATTCCGTTGCCACCTTCCTGTTA-3′; Bax forward: 5′-GAGCAGCCGCCCCAGGATG-3′, Bax reverse: 5′-ACGCGGCCCCAGTTGAAGTTG-3′; Bbcl-2 forward: 5′-ATGGGGTGAACTGGGGGAGGATTG-3′, Bcl-2 reverse primer: 5′-AGGGCCAGGCTGAGCAGGGTCTTC-3′and GAPDH forward: 5'-ACGGCAAATTCAACGGCACAGTCA-3′, GAPDH reverse: 5'-TGGGGGCATCGGCAGAAGG-3′. The Q-PCR program was set as follows: 95 °C for 6 min, followed by 45 cycles of denaturation at 95 °C for 20 s, annealing at 58 °C for 20 sand extension at 72 °C for 20 s. Every sample was done in triplicate and the experiment was repeated three times. The mRNA level was calculated as fold change using the 2−ΔΔCt method after normalization to relevant endogenous GAPDH control.
Bioinformatics annotation
Gene ontology (GO) analysis: To perform GO annotation, a panther classification system (http://www.pantherdb.org/) and a GO annotation database website (http://www.geneontology.org) were used to identify the differentially expressed proteins. We set the threshold as a 1.5-fold alteration and two-tailed p values less than .05. The logic algorithm for set operations was applied to further select differentially expressed proteins in accordance with the literature [Citation25].
KEGG pathway analysis: For KEGG pathway enrichment analysis, proteins in ZnO nanoparticle-treated samples with 1.5-fold alteration versus those from the control samples were selected. The differentially expressed proteins were classified according to KEGG function annotation (http://www.genome.jp/kegg/) to identify the signaling pathways.
Protein-protein interaction network analysis: To characterize the protein-protein interaction profile of the differentially expressed proteins among ZnO nanoparticle-treated samples, we further carried out bioinformatics analysis by means of the protein-protein interaction network (http://biodata.mshri.on.ca/osprey/servlet/Index) using STRING data to statistically identify the function and pathways that most strongly associated with the protein list.
Statistical analysis
Results were represented as mean ± standard deviation and either a > 1.5-fold or a < 1/1.5-fold alteration was regarded as the differentially expressed proteins. Statistical analysis was done with unpaired two-tailed Student’s t-tests. p < .05 was used to identify significant alterations.
Results
Characterization of ZnO nanoparticles
ZnO nanoparticles show typical crystalline granules with distinct edges (). The size distribution ranged from 10 to 35 nm in DMEM () and zeta potential was about −7.64 mV ().
Disruption of cell morphology
Cell morphology assay confirmed that the number of living cells treated with ZnO nanoparticles was reduced. In addition, cells exposed to ZnO nanoparticles also showed a tendency to agglomerate: they were shrunken and lost their extended shape and the higher the ZnO nanoparticle concentrations incubated, the more serious the cell morphology disrupted ().
Effect of ZnO nanoparticles on 661 W cells
Release of cytochrome c
Compared with the control sample, cells exposed to ZnO nanoparticles could be apparently induced a significant increase in cytochrome c levels in the cell culture supernatant, accompanied by a concentration-dependent manner (). Meanwhile, we also observed there was a significant difference for cytochrome c content between ZnO nanoparticle-treated samples and the control.
Figure 3. Effects of different concentrations of ZnO nanoparticles on 661 W cells for 6 h. (A) Alterations of cytochrome c levels in cell culture supernatants; (B) Cellular ATP content; (C) Collapse of mitochondrial membrane potential; (D) Analysis of intracellular ROS levels; (E) Alterations of intracellular total antioxidant enzyme activities and (F) Apoptosis/necrosis analysis. Results are shown as mean ± SD of three independent experiments. *p < .05 and **p < .01 versus relevant control.
