Abstract
During the development of artificial red blood cell (RBC) substitutes, oxidation side reaction is one of the major factors that hinder the application of haemoglobin (Hb)-based oxygen carriers (HBOCs). In order to avoid oxidation toxicity, we designed and prepared natural Hb conjugated with ferulic acid (FA) via simple chemical modification. In addition, the thiol groups on Hb surface were increased via the reaction of Hb with 2-iminothiolane (2-IT) and then modified with FA for the study of anti-oxidant ability. It was showed that Hb modified with FA (FA-Hb) had similar oxygen-binding capacity to natural Hb. Moreover, the anti-oxidant ability of FA-Hb in vitro in different systems was superior to natural Hb and in proportion to the degree of modification of FA. The results indicate that FA-Hb might have the potential to serve as a novel oxygen carrier with the capacity to reduce oxidative side reaction.
Introduction
In surgical operations, nature disasters, and battlefield wounds, blood transfusion, which is clinically significant, is widely applied for saving lives and maintaining the normal physiologic functions of the organisms. However, there are some inevitable risks of donated human red blood cell (RBC), such as severe shortage, short shelf life, cross matching test, and virus transmission [Citation1–3]. These intrinsic limitations aggravate the severe shortage of quality assured, donated human RBC units for eligible transfusion. As such, the development of suitable blood substitutes persists as one of the foremost important challenges in biomedicine.
Artificial blood substitutes aim to overcome the aforementioned drawbacks of collecting, storing, testing and trans-fusing donated human RBC units, and to provide immediate and safe oxygen delivery for a variety of clinical applications. Most well-developed artificial blood substitutes are “RBC substitutes” or, in another word, oxygen carriers which have several common features: (i) they are fully synthetic products or are derived from mammalian blood with long shelf-lives and from abundant source material; (ii) they do not require blood type matching before transfusion; (iii) they can be easily pasteurized to eliminate microbial or viral infection; and (iv) they can bind oxygen with high affinity and enable rapid tissue delivery. As an appropriate substitute of RBC, haemoglobin-based oxygen carriers (HBOCs) have been developed for transfusion medicine in recent years [Citation4,Citation5]. HBOCs are generally classified into two types: One is the acellular type. Based on different conjugation chemistries, three types of acellular HBOC have been developed and forwarded to human clinical trials, including cross-linked, polymerized, and conjugated (i.e. surface-modified) Hbs [Citation6–9]. Another kind is the cellular-type systems. Although they are not yet in clinical trials, they have been shown to have excellent O2 carrying ability and good safety profiles in vivo [Citation10–13]. The usefulness and safety of HBOCs have been demonstrated by many researchers in various fields such as biochemistry, physiology and toxicology [Citation14,Citation15].
Acellular type HBOCs possess the ability to transport and transfer oxygen as to re-establish oxygen homeostasis in tissues. Nevertheless, because of the elimination of constitutive enzymes and reductants (e.g. catalase, glutathione) necessary for methaemoglobin (MetHb) reduction during the Hb purification process [Citation16], they are prone to undergo autoxidation, followed by production of reactive oxygen species (ROS) and nonfunctional MetHb, The superoxide ion, a product from the autoxidation of Hb, subsequently dismutates to H2O2 and leads to the oxidative modification of Hb [Citation17]. The MetHb undergoes the heme loss and precipitation, giving rise to Heinz body [Citation18]. H2O2 may lead to irreversible cell damage and accelerate the autoxidation of Hb [Citation19]. Thus, the autoxidation of Hb has received considerable attention, especially in the development of HBOCs.
Improving the anti-oxidant capacity of HBOCs has been one of the most important research directions in blood substitutes. Chang et al. reported that a strategy has been developed to minimize the above-mentioned side reactions based on cross-linking Hb with superoxide dismutase (SOD) and catalase (CAT) extracted from RBCs [Citation20,Citation21]. Alagic et al. also designed novel chemical reagents to cross-link and connect Hb with SOD [Citation22], which showed the activity of SOD as well as lower oxygenation cooperativity of Hb. Furthermore, some other methods to enhance the anti-oxidant ability of HBOCs by chemical modification have also been reported in the literatures [Citation23–25].
