Abstract
The efficacy of anticancer drugs is rather limited in the treatment of brain glioma due to the hindrance of the blood–brain barrier (BBB). Herein, we reported an easy formulation of functional docetaxel nanomicelles for the treatment of brain glioma using a graft copolymer soluplus as basic material through dual-modifications with a glucose-lipid derivative and a dequalinium-lipid derivative. The studies were performed on brain glioma U87MG cells, in vitro BBB models and brain glioma-bearing nude mice. The functional docetaxel nanomicelles were approximately 100 nm. The results demonstrated that the functional docetaxel nanomicelles could transport across the BBB, enhance the cellular uptake, target to the mitochondria, induce the apoptosis, increase the cytotoxicity in the brain glioma cells, and extend survival span of the brain glioma-bearing mice. The action mechanisms were associated with dual-modifications by the glucose-lipid derivative and the dequalinium-lipid derivative, both of which are beneficial for the transport across the BBB. Furthermore, the modification with dequalinium-lipid derivative was able to target to the brain glioma cells and to the mitochondria. In conclusion, the functional docetaxel nanomicelles would be a promising formulation for the treatment of brain glioma, deserving further development for clinical trials.
Introduction
Brain glioma is an invasive high-grade malignant cancer, and the complete surgical removal of brain glioma is virtually impossible [Citation1]. Accordingly, chemotherapy plays an important role in eliminating the residual cancer cells during the clinical treatment of brain glioma [Citation2]. However, treatment efficacy of the anticancer drugs is rather limited in the treatment of brain glioma due to the hindrance of the blood–brain barrier (BBB), which is a highly tight physiological barrier in preventing the entrance of foreign drugs [Citation3–5]. How to improve the transport of anticancer drug across the BBB remains a major scientific issue [Citation6].
In this study, we hypothesized that a kind of functional docetaxel nanomicelles could transport across the BBB, and further eliminate the brain glioma cells. In this construct, a graft copolymer, soluplus (polyvinyl caprolactam-polyvinyl acetate-polyethylene glycol graft copolymer), was used for building the nanomicelles, and docetaxel was used as the anticancer drug. Moreover, a glucose-lipid derivative, and a dequalinium-lipid derivative were used as the functional materials for transferring drug nanomicelles across the BBB and for further improving the cellular uptake of drug nanomicelles by brain glioma cells.
Soluplus is a water-soluble copolymer with an average molecular weight ranging from 90,000 to 140,000 g/mol [Citation7]. Soluplus has a hydrophobic core, which is able to entrap hydrophobic drug. In this research, soluplus is used as basic material to form nanomicelles, and a broad-spectrum anticancer drug docetaxel (DTX) is used as a hydrophobic drug for constructing the drug nanomicelles.
The BBB is a highly selective semipermeable membrane barrier that is formed by brain endothelial cells and it allows the passage of water, and the selective transport of nutrients, for instance, sugars and amino acids [Citation8]. Recent studies have revealed that a family of structurally related facilitative stereospecific glucose transporters (GLUT) mediate the intake of glucose in brain [Citation9,Citation10]. Among these, GLUT1 isoform, which expresses in the luminal surface of brain capillaries [Citation11], can increase the transport of drug carriers across the BBB. In this study, a kind of glucose-vitamin E-derivative (4-aminopheny l-β-d-glucopyranoside-d-a-tocopheryl polyethylene glycol 1000 succinate, TPGS1000-GLU) conjugate has been modified onto the nanomicelles to transport the drug-loaded nanomicelles across the BBB.
Dequalinium (DQA) is a quaternary ammonium cation with the cationic charge centre. Our previous studies have demonstrated that DQA-lipid derivative can increase the cellular uptake and accumulate in mitochondria by electrostatic interactions [Citation12,Citation13]. In this study, dequalinium lipid derivative was synthesized by conjugating dequalinium with polyethylene glycol 2000 distearoyl phosphatidyl ethanolamine (DSPE- PEG2000) for enhancing the uptake of drug nanomicelles by brain glioma cells.
The objectives of the present study were to develop a kind of functional docetaxel nanomicelles, evaluate the effects on the treatment of brain glioma, and reveal the relevant mechanisms.
Materials and methods
Materials, cell line and animals
Docetaxel (DTX) was purchased from Xiezun Chem. Co., Ltd (Nanjing, China). Soluplus was provided by Fengli Jingqiu Pharmaceutical Co., Ltd. (Beijing, China). 4-aminophenyl-β-d-glucopyranoside (GLU) was purchased from J&K Scientific Ltd. (Beijing, China). d-α-Tocopherol polyethylene glycol 1000 succinate (TPGS1000), dequalinium (DQA), coumarin-6 (coumarin) and 1,1′-dioctadecyl-3,3,3′,3′-tetramethylindotricarbocyanine iodide (DiR) were purchased from Sigma–Aldrich (Saint Louis, MO). DSPE-PEG2000 and DSPE-PEG2000-NHS were purchased from NOF Corporation (Kanagawa, Japan).
Brain glioma U87MG cells were provided by Institute of Basic Medical Science, Chinese Academy of Medical Science (Beijing, China) and were cultured in minimum essential medium eagle (MEM) supplemented with 10% foetal bovine serum (FBS) and 1% non-essential amino acids (Macgene, Beijing, China). Murine brain microvascular endothelial cells (BMVECs) were provided by the Institute of Clinical Medical Sciences, China-Japan Friendship Hospital (Beijing, China) and were cultured in Dulbecco's modified eagle medium (DMEM) supplemented with 20% FBS, 40 U/mL heparin, 100 μg/mL endothelial cell growth factor (EGF), 100 U/mL penicillin, and 100 μg/mL streptomycin. The cells were cultured at 37 °C in a humidified atmosphere containing 5% CO2.