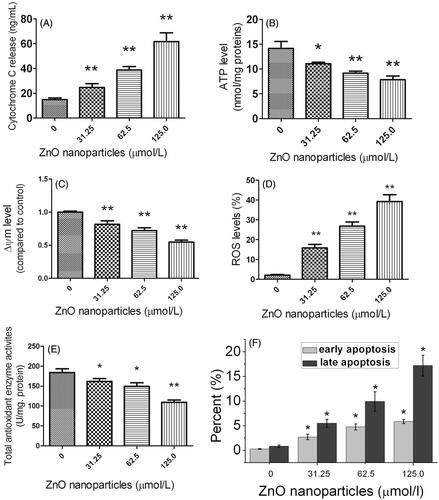
Reduction of ATP levels
Generally, ATP is the mainstream of energy currency within cells and is closely involved in many cellular processes. In this study, we measured the intracellular ATP levels before and after ZnO nanoparticle treatments. shows ZnO nanoparticle-treatment could markedly decrease the ATP generation of murine photoreceptor cells in a concentration-dependent manner, indicating that the cellular function of ATP synthesis has been inhibited by ZnO nanoparticles.
Collapse of ΔΨm
Since cytochrome c was shown to be released from mitochondria, we speculated that the ΔΨm has been altered due to the membrane disruption. The result shows that the percentage of cells with depolarized mitochondria elevated in a dose-dependent manner, whereas the percentage of cells with hyperpolarized mitochondria reduced (). This result indicates that a change of disrupted membrane potential has occurred. The ZnO nanoparticle-induced cytotoxic effect is due to the unbalance of the electric potential between the inner and outer mitochondrial membranes.
Increase of reactive oxygen species (ROS)
The generation of intracellular ROS is always correlated with ΔΨm disruption and cell apoptosis/necrosis. shows cells exposed to ZnO nanoparticles generated much more ROS than that of control cells. The higher concentrations of ZnO nanoparticles incubated with cells, the more ROS generated by the cells. Apparently, overproduction of intracellular ROS will activate the related apoptotic signaling pathway and thereby induce apoptosis.
Decline of total antioxidant enzyme activities
Maintenance of cellular homeostasis depends on the balance between the ROS generation and their clearance. Antioxidant enzymes play a critical role in the clearance of ROS. demonstrates the total antioxidant enzyme activities were declined after exposure to ZnO nanoparticles for 6 h (*p < .05 and **p < .01, respectively), indicating that ZnO nanoparticle-treatment efficiently inhibits the antioxidant enzyme activities.
Elevation of apoptosis/necrosis
Double staining of annexin-V and PI allows separating cells entering early apoptosis and those in late-stage apoptosis/necrosis from living cells. demonstrates ZnO nanoparticle treatment could markedly enhance the levels of both early apoptosis and late apoptosis/necrosis, accompanied by a dose-dependent manner. Importantly, it was noted that exposure to ZnO nanoparticles mainly induces late apoptosis of the cells.
Proteomic analysis
Outline of peptides and proteins identified by LC-MS/MS
The mean values of spectral counts for each protein in both control and cells treated with various ZnO nanoparticles were identified as a characteristic parameter of protein abundance. Summary of the proteomic profiling was shown in . We noted that ZnO nanoparticle-treatment induced more down-regulated proteins than up-regulated proteins.
Table 1. Summary of label-free quantitative proteomic profiling results.
Alterations of mitochondrial proteomic profiling
Based on LS-MS/MS technique, we monitored the alterations of proteomic profiling in murine photoreceptor cells after exposure to ZnO nanoparticles, mainly focused on the mitochondrial proteomic profiling. Results of proteomic analysis revealed that ZnO nanoparticles apparently induced down-regulation of cytochrome c and related proteins as well as the reduction of NADH-related proteins. In addition, we also noted that ZnO nanoparticles could enhance the production of Bax and Bak, whereas reduce Bcl-2, caspase 3, caspase 6, Bcl-XL expressions ().