Ferulic acid (FA) is a phytochemical substance commonly found in fruits and vegetables such as rice bran, sweet corn and tomatoes, which exhibits a wide range of therapeutic effects against various diseases like diabetes, cardiovascular disease and cancer. A wide spectrum of beneficial activity for human health has been advocated for this phenolic compound, at least in part, because of its strong antioxidant activity. FA is an effective scavenger of free radicals and it has been approved in certain countries as food additive to prevent lipid peroxidation. It possesses antioxidant property by virtue of its phenolic hydroxyl group in its structure, hence effectively scavenging superoxide anion radical and quenching the free radicals [Citation26,Citation27]. With these characteristics, FA can be a promising Hb modifier. However, there are few reports of the modification of Hb with FA in the literature. In this work, we focused on the desirable properties of the Hb modified with FA via a simple chemical process. Natural Hb was reacted with 2-iminothiolane (2-IT) in order that more amino groups could be converted to thiol groups, then the chemical modifier, bromoacetylethyleneglycolferulate (BAEGF) () [Citation28], was conjugated on Hb to form FA-Hb, thereby incorporating the ROS elimination system. The UV-visible spectral properties of FA-Hb were characterized, and the modification degrees of FA as well as the oxygen-carrying capacities were also evaluated. Subsequently, the anti-oxidant activities of FA-Hb in different systems were investigated to confirm as potential oxygen carriers with less oxidative side reactions.
Experimental
Reagents and instruments
Human umbilical cord blood was a kind gift from Tianjin Stem Cell Gene Engineering Company (Tianjin, China). (Z)-ferulic acid, bromoacetic acid, ethylene glycol, 2-iminothiolane (2-IT), ascorbic acid (Vc), xanthine, xanthine oxidase and other chemical reagents used were of analytical grade from Aladdin Reagent Co. Ltd. (Shanghai, China). The solutions were prepared with deionized water. UV-visible spectrophotometry analysis was conducted using SHIMADZU UV-2550 (Kyoto, Japan), and the ultrafiltration membrane was used with 10 kDa ultrafiltration centrifugal tubes (Nanosep, Omega™, Beijing, China). The 1 H NMR test was performed by NanoBay 400 MHz (AvanceTMIIIHD, Bruker, Karlsruhe, Germany); the oxygen affinity was measured on a hemoxanalyzer (TCS Scientific Corporation, New Hope, PA, USA).
Methods
Purification of Hb from red blood cells
Preparation of purified Hb from human placenta referred to the method described by Walder et al. [Citation29]. The final product in the retentate was collected and stored at −80 °C.
Synthesis of BAEGF
BAEGF was synthesized as modifier according to the method described previously [Citation28].
Direct modification of BAEGF to Hb
Ten millilitres of 1 mM stroma-free Hb solution was in vacuum for 30 min to deoxygenate and then was sealed. Seventy milligrams of BAEGF was dissolved in 500 μL DMSO, which then was mixed with 500 μL of 10 mM Vc solution. The mixture was injected into the stroma-free Hb solution and the reaction was carried out at 4 °C for 5 h with gentle stirring under N2. The FA-Hb product was purified as the retentate after 10 kDa ultrafiltration centrifugal tube (Nanosep, Omega™) at 4000 r/min for 15 min at 4 °C, and this step was repeated three times. The final product (FA-Hb) was exchanged into 2 mM PBS (pH = 7.4).