Male BALB/c nude mice (18–20 g) were obtained from Department of Laboratory Animal Science, Peking University (Beijing, China). All of the animal experiments adhered to the principles of care and use of laboratory animals, and were approved by the Institutional Animal Care and Use Committee of Peking University.
Nanomicelles
TPGS1000-GLU and DSPE-PEG2000-DQA were synthesized according to our previous research [Citation12,Citation14]. Four types of nanomicelles were fabricated, including docetaxel nanomicelles, GLU modified docetaxel nanomicelles, DQA modified docetaxel nanomicelles, and functional docetaxel nanomicelles. To prepare functional docetaxel nanomicelles, soluplus, docetaxel, TPGS1000-GLU and DSPE-PEG2000-DQA (30:1:2:2, w/w) were dissolved in methanol in a pear-shaped bottle. The methanol was removed using a rotary vacuum evaporator and the thin film was hydrated with deionized water, followed by moderate stirring for 2 h. To prepare fluorescence labelled functional nanomicelles, soluplus, coumarin (or DiR), TPGS1000-GLU and DSPE-PEG2000-DQA were dissolved at a mass ratio of 300:1:20:20, followed by the same procedures as described above. Other types of nanomicelles were similarly prepared.
The nanomicelles were observed using an atomic force microscope (AFM) (SPI3800N series SPA-400, NSK Ltd., Tokyo, Japan) and a transmission electron microscopy (TEM) (Tecnai G2 20ST, FEI Co., Japan). The size and zeta potential of the nanomicelles were measured using a Nano Series Zenith 4003 Zetasizer (Malvern Instruments Ltd., Malvern, UK). The encapsulation efficiency of docetaxel was measured by a high-performance liquid chromatography system (Agilent Technologies Inc., Cotati, CA) and an XDB-C18 column (Agilent, 250 × 4.6 mm, 5 µm). The mobile phase for measuring docetaxel consisted of acetonitrile, methanol and deionized water (35:40:25, v/v), and the detection wavelength was set at 232 nm. Encapsulation efficiency (EE) of docetaxel was calculated as EE (%) = (We/Wtotal) × 100%, where We is the measured amount of docetaxel in the nanomicelle suspensions after passing over a Sephadex G-50 column, and Wtotal is the total amount of docetaxel in an equal volume of initial nanomicelle suspensions.
In vitro release rates of docetaxel in the nanomicelles were determined at 37 °C for 36 h by dialysis against phosphate buffered saline (137 mM NaCl, 2.7 mM KCl, 8 mM Na2HPO4 and 2 mM KH2PO4, PBS pH 7.4) containing 10% FBS. The release rate (RR) in vitro of docetaxel from the nanomicelles was calculated using the formula RR(%) = (Wi/Wtotal) × 100%, where Wi is the measured amount of docetaxel at the ith time-point in the release medium, and Wtotal is the total amount of docetaxel in an equal volume of nanomicelle suspensions before dialyses.
Transport ability across the BBB
In vitro BBB model was established according to our previous research [Citation15]. Briefly, BMVECs were seeded onto the gelatin solution-coated polyester membrane inserts of transwell plates (Corning, NY) at a density of 1 × 104 cells/well, and incubated for 6 days. The culture medium was changed every other day. Then, the trans-epithelial electric resistance (TEER) was measured using Epithelial Vol-Ohm Meter (Millicell ERS-2, Millipore, Billerica, MA) to monitor the tightness and integrity of the BBB model. The model was able to be applied for experiments when the TEER values reached 200 Ω·cm2. Then, brain glioma U87MG cells were seeded at the bottom of the plates at a density of 2 × 104 cells/well. After incubation for 24 h, varying nanomicelle formulations were added to the upper inserts at a concentration of 5 μM docetaxel. Blank culture medium was added as blank control. After incubation for 4 h, the inserts were moved away, and brain glioma U87MG cells were further incubated for 24 h. Then the inhibition rate of brain glioma U87MG cells was determined using the sulforhodamine B (SRB) staining assay [Citation16].
Cellular uptake
Coumarin was used as the fluorescent probe and encapsulated into the four types of nanomicelles to observe the cellular uptake of the nanomicelles. Briefly, brain glioma U87MG cells were seeded in 6-well plates at a density of 3 × 105 cells/well, and cultured for 24 h. Then, the culture medium were replaced with fresh medium containing coumarin nanomicelles, GLU modified coumarin nanomicelles, DQA modified coumarin nanomicelles, or functional coumarin nanomicelles at a concentration of 2 μM coumarin. After incubation for 4 h, the cells were harvested and re-suspended in PBS (pH 7.4). The fluorescence intensity in the cells was measured by a flow cytometry (Becton Dickinson FACS Calibur, Mountain View, CA).
To obtain visual images of cellular uptake, brain glioma U87MG cells were seeded in glass bottom dishes (φ = 15 mm; Nest, Beijing, China) at a density of 1.5 × 105 cells per dish, and then cultured for 24 h. Four types of coumarin-labelled nanomicelles were added into the dishes at a concentration of 2 μM coumarin, respectively. After incubation for another 4 h, the cells were washed with PBS and stained with Hoechst 33342 (Invitrogen, Beijing, China) at 37 °C for 15 min. The images were obtained using a confocal laser scanning fluorescence microscope (Leica, Heidelberg, Germany).