Validation of caspase 3, bax and bcl-2 molecules
Based on the findings determined by LS-MS/MS, we selected three molecules associated with apoptotic signaling pathway, i.e. Bax, Bcl-2 and caspase 3, to validate their expressions using Q-PCR and ELISA techniques. demonstrates that after exposure to various concentrations of ZnO nanoparticles, Bax and caspase 3 expressions were elevated at mRNA and protein levels, whereas Bcl-2 levels were decreased, indicating that LS-MS/MS, Q-PCR and ELISA show the same tendency.
Figure 5. Determinations of Bax, Bcl-2 and Caspase 3 expressions at mRNA and protein levels after exposure to different concentrations of ZnO nanoparticles for 6 h. (A), (B) and (C) were determined using quantitative PCR, (D), (E) and (F) were determined by ELISA. *p < .05 and **p < .01 versus relevant controls.
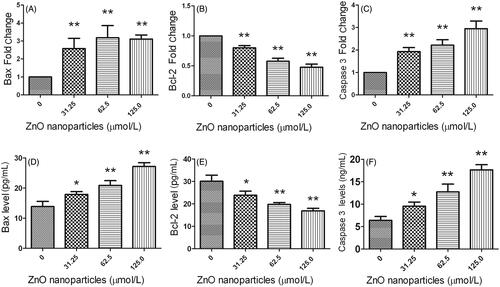
Gene ontology (GO) enrichment analysis
The GO enrichment analysis is a community-based bioinformatics resource that covers several domains of molecular and cellular biology using ontologies to represent biological knowledge. Using GO database, we selected differentially expressed proteins to analyze their molecular function and participation on biological process. Results indicate that most of the up-regulated differentially expressed proteins are mainly involved in cytochrome c oxidase activity for molecular function annotation and were mainly related to hydrogen ion transmembrane transport for biological progress (). Meanwhile, it is noted that most of the down-regulated differentially expressed proteins play crucial roles in cadherin binding involved in cell-cell adhesion for molecular function and is closely associated with cell-cell adhesion for biological process. These results demonstrate that the differentially expressed proteins are mainly involved in mitochondrial functions.
KEGG pathway analysis
The differentially expressed proteins were further classified using KEGG pathway analysis. Results indicated the up-regulated differentially expressed proteins were mainly involved in oxidative phosphorylation, spliceosome, TNF signaling and ribosome, while the down-regulated differentially expressed proteins were mainly involved in ribosome, fatty acid degradation, fatty acid metabolism, ubiquitin mediated proteolysis and citrate cycle (), indicating the differentially expressed proteins are associated with mitochondrial functions.
Analysis of protein–protein interaction networks
Association network analysis for differentially expressed proteins was performed using STRING database. shows that there are two clusters that were mainly associated with mitochondrial functions. One is the cluster related to apoptotic signaling pathway (top left circle), including caspase 3, caspase 6, Bax and Bak1; the other is that linked to cytochrome C-related proteins (bottom right circle), including Uqcrc 1, Uqcrc 2, Ndufs 4and Cox 5 b. Meanwhile, we also noted protein B-cell receptor-associated protein 31 (Bcap31), an endoplasmic reticulum membrane chaperone, is the link between the cluster related to apoptotic signaling pathway and the cluster related to cytochrome c proteins. Therefore, the analysis of protein-protein interaction confirmed the central role of mitochondrial functions in ZnO nanoparticle-induced cell death.
Discussion
ZnO nanoparticles can exhibit cytotoxic effect and induce cell death, one of the mechanisms is involved in ROS-triggered mitochondria-mediated apoptosis [Citation26,Citation27]. Nevertheless, the detailed mechanism remains unclear.