Conversion of amino groups of Hb to thiol groups with 2-IT and modification by BAEGF
shows the conversion of amino to thiol groups on Hb surface and the modification of BAEGF. The stroma-free Hb was diluted to 1 mM by deionized water. Ten millilitres of Hb solution was added Vc 10 times the mole ratio of Hb, and then reacted with 2-IT (five times the mole ratio of Hb). The reaction was carried out in vacuum at 4 °C for 3 h and was protected with N2. Then a sample was taken out for thiol group determination, using a 4, 4′-dithiodipyridine (4, 4′-PDS) analysis to determine the extent of accessible thiol groups [Citation30]. Afterwards, BAEGF, the dosage of which was identical with 2-IT, was added into the solution and the mixture was reacted for another 3 h under the same conditions. After the reaction was over, the product was purified as the retentate after 10 kDa ultrafiltration centrifugal tube (Nanosep, Omega™) at 4000 r/min for 15 min at 4 °C, and this operation was repeated thrice. The final product (FA-Hb) was exchanged into 2 mM PBS (pH = 7.4) and diluted to the volume before centrifugation. The residual thiol groups and modification degree of BAEGF were determined according to the method described previously [Citation28]. Besides, parallel experiments were also carried out, using different amounts of 2-IT to increase the number of thiol groups of Hb, and then modified with BAEGF. 2-IT dosage was selected 10, 15 and 20 times more than Hb in mole ratio, and the subsequent amounts of BAEGF were equal to that of 2-IT, respectively. Other reaction conditions were as mentioned above.
Study of the structure and the oxygen affinity of FA-Hb
UV-vis full wavelength scanning of the FA-Hb solution (0.1 g/L) was recorded in 300 nm to 800 nm range. Oxygen dissociation curve of FA-Hb (6.0 g/L, direct modification with FA without conversion of amino groups of Hb to thiol groups) was measured using a Hemoxanalyzer at 37 °C in PBS, pH = 7.4. Triplicate measurements for each sample were performed to obtain the average value of P50, which was obtained from the oxygen dissociation curves.
Autoxidation experiments of Hb/FA-Hb
Autoxidation experiments for Hb/FA-Hb samples (25 μM) in PBS buffer, pH = 7.4, containing 0.1 mM EDTA at 37 °C were carried out in the dark. Absorbance changes in the range of 500–700 nm due to the spontaneous oxidation of Hbs were recorded on UV-vis spectrometer at 0.5 h intervals. The whole experimental time was 3 h. The blank assays were carried out under the same conditions without Hb/FA-Hb. The time-dependent changes in concentration of the MetHb were calculated based on the method described by Abugo et al. [Citation31].
Autoxidation experiments of Hb/FA-Hb with xanthine–xanthine oxidase
The Hb/FA-Hb solution was diluted to the concentration of 0.1 mM with deionized water, containing 50 mM potassium phosphate, 1 mM EDTA and 0.5 mM xanthine, pH = 7.3. 50 ml of the aforementioned Hb solution was mixed with15μL xanthine oxidase in sealed tube at 37 °C. The mixture was sampled by UV-vis spectrometer in the range of 500–700 nm at 5 min intervals for 1 h. The blank assays were carried out under the same conditions without Hb/FA-Hb. The time-dependent changes in concentration of the MetHb were calculated based on the above-mentioned method [Citation31].
Results
Standard curve of BAEGF
shows the excellent correlation between the absorbance at 327 nm and the concentration of BAEGF. This is in good accordance with Lambert–Beer law in dilute solution, which can act as the basis to calculate the modification degree of BAEGF on Hb.
Characteristics of Hb and FA-Hb by UV-vis
The UV-vis spectra of Hb and FA-Hb (without reaction with 2-IT) were shown in . For the curve of Hb, there were three typical peaks of Hb at 410 nm, 541 nm and 577 nm, respectively. For the curve of FA-Hb, there was no shift and shape change of the aforementioned three peaks, indicating that the spatial conformation of Hb did not change after modification. These results suggested that FA-Hb had a good oxygen carrying ability similar to Hb. Furthermore, there appeared a new absorption peak at 327 nm on the UV-vis curve of FA-Hb, which is the characteristic peak of FA, but at the same position in the UV-vis spectrum of Hb there is no such peak. The change of UV-vis absorption peak at 327 nm indicates that BAEGF was successfully modified onto Hb.
Modification degree of FA to Hb after increasing the thiol groups on Hb by 2-IT
Different proportions of 2-IT were reacted with Hb to increase the thiol group on the surface of Hb, and then different proportions of BAEGF were used to react with Hb in order to increase the modification degree of FA. The results of the reaction are listed in . It can be seen from the results that the number of thiol groups on Hb surface increased after modification of Hb with 2-IT, so did FA. The degree of modification of FA was proportional to the dosage of 2-IT and BAEGF. After BAEGF modification, the number of thiol groups on Hb decreased to almost 0. The results showed that BAEGF reacted completely with thiol groups on Hb and all the latter were blocked.