Cytotoxicity
Cytotoxicity of the nanomicelles was evaluated using the SRB assay. Brain glioma U87MG cells were seeded in 96-well plates at a density of 5 × 103 cells/well, and cultured for 24 h. Then, docetaxel nanomicelles, GLU modified docetaxel nanomicelles, DQA modified docetaxel nanomicelles, and functional docetaxel nanomicelles were separately added into each well at varying concentrations ranging from 0 to 10 μM docetaxel. Blank culture medium was used as blank control. After incubation for 48 h, the cells were washed with deionized water, and fixed with 10% trichloroacetic overnight. Then the cells were stained with SRB and the absorbance of each well was measured using a microplate reader (Infinite F50, Tecan Group Ltd., England) at a wavelength of 540 nm. Survival rate = (A540nm of treated well/A540nm of blank control well) × 100%, where A540nm refers to absorbance value at 540 nm.
To further investigate the cytotoxicity of the nanomicelles, multicellular glioma spheroids model was established. Briefly, agarose was added into serum free MEM culture medium and heated to 80 °C for 30 min to form 2% (w/v) solution, and 50 μL of the solution was added into each well of the 96-well plates to coat the well with a thin layer at the bottom. Then, brain glioma U87MG cells were seeded into each well at a density of 1 × 103 cells/well with 100 μL culture medium. The plates were then gently shaken for 2 min and the cells were incubated for 4 days to form brain glioma spheroids. Then, docetaxel nanomicelles, GLU modified docetaxel nanomicelles, DQA modified docetaxel nanomicelles, and functional docetaxel nanomicelles were separately added into each well at a concentration of 5 μM docetaxel. Blank culture medium was added as a blank control. After incubation for 48 h, the spheroids were fixed by 2.5% glutaraldehyde for 60 min, rinsed three times by PBS (pH7.4), dehydrated, and embedded. Afterwards, the spheroids were imaged using a scanning electron microscope (SEM, JSM-5600 LV, JEOL, Japan).
Induction of apoptosis
Apoptosis of brain glioma cells induced by the anticancer drug was identified using a fluorescein annexin V staining kit (KeyGen Biotechnology, Beijing, China). Briefly, brain glioma U87MG cells were seeded at 5 × 105 cells/well into six-well culture plates, and incubated for 24 h at 37 °C. Then, the cells were exposed to docetaxel nanomicelles, GLU modified docetaxel nanomicelles, DQA modified docetaxel nanomicelles, and functional docetaxel nanomicelles for 6 h. The final concentration of docetaxel was 1 μM. Blank culture medium was used as a blank control. After incubation for 12 h, the cells were assessed by the flow cytometry according to manufacturer instructions. Each assay was repeated in triplicate.
The expressions of apoptotic enzymes in the brain glioma cells were performed using Western blotting test. Briefly, the cells were treated with the varying nanomicelles. Control experiment was performed by adding blank culture medium. The final concentration of docetaxel was 1 μM. After incubation for 12 h, the cells were harvested and lysed. Total protein concentrations were determined using a bicinchoninic acid (BCA) protein assay at 540 nm according to the manufacturer's instruction. After separation by NuPAGE Bis-Tris Gels (Meilunbio, Dalian, China), the proteins were transferred to polyvinylidene fluoride membranes (Millipore, Billerica, MA) by electrophoresis. Then the membranes were incubated with primary antibodies including caspase 8, caspase 9 and caspase 3 (Bioss, Beijing, China) overnight at 4 °C. Then, the membranes were incubated in horseradish peroxidase conjugated secondary antibodies for 1 h at room temperature. Signals were detected using the Pierce ECL Western blotting substrate (Meilunbio, Dalian, China).
Co-localization with mitochondria
Brain glioma U87MG cells were seeded in glass bottom dishes at a density of 1.5 × 105 cells in each dish, and then cultured for 24 h. The culture media were replaced with fresh media containing coumarin nanomicelles, GLU modified coumarin nanomicelles, DQA modified coumarin nanomicelles or functional coumarin nanomicelles at a concentration of 2 μM coumarin. After incubation for 4 h, the cells were washed with PBS (pH7.4) and stained with MitoTracker Deep Red FM (Invitrogen, Beijing, China) at 37 °C for 1 h and then Hoechst 33342 at 37 °C for 15 min. The images for co-localization with mitochondria were obtained using the confocal laser scanning fluorescence microscope.
Brain glioma-bearing mouse model
To investigate the efficacy of the nanomicelles in vivo, a brain glioma-bearing mouse model was established. Male BALB/c nude mice (18–20 g) were included for the study. Briefly, mice were anesthetized by intraperitoneal injection of 4% chloral hydrate (10 μL/g), and fixed on a stereotaxic apparatus (RWD Life Science, Shenzhen, China). Then an incision was made to expose the cranium, and the cranium was drilled 1 mm to the posterior, 2 mm to the right of the bregma and 3 mm in depth. Brain glioma U87MG cells (1 × 105 cells/μL, 3 μL per mouse) were injected into the brain at a rate of 1 μL/min. Then the burr hole was covered with bone wax, and the incision was sutured.
In vivo distribution in brain glioma-bearing mice
To investigate the in vivo distribution of the nanomicelles in brain glioma-bearing mice, DiR was incorporated into the nanomicelles as the fluorescent probe. The brain glioma-bearing mice were made as above, and after 10 days, the mice were randomly divided into 3 groups and administered with free DiR, DiR nanomicelles or functional DiR nanomicelles by tail intravenous injection (1 μg DiR per mouse). The mice were scanned at 0, 8, 16 and 24 h using an IVIS Spectrum Preclinical In Vivo Imaging System (Perkin Elmer, Waltham, USA). The mice were then sacrificed, and the major organs, including the brains, hearts, livers, spleens, lungs and kidneys, were obtained and scanned the same way above.