Mitochondria play a critical role in cell survival and are also the sources of ATP generation through the oxidative phosphorylation system. During mitochondrial oxidative phosphorylation, electrons are transferred to oxygen molecules and produce superoxide anions. Due to highly toxicity, superoxide anions are usually neutralized by antioxidant enzymes. Under the pathological conditions, mitochondrial dysfunction leads to ATP depletion, superoxide anion overload and release of proapoptotic molecules including cytochrome c [Citation28]. Cytochrome c is an important electron transporter in the mitochondrial respiratory chain and is also an essential mediator of the intrinsic apoptosis pathway [Citation29]. In our study, we noted that ZnO nanoparticles induce reduction of ATP synthesis and release of cytochrome c, collapse of ΔΨm, elevation of ROS and activation of mitochondria-related apoptotic proteins. All these factors lead to the activation of intrinsic apoptotic pathway.
Oxidative stress is the most discussed paradigm for the nanoparticle-induced toxicity. This can be attributed to their small size because large surface area is usually considered to generate ROS and induce oxidative stress [Citation30]. Under pathological conditions, mitochondria generate excessive ROS, if ROS cannot be degraded thoroughly, the excessive ROS will lead to oxidative stress, inducing oxidation of DNA, membranes, cellular lipids and proteins, damaging their normal physiological functions, ultimately causing cell death [Citation27,Citation31]. In our study, we found that ZnO nanoparticle-treated cells release cytochrome c, produce excessive ROS, decline total antioxidant enzyme activities and collapse ΔΨm, these alterations disrupt the mitochondria homeostasis, leading to the dysfunction of mitochondria. Therefore, the mitochondrial dysfunction will cause the unbalance of continuous protein synthesis and degradation, especially the apoptotic related proteins, finally triggering the activation of intrinsic apoptotic pathway. These findings can also be confirmed by our apoptosis measurement by flow cytometry.
Apoptosis plays a pivotal role in the pathogenesis and occurs through two major pathways: one is the intrinsic pathway, it is also known as the mitochondria-mediated apoptotic pathway, which is initiated due to loss of integrity of the outer mitochondrial membrane; the other is the extrinsic pathway, which can be initiated through ligand binding to cell surface receptors. In mammals, mitochondria play a crucial role in apoptosis that is regulated by Bcl-2 family members [Citation32]. Of the Bcl-2 family members, the proapoptotic proteins (e.g. Bak and Bax) play a role in promoting mitochondria-mediated apoptosis [Citation32,Citation33]. These Bcl-2-related proteins promote permeabilization of the outer membrane of mitochondria, leading to caspase activation and cell death. Du et al. [Citation34] observed that silica nanoparticles could induce H9c2 cardiomyocyte apoptosis by means of the mitochondrial pathway-related apoptosis signaling pathway which is involved in Bcl-2/Bax, cytochrome c and caspase proteins; Choudhury et al. [Citation35] found exposure to ZnO nanoparticles could promote human embryonic kidney (HEK-293) cells release mitochondrial cytochrome c, leading to cell death ); Similarly, we achieve the identical outcomes from cells exposure to ZnO nanoparticles. These results confirm that exposure to ZnO nanoparticles apparently cause cytochrome c release from mitochondria, generate excessive ROS, inhibit the total antioxidant enzyme activities, promote the expressions of Bax, Bak, caspase 3 and caspase-6 and decrease the expression of Bcl-2. Based on these findings, we confirm that exposure to ZnO nanoparticles will trigger the intrinsic mitochondria-mediated apoptotic signaling pathway.