Table 1. Modification degree of FA after reaction of Hb with various dosage of 2-IT and BAEGF.
Oxygen affinity of Hb and FA-Hb
The oxygen affinity (P50) of Hb and FA-Hb are shown in , respectively. In MetHb, one or more Fe3+ ions cannot bind to oxygen. The affinity between MetHb and oxygen increases so that the oxygen dissociation curve moves to the left with the reduced value of P50, which could result in functional anaemia and tissue anoxia. After the modification of BAEGF, the P50 value of Hb becomes a little higher (13.04 mmHg) than that before modification (10.88 mmHg), which may be owed to the coordination effect of BAEGF on oxygen release. The consistency in P50value of FA-Hb is more significant than that of Hb.
Autoxidation study of Hb and FA-Hb
shows the time course of MetHb formation during the autoxidation of Hb and FA-Hb. In the case of Hb or FA-Hb without 2-IT modification (), there is a gradually time-dependent elevation in the MetHb percentage, and the MetHb percentage of FA-Hb increases more slowly than that of Hb. At the end of the experimental time (3 h), the MetHb percentages of Hb and FA-Hb are 40% and 30%, respectively. Moreover, the number of thiol groups on Hb surface also increased after the reaction of 2-IT with Hb, followed by the increase of modification degree of FA on Hb in the next step. It can be seen from the that with the increasing modification degree of FA, the autoxidation rate of FA-Hb decreases distinctly, In 3 h, the MetHb content of FA-Hb was 29.7% after the reaction of 5-fold 2-IT (mole ratio) with Hb, while the amount of 2-IT was 20 times higher than that of Hb, the MetHb level of FA-Hb was only 23.5% at the same time. The results of autoxidation experiment show that the modification of FA can benefit the anti-oxidant ability of Hb.
Figure 6. Time course of MetHb formation in Hb and various FA-Hb at 37 °C. (A) Hb; (B) FA-Hb (without 2-IT modification); (C) FA-Hb (2-IT:BAEGF:Hb = 5:5:1); (D) FA-Hb(2-IT:BAEGF:Hb =10:10:1); (E) FA-Hb (2-IT:BAEGF:Hb =15:15:1); (F) FA-Hb (2-IT:BAEGF:Hb =20:20:1); (G) the summary and comparison of autoxidation rate from (A)–(F). The inner charts of (A)–(F) represent the UV-vis spectrum of the corresponding product, respectively.
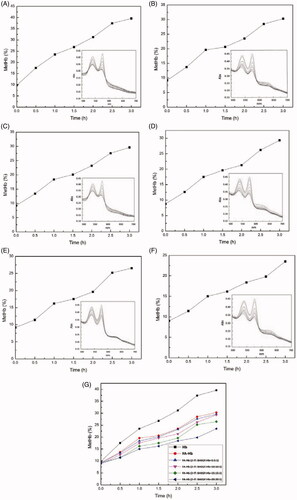
Autoxidation study of Hb and FA-Hbwithxanthine-xanthine oxidase
shows the time course of MetHb formation during the autoxidation of Hb and FA-Hb in the presence of xanthine–xanthine oxidase. Compared with the autoxidation course without xanthine–xanthine oxidase, the oxidation rate of Hb and FA-Hb is accelerated under the action of xanthine–xanthine oxidase system. The MetHb content in Hb increased from the initial 6% to about 80% in just 1 h (). In contrast, due to the anti-oxidation ability of FA, the MetHb content in FA-Hb (without 2-IT modification) is about 73% in 1 h, which is lower than that of Hb (). Furthermore, the content of MetHb decreased with the increase of FA modification. For example, in 1 h, the MetHb content of FA-Hb was 69.1% after the reaction of 10-fold 2-IT (mole ratio) with Hb (). When the mole ratio changed to 20:1, the MetHb level of FA-Hb was 61% at the same time (). The experimental results showed that in the presence of xanthine–xanthine oxidase, FA-Hb showed better anti-oxidant activity than unmodified Hb. The higher the modification degree of FA was, the lower the MetHb content became.