Treatment efficacy and safety evaluation
To study the overall treatment efficacy of the nanomicelles, brain glioma-bearing mice were randomly divided into four groups (6 mice/group) at day 10 after inoculation. Physiological saline, free docetaxel, docetaxel nanomicelles and functional docetaxel nanomicelles were administered to mice by tail intravenous injection (5 mg/kg). Treatments were applied every other days (four times in total). The mice were observed, and then the survival rates were calculated and the survival curve was made accordingly.
To evaluate the potential toxicity of the functional nanomicelles, the hearts, livers, spleens, lungs, and kidneys were removed from sacrificed mice after treatment. The tissues were fixed using 4% paraformaldehyde solution, and decalcified in 10% ethylene diamine tetraacetic acid disodium (EDTA-2Na). Then the organs were made into paraffin sections (4 μm in thickness) and stained with hematoxylin-eosin (H&E). The images of the organ sections were obtained using a Caikon XDS-300 C system (Caikon, Beijing, China).
Statistical analysis
The data were represented as means ± standard deviations (SD). Analysis of variance (ANOVA) was used to determine the significance among groups, after which post hoc tests with the Bonferroni correction were used for multiple comparisons between individual groups. A value of p < .05 was considered to be significant.
Results
Nanomicelles
The nanomicelles were mainly formed by soluplus, and docetaxel was encapsulated into the hydrophobic core of the nanomicelles (). Besides, the lipophilic parts of DSPE-PEG2000-DQA and TPGS1000-GLU were decorated into the nanomicelles, while the functional parts were exposed outside of the nanomicelles to interact with the BBB and brain glioma cells.
Figure 1. Characterization of functional docetaxel nanomicelles. (A) Structural representation of functional docetaxel nanomicelles; (B) size distribution; (C) atomic force microscope image; (D) transmission electron microscope image; (E) release rates of docetaxel from nanomicelles. Data are presented as mean ± standard deviation (SD) (n = 3).
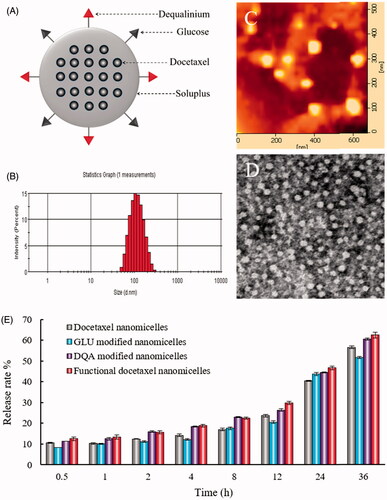
To investigate the physicochemical properties of the nanomicelles, the encapsulation efficiency, size, polydispersity index (PDI) and zeta potential were characterized (, ). In all nanomicelles, the encapsulation efficiencies of docetaxel were greater than 94%. The average particle size of functional docetaxel nanomicelles was approximately 100 nm, with a narrow PDI (< 0.20). The zeta potential values of functional docetaxel nanomicelles and DQA modified docetaxel nanomicelles were 3.32 ± 0.87 mV and 5.14 ± 0.34 mV, while those of docetaxel nanomicelles and GLU modified docetaxel nanomicelles were −3.37 ± 1.60 mV and −4.50 ± 4.13 mV. AFM and TEM images indicated that functional docetaxel nanomicelles () were round and uniform with smooth surfaces. The nanomicelles showed a sustained release behaviour. At 36 h, the drug release ratios of all the nanomicelles were approximately 60% ().
Table 1. Characterization of the functional docetaxel nanomicelles.
Transport ability across the BBB
Transport ability of the nanomicelles across the BBB was investigated on the established BBB model (). TEER values were used to monitor the integrity of the BBB, and a TEER value above 200 Ω·cm2 indicated a successful in vitro BBB model. In this model, BMVECs were grown in the upper compartment, while brain glioma U87MG cells were grown in the lower compartment of the BBB insert (). Drug treatments were applied to the upper compartment of the BBB insert, and the survival rates of the brain glioma cells indicated the transport capability of the nanomicelles across the BBB. The rank of survival rates was docetaxel nanomicelles > GLU modified docetaxel nanomicelles > DQA modified docetaxel nanomicelles > functional docetaxel nanomicelles (), indicating that functional docetaxel nanomicelles had the strongest ability to transport across the BBB.
Figure 2. Transport ability of functional docetaxel nanomicelles across the BBB model. (A) Schematic representation of the BBB model. (B) Functional docetaxel nanomicelles transporting across the BBB. (C) Transport ability indicated by the survival rate of brain glioma U87MG cells after drug transport across the BBB. p < .05, a, vs. 1; b, vs. 2; c, vs. 3. Data presented as mean ± standard deviation (n = 3). BBB is the abbreviation of the blood–brain barrier; BMVECs is the abbreviation of the brain microvascular endothelial cells; DQA is dequalinium.