Proteomics is becoming of increasingly importance for the investigation of many physiological functions. Mass spectrometry plays an integral role in the isolation and identification of proteins and their post-translational modifications. Meanwhile, highly complex protein mixtures can also be directly analyzed after proteolysis by LC-MS/MS [Citation36]. Based on LC-MS/MS technique, we explored the alterations of ZnO nanoparticle-treated cell proteomic profiling, performed relative bioinformatics analysis. GO analysis reveals that differentially expressed proteins are closely linked to cytochrome c oxidase activity and hydrogen ion transmembrane transport; KEGG pathway analysis reveals that differentially expressed proteins are associated with oxidative phosphorylation. These findings confirm the ZnO nanoparticle-induced alterations of proteomic profiling are involved in the mitochondrial functions. ZnO nanoparticles also induce the dysfunction of mitochondria, leading to the disruption of mitochondrial homeostasis. The disrupted mitochondrial homeostasis will further initiate apoptotic signaling pathways, leading to cell death. Chupani et al. [Citation37] found that ZnO nanoparticles affect the protein abundances associated with cell motility, immune system response, oxidative stress response and cell metabolism in mitochondrial dysfunction . However, their observations differ in our findings and we suppose these different results may be attributed to the different treatment strategies and species.
Protein-protein interaction maps provide a valuable framework for a better understanding of the functional organization of the proteome. Using protein-protein interaction analysis, we found two clusters are closely associated with mitochondria-related functions. One is the mitochondria-related apoptotic proteins, the other is cytochrome c-related proteins and both are involved in the mitochondria-mediated apoptotic signaling pathway. Moreover, we also noted these two clusters are linked by Bcap31, a protein that is involved in the proliferation and apoptosis of keratinocytes [Citation38] and also interact with membrane-bound form of the immunoglobulin D (mIgD), cellubrevin, major histocompatibility complex class I and Bcl-2/Bcl-X(L), indicating that Bcap31 plays an important role in regulating apoptosis [Citation39]. Therefore, we infer that Bcap 31 is a link in signaling transduction between cytochrome c and pro-apoptotic factors (procaspases, caspase activators and caspase-independent factors such as apoptosis-inducing factor), which finally initiates the mitochondria-mediated apoptotic signaling pathways under ZnO nanoparticle-exposure conditions.
Conclusion
In summary, our results indicate that ZnO nanoparticles promote cytochrome c release, decrease the intracellular ATP level, collapse the mitochondrial membrane potential, generate excessive reactive oxygen species, reduce the total antioxidant enzyme activities, elevate the expressions of both Bax and caspase 3,decrease the level of Bcl-2 and thus enhance the cell apoptosis. The proteomic analysis reveals that differentially expressed proteins are closely associated with oxidative phosphorylation that disrupt the mitochondrial homeostasis, damage mitochondrial functions and finally trigger the mitochondria-mediated apoptotic signaling pathway. Our results confirm that mitochondria play a central role in ZnO nanoparticle-induced murine photoreceptor cell death and will facilitate the understanding of mitochondria-induced cell death.
Acknowledgements
We thank Professor Peiming Gu of Thermo Fisher Scientific (Shanghai) and Shanghai SPR Biolabs, Inc. for the kind assistance in proteomic analysis.
Disclosure statement
No potential conflict of interest was reported by the authors.
Additional information
Funding
References
- Xu D, Liu M, Zou H, et al. A new strategy for fabrication of water dispersible and biodegradable fluorescent organic nanoparticles with AIE and ESIPT characteristics and their utilization for bioimaging. Talanta. 2005;174:803–808.
- Thapa A, Soares AC, Soares JC, et al. Carbon nanotube matrix for highly sensitive biosensors to detect pancreatic cancer biomarker CA19-9. ACS Appl Mater Interfaces. 2017;9:25878–25886.
- Wu Z, Xu XL, Zhang JZ, et al. Magnetic cationic amylose nanoparticles used to deliver survivin-small interfering RNA for gene therapy of hepatocellular carcinoma in vitro. Nanomaterials. 2017;7:110.
- Yurkin ST, Wang Z. Cell membrane-derived nanoparticles: emerging clinical opportunities for targeted drug delivery. Nanomedicine. 2017;12:2007–2019.