Figure 7. Time course of MetHb formation in Hb and various FA-Hb at 37 °C in the presence of xanthine–xanthine oxidase. (A) Hb; (B) FA-Hb (without 2-IT modification); (C) FA-Hb (2-IT:BAEGF:Hb = 5:5:1); (D) FA-Hb (2-IT:BAEGF:Hb =10:10:1); (E) FA-Hb (2-IT:BAEGF:Hb =15:15:1); (F) FA-Hb (2-IT:BAEGF:Hb =20:20:1); (G) the summary and comparison of autoxidation rate from (A)–(F). The inner charts of (A)–(F) represent the UV-vis spectrum of the corresponding product, respectively.
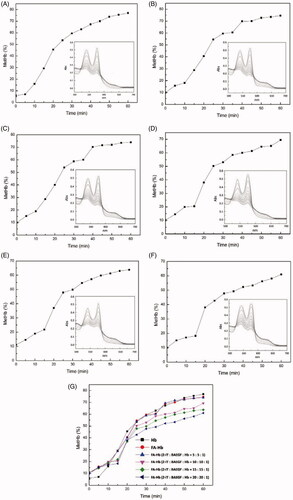
Discussion
Haemoglobin possesses the ability to transport and transfer oxygen as blood substitute to re-establish oxygen homeostasis in tissues. Nevertheless, unmodified Hb is not ideal oxygen carriers because of two main problems: one is the dissociation into dimers resulting in renal toxicity and a short circulation; the other is prone to cause oxidative stress injury. During severe injury and shock, the increase of intracellular H2O2 in the body accounts for the excessive generation of free radicals. Thus, HBOCs with free radical scavenging ability are still of great significance in practical application.
Nowadays, various HBOCs have been developed for clinical application as RBC substitutes such as cross-linking [Citation32], surface modification [Citation33] and encapsulation [Citation34]. Unfortunately, serious adverse effects still occurred in phase II and phase III clinical trials [Citation35]. In any case, HBOCs should have good oxygen delivery capacity and good anti-oxidation ability, hence the requirement of appropriate chemical modification of Hb to decrease the content of MetHb. As far as the method itself is concerned, it is an effective approach to improve the anti-oxidant ability of HBOC by combining free radical scavengers with Hb.
Ferulic acid is a well-known stable and available reagent, which has shown good performance on quenching ROS. To some extent, FA can simulate the function of SOD and CAT so that it is expected to produce the anti-oxidation effect on HBOCs spontaneously, and reduce toxicity [Citation36]. In this study, we first synthesized the modifier BAEGF containing FA structure and then it was reacted with Hb to form FA-Hb, thereby incorporating the MetHb elimination system into the Hbs themselves. In addition, by increasing the thiol groups on Hb surface with 2-IT, we also modified Hb with more FA structures. Meanwhile, we evaluated the oxygen affinity of FA-Hb and the effect of conjugation on the suppression of MetHb formation.
In contrast with natural Hb, the UV-vis wavelength scanning showed that there was a successful modification of FA on Hb and no change in the peak position and absorption intensity of Hb, which demonstrated the maintenance in the spatial conformation around heme in FA-Hb. Therefore, the modification had no effects on the function of carrying O2. In addition, the oxygen dissociation curve showed a similar oxygen affinity between Hb and FA-Hb, indicating a good oxygen-carrying ability of FA-Hb.
When BAEGF was conjugated on Hb, the modification of bromoacetyl in BAEGF was preferentially produced on β-Cys93 of Hb.Cys93, which is located on β-chain surface, participated in the oxygen free radical reaction and accelerated the production of MetHb. The site-specifically modification of FA on β-Cys93effectively blocked the β-chain heme’s oxidation, thereby reducing the production of free radicals [Citation37]. Therefore, the content of MetHb was decreased after the modification of FA, compared with natural Hb. After the reaction of 2-IT with Hb, the number of thiol groups on Hb increased and the amount of BAEGF modified on Hb increased thereupon. From the results of Hb autoxidation experiment, it can be seen that the more FA structures are connected to Hb, the lower the content of MetHb produced during autoxidation. The above experimental results showed that the anti-oxidant ability of Hb was further improved and proportional to the amounts of FA.