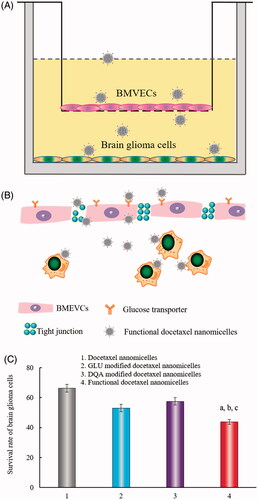
Cellular uptake
The cellular uptake of nanomicelles by brain glioma U87MG cells was assayed with the flow cytometry, and coumarin was encapsulated into nanomicelles as fluorescent probe. After the treatment with varying nanomicelles, the fluorescence intensity in brain glioma U87MG cells was measured. The results demonstrated that the functional coumarin nanomicelles were internalized more than other control groups (). The rank of cellular uptake was functional coumarin nanomicelles > DQA modified coumarin nanomicelles > GLU modified coumarin nanomicelles > coumarin nanomicelles.
Figure 3. Cellular uptake by brain glioma cells. (A) Cellular uptake by brain glioma U87MG cells observed with flow cytometry. (a) Coumarin nanomicelles; (b) GLU modified coumarin nanomicelles; (c) DQA modified coumarin nanomicelles; (d) functional coumarin nanomicelles. (B) Cellular uptake by brain glioma U87MG cells under fluorescence microscope. (B1) Coumarin nanomicelles; (B2) GLU modified coumarin nanomicelles; (B3) DQA modified coumarin nanomicelles; (B4) Functional coumarin nanomicelles. Courmarin was used as a fluorescent probe.
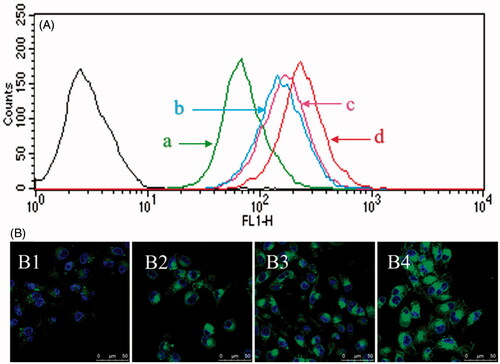
To directly observe cellular uptake of the nanomicelles by brain glioma U87MG cells, the cells were imaged under the confocal laser scanning fluorescence microscope. The results demonstrated that the cells of functional coumarin nanomicelles showed the strongest fluorescence signals (). The rank of fluorescence intensity was consistent with that from the flow cytometry.
Cytotoxicity
The cytotoxicity of the nanomicelles on brain glioma U87MG cells was investigated using a SRB assay. The cells were treated with varying nanomicelles at a series of docetaxel concentrations (0–10 μM). The results showed that functional docetaxel nanomicelles demonstrated the strongest cytotoxicity on the brain glioma cells (). The ranking for cytotoxicity was functional docetaxel nanomicelles > DQA modified docetaxel nanomicelles > GLU modified docetaxel nanomicelles > docetaxel nanomicelles.
Figure 4. Cytotoxicity to brain glioma cells and the glioma spheroids after treatment with functional docetaxel nanomicelles. (A) Cytotoxic effect to brain glioma U87MG cells; p < .05, a, vs. docetaxel nanomicelles; b, vs. GLU modified docetaxel nanomicelles; c, vs. DQA modified docetaxel nanomicelles. Data presented as mean ± standard deviation (SD), (n = 6). (B) Cytotoxic effect to brain glioma U87MG glioma spheroids. (B1) Docetaxel nanomicelles; (B2) GLU modified docetaxel nanomicelles; (B3) DQA modified docetaxel nanomicelles; (B4) functional docetaxel nanomicelles.
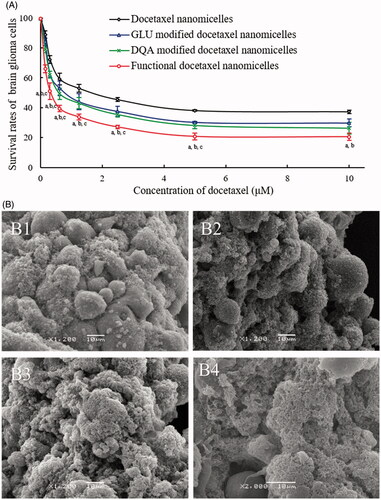
The cytotoxicity of the nanomicelles on the multicellular glioma spheroids was further evaluated. After treatment with varying nanomicelles, the ranking for cytotoxicity was functional docetaxel nanomicelles () > DQA modified docetaxel nanomicelles () > GLU modified docetaxel nanomicelles () > docetaxel nanomicelles (), demonstrating that the functional docetaxel nanomicelles had the strongest destructing effect to the brain glioma spheroids as well.
Induction of apoptosis
The induced apoptosis of brain glioma cells was also evaluated by the flow cytometry (). After incubation with blank medium (control), docetaxel nanomicelles, GLU modified docetaxel nanomicelles, DQA modified docetaxel nanomicelles, and functional docetaxel nanomicelles for 12 h, the total percentages of apoptosis in the brain glioma U87MG cells were 1.73 ± 0.17%, 8.37 ± 0.97%, 11.96 ± 0.37%, 13.18 ± 0.71%, and 17.54 ± 2.16%, respectively.
Figure 5. Induction of apoptosis on brain glioma cells after treatment with functional docetaxel nanomicelles. (A) Induction of apoptosis; (B) activation of apoptotic enzymes upstream caspases 8, 9 and downstream caspase 3. 1. Blank control; 2. Docetaxel nanomicelles; 3. GLU modified docetaxel nanomicelles; 4. DQA modified docetaxel nanomicelles; 5. Functional docetaxel nanomicelles. p < .05, a, vs. 1; b, vs. 2; c, vs. 3; d, vs. 4. Data presented as mean ± standard deviation (SD), (n = 3).