- Saraswathi VS, Tatsugi J, Shin PK, et al. Facile biosynthesis, characterization, and solar assisted photocatalytic effect of ZnO nanoparticles mediated by leaves of L. speciosa. J Photochem Photobiol B. 2017;28:89–98.
- Zhao Y, Feng YN, Li L, et al. Tissue-specific regulation of the contents and correlations of mineral elements in hens by zinc oxide nanoparticles. Biol Trace Elem Res. 2017;177:353–366.
- Sonia S, Ruckmani K, Sivakumar M. Antimicrobial and antioxidant potentials of biosynthesized colloidal zinc oxide nanoparticles for a fortified cold cream formulation: A potent nanocosmeceutical application. Mater Sci Eng C Mater Biol Appl. 2017;79:581–589.
- El-Gharbawy RM, Emara AM, Abu-Risha SES. Zinc oxide nanoparticles and a standard antidiabetic drug restore the function and structure of beta cells in Type-2 diabetes. Biomed Pharmacother. 2016;84:810–820.
- Guo D, Wu C, Jiang H, et al. Synergistic cytotoxic effect of different sized ZnO nanoparticles and daunorubicin against leukemia cancer cells under UV irradiation. J Photochem Photobiol B. 2008;93:119–126.
- Hassan HFH, Mansour AM, Abo‐Youssef AMH, et al. Zinc oxide nanoparticles as a novel anticancer approach; in vitro and in vivo evidence. Clin Exp Pharmacol. 2017;44:235–243.
- Wang D, Guo D, Bi H, et al. Zinc oxide nanoparticles inhibit Ca2+-ATPase expression in human lens epithelial cells under UVB irradiation. Toxicol In Vitro. 2013;27:2117–2126.
- Zhang J, Qin X, Wang B, et al. Zinc oxide nanoparticles harness autophagy to induce cell death in lung epithelial cells. Cell Death Dis. 2017;8:e2954.
- Guo D, Bi H, Wu Q, et al. Zinc oxide nanoparticles induce rat retinal ganglion cell damage through bcl-2, caspase-9 and caspase-12 pathways. J Nanosci Nanotechnol. 2013;13:3769–3777.
- Guo DD, Li QN, Li CM, et al. Zinc oxide nanoparticles inhibit murine photoreceptor-derived cell proliferation and migration via reducing TGF-β and MMP-9 expression in vitro. Cell Prolif. 2015;48:198–208.
- Acin-Perez R, Salazar E, Kamenetsky M, et al. Cyclic AMP produced inside mitochondria regulates oxidative phosphorylation. Cell Metab. 2009;9:265–276.
- Sun L, Yuan Q, Xu T, et al. Pioglitazone improves mitochondrial function in the remnant kidney and protects against renal fibrosis in 5/6 nephrectomized rats. Front Pharmacol. 2017;8:545.
- Suhaili SH, Karimian H, Stellato M, et al. Mitochondrial outer membrane permeabilization: a focus on the role of mitochondrial membrane structural organization. Biophys Rev. 2017;9:443–457.
- Düssmann H, Perez-Alvarez S, Anilkumar U, et al. Single-cell time-lapse imaging of intracellular O2 in response to metabolic inhibition and mitochondrial cytochrome-c release. Cell Death Dis. 2017;8:e2853.
- Guo DD, Hu B, Tang HY, et al. Proteomic profiling analysis reveals a link between experimental autoimmune uveitis and complement activation in rats. Scand J Immunol. 2017;85:331–342.
- Chupani L, Zusková E, Niksirat H, et al. Effects of chronic dietary exposure of zinc oxide nanoparticles on the serum protein profile of juvenile common carp (Cyprinus carpio L.). Sci Total Environ. 2017;579:1504–1511.
- Juang YM, Lai BH, Chien HJ, et al. Changes in protein expression in rat bronchoalveolar lavage fluid after exposure to zinc oxide nanoparticles: an iTRAQ proteomic approach. Rapid Commun Mass Spectrom. 2014;28:974–980.