Under the catalysis of xanthine oxidase, free oxygen molecules were reacted with xanthines to generate superoxide anion radical (O2−), which was prone to combine with H+ and then was further converted into H2O2. H2O2 could rapidly oxidize the deoxyHb to MetHb eventually, hence the acceleration of autoxidation of Hb. Therefore, we determined the autoxidation rates of Hb and FA-Hb in the existence of xanthine-xanthine oxidase. It was found that although the autoxidation rate of both Hb and FA-Hb became rapid than that without xanthine–xanthine oxidase, the MetHb content of FA-Hb was still lower when compared with Hb in the same period of time. Similar to the autoxidation process without xanthine–xanthine oxidase, the content of MetHb decreased with the increase of FA structures. These results showed the enhanced anti-oxidation ability of FA-Hb under different conditions and demonstrated that FA-Hb possessed a good free radical scavenging function and could effectively improve the antioxidant ability of HBOC.
Conclusions
In this paper, we have presented FA-Hb, a novel HBOC with oxygen-carrying and anti-oxidant activities. FA-Hb could not only maintain the integrity of Hb chemical structure, but show an oxygen affinity similar to natural Hb as well. In addition, the oxidative action of Hb to MetHb was decreased after the modification of FA. As the degree of FA modification increased, the anti-oxidant capacity of Hb was also enhanced gradually. Excitingly, FA-Hb exhibited excellent anti-oxidant activity under different experimental conditions. These excellent characteristics suggest that FA-Hb may become a promising oxygen carrier.
Acknowledgements
We appreciate Professor Liu Jiaxin and Wang Hong, for helpful discussions and Li Shen for communicating unpublished results. This report would not have been possible without the support and substantive contributions of the Senior Research Scientists at Institute of Blood Transfusion, Chinese Academy of Medical Sciences, Chengdu, P. R. China.
Disclosure statement
The authors report no declarations of interest. The authors alone are responsible for the content and writing of the paper.
Additional information
Funding
References
- Seifried E, Mueller MM. The present and future of transfusion medicine. Blood Transfus. 2011;9:371–376.
- Modery-Pawlowski CL, Tian LL, Pan V, et al. Synthetic approaches to RBC mimicry and oxygen carrier systems. Biomacromolecules. 2013;14:939–948.
- Chang TMS. From artificial red blood cells, oxygen carriers, and oxygen therapeutics to artificial cells, nanomedicine, and beyond. Artif Cells Blood Substit Immobil Biotechnol. 2012;40:197–199.
- Winslow RM. Progress on blood substitutes. Nat Med. 1997;3:474.
- Chang TMS. Blood substitutes: principles, methods, products and clinical trials. Switzerland: S. Karger AG; 1997.
- Gould SA, Moore EE, Hoyt DB, et al. The first randomized trial of human polymerized hemoglobin as a blood substitute in acute trauma and emergency surgery. J Am Coll Surg. 1998;187:113–122.
- McCarthy MR, Vandegriff KD, Winslow RM. The role of facilitated diffusion in oxygen transport by cell-free hemoglobins: implications for the design of hemoglobin-based oxygen carriers. Biophys Chem. 2001;92:103–117.
- Sloan EP, Koenigsberg M, Gens D, et al. Diaspirin cross-linked hemoglobin (DCLHb) in the treatment of severe traumatic hemorrhagic shock: a randomized controlled efficacy trial. JAMA. 1999;282:1857–1864.
- Mullon J, Giacoppe G, Clagett C, et al. Transfusions of polymerized bovine hemoglobin in a patient with severe autoimmune hemolytic anemia. N Engl J Med. 2000;342:1638–1643.
- Sakai H, Cabrales P, Tsai AG, et al. Oxygen release from low and normal P50Hb vesicles in transiently occluded arterioles of the hamster window model. Am J Physiol. 2005;288:H2897–H2903.