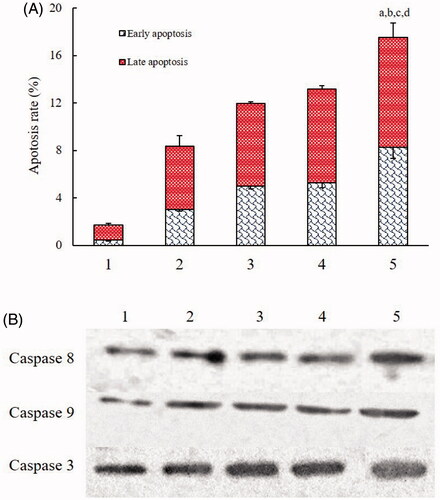
The induced apoptosis was further confirmed by the activated apoptotic enzymes by Western blotting test. The results demonstrated that the functional docetaxel nanomicelles could significantly increase the expression of apoptotic enzymes caspase 8, caspase 9 and caspase 3 (). Besides, GLU modified docetaxel nanomicelles and DQA modified docetaxel nanomicelles could also increase the expression of caspases.
Co-localization with mitochondria
The co-localization with mitochondria was evaluated using the confocal laser scanning fluorescence microscopy. The images were used to observe the co-localization of fluorescent probe-labelled nanomicelles in the mitochondria (), and the quantitative co-localization rates were calculated by evaluating the overlapping between the red (mitotracker) and green (coumarin) fluorescence (). The results showed that the ranking for co-localization with mitochondria was functional coumarin nanomicelles (54.95%) > DQA modified coumarin nanomicelles (53.57%) > GLU modified coumarin nanomicelles (38.95%) > coumarin nanomicelles (30.39%).
In vivo distribution in brain glioma-bearing mice
The in vivo distribution of the nanomicelles was investigated on brain glioma-bearing nude mice after intravenous administration of varying fluorescent probe (DiR)-labelled nanomicelles at indicated time points. The mice were observed using the in vivo imaging system (). The results showed that the functional DiR nanomicelles exhibited the strongest fluorescence intensity in the brain as compared to the control formulations. The mice were sacrificed at 24 h after administrations, major organs were isolated for ex vivo imaging. The results demonstrated that the functional DiR nanomicelles could accumulate in the brain glioma site. In contrast, the control formulations exhibited less distribution in the brain. In addition, the control formulations mainly accumulated in the livers.
Figure 7. In vivo imaging and efficacy on brain glioma-bearing nude mice after treatment with functional nanomicelles. (A) In vivo imaging of glioma-bearing nude mice after administration of functional DiR nanomicelles; (B) Kaplan–Meier survival curves of brain glioma-bearing nude mice after treatment with functional docetaxel nanomicelles; (C) Preliminary safety evaluation on major organs after treatment with physiological saline and functional docetaxel nanomicelles (hematoxylin-eosin staining).
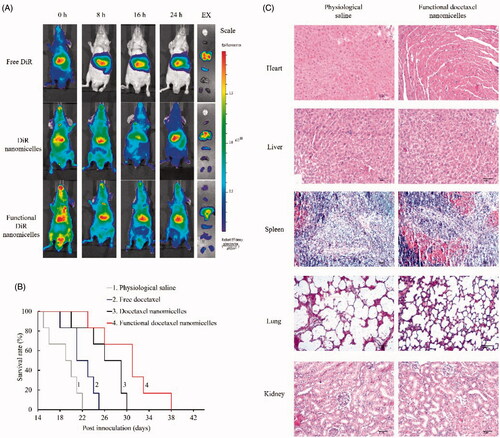
Treatment efficacy and safety evaluation
The treatment efficacy of the nanomicelles was evaluated by the survival time of the brain glioma-bearing mice after treatment with varying formulations, and Kaplan–Meier survival curves were plotted. The results showed the ranking for survival days () after intravenous administration was functional docetaxel nanomicelles > docetaxel nanomicelles > free docetaxel > physiological saline, indicating that the functional docetaxel nanomicelles could significantly increase the survival span of brain glioma-bearing mice as compared to other control formulations.
To evaluate safety of the nanomicelles on brain glioma-bearing mice, major organs were isolated from sacrificed mice after treatments. The organs were made into paraffin sections and stained with H&E staining (). The results showed that the functional docetaxel nanomicelles did not cause significant damage to the organs, including hearts, livers, spleens, lungs and kidneys.
Discussions
Chemotherapy for brain glioma still faces challenging difficulty due to the low penetration of most anticancer drugs into the inside of the BBB [Citation17]. In fact, many chemotherapeutic drugs that are effective to cancers are unable to treat brain glioma due to restriction of the BBB [Citation18]. To solve this problem, functional nanomedicines have drawn much attention in the latest years [Citation19,Citation20]. Our previous investigations have demonstrated that the functionalized nanocarriers are able to enhance the efficacies of anticancer drugs in treatment of brain glioma [Citation21–23].
In this study, we report an easy formulation of functional nanomicelles to reach such purpose by assembling soluplus, glucose-lipid derivative and dequalinium-lipid derivative. Soluplus has a polyethylene glycol backbone as the hydrophilic part and several vinylcaprolactam/vinyl acetate side chains as the hydrophobic part. In the formulation, docetaxel is entrapped into the hydrophobic core of soluplus according to hydrophilic-lipophilic characteristics while two targeting conjugates are incorporated into the nanomicelles to play the dual targeting functions ().