- Pandey A, Mann M. Proteomics to study genes and genomes. Nature. 2000;405:837–846.
- Wiśniewski JR, Zougman A, Nagaraj N, et al. Universal sample preparation method for proteome analysis. Nat Methods. 2009;6:359–362.
- Guo D, Gu P, Liu Z, et al. Proteomic analysis of rat plasma with experimental autoimmune uveitis based on label-free liquid chromatography-tandem mass spectrometry (LC-MS/MS). J Chromatogr B. 2015;976:84–90.
- Mi H, Muruganujan A, Thomas PD. PANTHER in 2013: modeling the evolution of gene function, and other gene attributes, in the context of phylogenetic trees. Nucleic Acids Res. 2012;41:377–D386.
- Yu KN, Yoon TJ, Minai-Tehrani A, et al. Zinc oxide nanoparticle induced autophagic cell death and mitochondrial damage via reactive oxygen species generation. Toxicol In Vitro. 2013;27:1187–1195.
- Guo D, Bi H, Liu B, et al. Reactive oxygen species-induced cytotoxic effects of zinc oxide nanoparticles in rat retinal ganglion cells. Toxicol In Vitro. 2013;27:731–738.
- Khalil B, Liévens JC. Mitochondrial quality control in amyotrophic lateral sclerosis: towards a common pathway? Neural Regen Res. 2017;12:1052–1061.
- Muneeswaran G, Kartheeswaran S, Pandiaraj M, et al. Investigation of structural dynamics of Thrombocytopenia Cargeeg mutants of human apoptotic cytochrome c: a molecular dynamics simulation approach. Biophys Chem. 2017;230:117–126.
- Akhtar MJ, Ahamed M, Kumar S, et al. Zinc oxide nanoparticles selectively induce apoptosis in human cancer cells through reactive oxygen species. Int J Nanomed. 2012;7:845–857.
- Moris D, Spartalis M, Tzatzaki E, et al. The role of reactive oxygen species in myocardial redox signaling and regulation. Ann Transl Med. 2017;5:324.
- Youle RJ, Strasser A. The BCL-2 protein family: opposing activities that mediate cell death. Nat Rev Mol Cell Biol. 2008;9:47–59.
- Someya S, Xu J, Kondo K, et al. Age-related hearing loss in C57BL/6J mice is mediated by Bak-dependent mitochondrial apoptosis. Proc Natl Acad Sci USA. 2009;106:19432–19437.
- Du ZJ, Cui GQ, Zhang J, et al. Inhibition of gap junction intercellular communication is involved in silica nanoparticles-induced H9c2 cardiomyocytes apoptosis via the mitochondrial pathway. I Int J Nanomed. 2017;12:2179–2188.
- Choudhury SR, Ordaz J, Lo CL, et al. From the cover: zinc oxide nanoparticles-induced reactive oxygen species promotes multimodal cyto-and epigenetic toxicity. Toxicol Sci. 2017;156:261–274.
- Peng J, Elias JE, Thoreen CC, et al. Evaluation of multidimensional chromatography coupled with tandem mass spectrometry (LC/LC-MS/MS) for large-scale protein analysis: the yeast proteome. J Proteome Res. 2003;2:43–50.
- Chupani L, Niksirat H, Lünsmann V, et al. Insight into the modulation of intestinal proteome of juvenile common carp (Cyprinus carpio L.) after dietary exposure to ZnO nanoparticles. Sci Total Environ. 2017;613-614:62–71.
- Ruchusatsawat K, Thiemsing L, Mutirangura A, et al. BCAP 31 expression and promoter demethylation in psoriasis. Asian Pac J Allergy Immunol. 2017;35:86–90.
- Song C, Yan B, Chen L, et al. Novel immunohistochemical monoclonal antibody against rat B cell receptor Associated Protein 31 (BAP31). Hybridoma. 2009;28:363–367.