For the nanomicelle assembly, a low critical micelle concentration (CMC) is critical to the stability during the blood circulation after drug administration [Citation24–26]. The present study indicates that the functional docetaxel nanomicelles are stable during drug administration because the nanomicelle material soluplus has a low-CMC value of 7.6 mg·L−1 [Citation27,Citation28].
The constructed functional docetaxel nanomicelles are round shape with approximately 100 nm particle size. Such a particle size would be beneficial for the enhanced permeability and retention (EPR) effect ( and ). Albeit the EPR effect in the BBB could be weaker than that in solid tumour tissues, the nanomicelles could transport across the BBB by the enhanced permeability. This is because the permeability of the BBB would develop with advanced stages of brain glioma [Citation29].
In fact, the transport ability of the functional docetaxel nanomicelles is mainly associated with the transfer mediated by the glucose transporter. This hypothesis is proved by the results from the transport study in which GLU modified docetaxel nanomicelles demonstrate an improved transport across the BBB (). The mechanism could be explained by the fact that GLUT is a membrane protein, which facilitates the transport of glucose over a membrane such as the BBB. Accordingly, GLUT protein transports the glucose derivative-modified nanomicelles toward the inside of the BBB. Binding of glucose derivative to one site induces a conformational change associated with transport, and releases glucose derivative to the other side of the BBB. In addition, the moiety of TPGS1000 on the functional docetaxel nanomicelles also contributes to the transport ability across the BBB. This is because the BBB overexpresses glycoprotein while TPGS1000 is a glycoprotein inhibitor [Citation30], which blocks the efflux of the nanomicelles.
The enhanced cellular uptake of functional docetaxel nanomicelles is helpful to treat brain glioma. The results from both flow cytometry and confocal microscopy indicate that the functional coumarin nanomicelles are the most internalized by the brain glioma cells (). The improved cellular uptake by brain glioma is also associated with the modification of glucose derivative on the functional nanomicelles because the brain glioma cells highly express the glucose transporters. Moreover, the modification of DQA on the functional nanomicelles assists to the enhanced cellular uptake because the negatively charged glioma cells are easy to capture the cationic DQA modified nanomicelles.
The robust efficacy of the functional docetaxel nanomicelles can be demonstrated by the cytotoxic effect to the brain glioma cells (), and to brain glioma spheroids (). These results reveal that the functional docetaxel nanomicelles have the strongest killing effect to brain glioma cells and multicellular glioma spheroids as compared to the control formulations.
The enhanced efficacy is also related to the induction of apoptosis in the brain glioma cells (). The induced apoptosis by functional docetaxel nanomicelles is associated with a cascade of activations in apoptotic enzymes, from the initiator caspase 8 and 9 to effector caspase 3 (). In contrast to the control formulations, the enhanced apoptosis by the functional docetaxel nanomicelles could be attributed to the role of the modification of DQA. This is because DQA is mitochondrial targeting molecule [Citation31]. The targeting action can be seen from the results of co-localization with mitochondria of the brain glioma cells (). As the cationic charge centre of the DQA promotes the accumulation of the functional docetaxel nanomicelles into mitochondria of brain glioma cells by electrostatic interaction.
The in vivo imaging can directly observe the distribution status of the functional nanomicelles. In contrast to the control formulations, the fluorescent probe-labelled functional nanomicelles accumulated more into the brain tissues (), displaying a brain glioma targeting effect in animals. The overall efficacy is verified in brain glioma-bearing nude mice (), demonstrating that the functional docetaxel nanomicelles are able to extend survival span as compared to control groups. As a preliminary evaluation, H&E staining on the major organs reveals a safe treatment with the functional docetaxel nanomicelles ().
Conclusions
The present study develops an easy formulation of functional docetaxel nanomicelles, which could transport across the BBB, enhance the cellular uptake, target to the mitochondria, induce the apoptosis, increase the cytotoxicity in brain glioma cells and extend survival span of the brain glioma-bearing mice. The action mechanisms are associated with dual-modifications of TPGS1000-GLU and DSPE-PEG2000-DQA, both of which are beneficial for the transport across the BBB. In addition, the modification with DSPE-PEG2000-DQA helps target to the brain glioma cells and to the mitochondria. In conclusion, the functional docetaxel nanomicelles would be a promising formulation for treatment of brain glioma, deserving further development for clinical trials.
Disclosure statement
The authors report no conflicts of interest in this work.
Additional information
Funding
References
- Phillips HS, Kharbanda S, Chen R, et al. Molecular subclasses of high-grade glioma predict prognosis, delineate a pattern of disease progression, and resemble stages in neurogenesis. Cancer Cell. 2006;9:157–173.
- Parney IF, Chang SM. Current chemotherapy for glioblastoma. Cancer J. 2003;9:149–156.
- Gottesman MM, Fojo T, Bates SE. Multidrug resistance in cancer: role of ATP-dependent transporters. Nat Rev Cancer. 2002;2:48–58.
- Cordon-Cardo C, O'Brien JP, Casals D, et al. Multidrug-resistance gene (P-glycoprotein) is expressed by endothelial cells at blood-brain barrier sites. Proc Natl Acad Sci USA. 1989;86:695–698.
- Hawkins BT, Davis TP. The blood-brain barrier/neurovascular unit in health and disease. Pharmacol Rev. 2005;57:173–185.
- Papademetriou IT, Porter T. Promising approaches to circumvent the blood-brain barrier: progress, pitfalls and clinical prospects in brain cancer. Ther Deliv. 2015;6:989–1016.
- Bochmann ES, Neumann D, Gryczke A, et al. Micro-scale prediction method for API-solubility in polymeric matrices and process model for forming amorphous solid dispersion by hot-melt extrusion. Eur J Pharm Biopharm. 2016;107:40–48.
- Gabathuler R. Approaches to transport therapeutic drugs across the blood-brain barrier to treat brain diseases. Neurobiol Dis. 2010;37:48–57.
- Devraj K, Klinger ME, Myers RL, et al. GLUT-1 glucose transporters in the blood-brain barrier: differential phosphorylation. J Neurosci Res. 2011;89:1913–1925.
- Shah KK, Boreddy PR, Abbruscato TJ. Nicotine pre-exposure reduces stroke-induced glucose transporter-1 activity at the blood-brain barrier in mice. Fluids Barriers CNS. 2015;12:10.
- Rumsey SC, Kwon O, Xu GW, et al. Glucose transporter isoforms GLUT1 and GLUT3 transport dehydroascorbic acid. J Biol Chem. 1997;272:18982–18989.
- Wang XX, Li YB, Yao HJ, et al. The use of mitochondrial targeting resveratrol liposomes modified with a dequalinium polyethylene glycol-distearoylphosphatidyl ethanolamine conjugate to induce apoptosis in resistant lung cancer cells. Biomaterials. 2011;32:5673–5687.
- Li N, Zhang CX, Wang XX, et al. Development of targeting lonidamine liposomes that circumvent drug-resistant cancer by acting on mitochondrial signaling pathways. Biomaterials. 2013;34:3366–3380.
- Mu LM, Wu JS, Xie HJ, et al. The use of a new functional glucose conjugate material, TPGS1000-Glu, in treatment of brain glioma by incorporating into epirubicin liposomes. J Chin Pharm Sci. 2016;25: 266–274.
- Ju RJ, Zeng F, Liu L, et al. Destruction of vasculogenic mimicry channels by targeting epirubicin plus celecoxib liposomes in treatment of brain glioma. Int J Nanomed. 2016;11:1131–1146.
- Ju RJ, Cheng L, Peng XM, et al. Octreotide-modified liposomes containing daunorubicin and dihydroartemisinin for treatment of invasive breast cancer. Artif Cells Nanomed Biotechnol 2018. [13 p.]. doi: https://doi.org/10.1080/21691401.2018.1433187.
- Dolecek TA, Propp JM, Stroup NE, et al. CBTRUS statistical report: primary brain and central nervous system tumors diagnosed in the United States in 2005–2009. Neuro-Oncol. 2012;14(Suppl 5):v1–49.
- Zou D, Wang W, Lei D, et al. Penetration of blood-brain barrier and antitumor activity and nerve repair in glioma by doxorubicin-loaded monosialoganglioside micelles system. Int J Nanomed. 2017;12:4879–4889.
- Xue J, Zhao Z, Zhang L, et al. Neutrophil-mediated anticancer drug delivery for suppression of postoperative malignant glioma recurrence. Nat Nanotechnol. 2017;12:692–700.
- Kreuter J. Drug delivery to the central nervous system by polymeric nanoparticles: what do we know? Adv Drug Deliv Rev. 2014;71:2–14.
- Ying X, Wen H, Lu WL, et al. Dual-targeting daunorubicin liposomes improve the therapeutic efficacy of brain glioma in animals. J Control Release. 2010;141:183–192.
- Li XY, Zhao Y, Sun MG, et al. Multifunctional liposomes loaded with paclitaxel and artemether for treatment of invasive brain glioma. Biomaterials. 2014;35:5591–5604.
- Liu S, Zhang SM, Ju RJ, et al. Antitumor efficacy of Lf modified daunorubicin plus honokiol liposomes in treatment of brain glioma. Eur J Pharm Sci. 2017;106:185–197.
- Chiappetta DA, Hocht C, Taira C, et al. Oral pharmacokinetics of the anti-HIV efavirenz encapsulated within polymeric micelles. Biomaterials. 2011;32:2379–2387.
- Chiappetta DA, Sosnik A. Poly(ethylene oxide)-poly(propylene oxide) block copolymer micelles as drug delivery agents: improved hydrosolubility, stability and bioavailability of drugs. Eur J Pharm Biopharm. 2007;66:303–317.
- Moretton MA, Cohen L, Lepera L, et al. Enhanced oral bioavailability of nevirapine within micellar nanocarriers compared with Viramune(R). Colloid Surface B. 2014;122:56–65.
- Homayouni A, Sadeghi F, Nokhodchi A, et al. Preparation and characterization of celecoxib dispersions in soluplus(®): comparison of spray drying and conventional methods. Iran J Pharm Res. 2015;14:35–50.
- Soulairol I, Tarlier N, Bataille B, et al. Spray-dried solid dispersions of nifedipine and vinylcaprolactam/vinylacetate/PEG6000 for compacted oral formulations. Int J Pharm. 2015;481:140–147.
- Wohlfart S, Khalansky AS, Gelperina S, et al. Efficient chemotherapy of rat glioblastoma using doxorubicin-loaded PLGA nanoparticles with different stabilizers. PLoS One. 2011;6:e19121.
- Wang AT, Liang DS, Liu YJ, et al. Roles of ligand and TPGS of micelles in regulating internalization, penetration and accumulation against sensitive or resistant tumor and therapy for multidrug resistant tumors. Biomaterials. 2015;53:160–172.
- D’Souza GG, Boddapati SV, Weissig V. Mitochondrial leader sequence–plasmid DNA conjugates delivered into mammalian cells by DQAsomes co-localize with mitochondria. Mitochondrion. 2005;5:352–358.