- Cabrales P, Sakai H, Tsai AG, et al. Oxygen transport by low and normal oxygen affinity hemoglobin vesicles in extreme hemodilution. Am J Physiol. 2005;288:H1885–H1892.
- Jia Y, Duan L, Li J. Hemoglobin-based nanoarchitectonic assemblies as oxygen carriers. Adv Mater Weinheim. 2016;28:1312–1318.
- Hosaka H, Haruki R, Yamada K, et al. Hemoglobin-albumin cluster incorporating a Pt nanoparticle: artificial O2 carrier with anti-oxidant activities. PLoS One. 2014;9:e110541.
- Reiss JG. Oxygen carriers (“blood substitutes”)-raisond’etre, chemistry, and some physiology. Chem Rev. 2001;101:2797–2920.
- D’Agnillo F, Alayash AI. Redox cycling of diaspirin cross-liked Hb induced G2/M arrest and apoptosis in cultured endothelial cells. Blood. 2001;98:3315–3323.
- Naito Y, Fukutomi I, Masada Y, et al. Virus removal from hemoglobin solution using Planova membrane. Artif Organs. 2002; 5:141–145.
- Nagababu E, Ramasamy S, Rifkind JM, et al. Site-specific cross-linking of human and bovine hemoglobins differentially alters oxygen binding and redox side reactions producing rhombic heme and heme degradation. Biochemistry. 2002;41:7407–7415.
- Winterbourn CC. Oxidative reactions of hemoglobin. Meth Enzymol. 1990;186:265–272.
- Johnson RM, Goyette G, Jr, Ravindranath Y, et al. Hemoglobin autoxidation and regulation of endogenous H2O2 levels in erythrocytes. Free Radic Biol Med. 2005;39:1407–1417.
- Wang MY, Yu YT, Chang TMS. New method for preparing more stable microcapsules for the entrapment of genetically engineered cells. Artif Cells Blood Subs Immob Biotech. 2005;33:257–269.
- Gu J, Chang TMS. Extraction of erythrocyte enzymes for the preparation of polyhemoglobin-catalase-superoxide dismutase. Artif Cells Blood Subs and Biotech. 2009;37:69–77.
- Alagic A, Koprianiuk A, Kluger R. Hb-superoxide dismutases-chemical linkages that create a dual-function protein. J Am Chem Soc. 2005;127:8036–8043.
- Hathazi D, Mot AC, Vaida A, et al. Oxidative protection of hemoglobin and hemerythrin by cross-linking with a nonheme iron peroxidase: potentially improved oxygen carriers for use in blood substitutes. Biomacromolecules. 2014;15:1920–1927.
- Schaer CA, Deuel JW, Bittermann AG, et al. Mechanisms of haptoglobin protection against hemoglobin peroxidation triggered endothelial damage. Cell Death Differ. 2013;20:1569–1579.
- Simoni J, Simoni G, Wesson DE, et al. ATP-adenosine-glutathione cross-linked hemoglobin as clinically useful oxygen carrier. Curr Drug Discovery Technol. 2012;9:173–187.
- Ou S, Kwok KC. Ferulic acid: pharmaceutical functions, preparation and applications in foods. J Sci Food Agric. 2004;84:1261–1269.
- Mathew S, Abraham T. Ferulic acid: an anti-oxidant found naturally in plant cell walls and feruloylesterases involved in its release and their applications. Crit Rev Biotechnol. 2004;24:59–83.
- Guo S, Wang P, Chen C, et al. Processing of ferulic acid modified hemoglobin. Artif Cells Nanomed Biotechnol. 2016;44:1075–1079.
- Walder RY, Andracki ME, Walder JA. Preparation of intramolecularly cross-linked hemoglobins. Meth Enzymol. 1994;231:274–280.
- Riener CK, Kada G, Gruber HJ. Quick measurement of protein sulfhydryls with Ellman's reagent and with 4, 4'-dithiodipyridine. Anal Bioanal Chem. 2002;373:266–276.
- Abugo OO, Rifkind JM. Oxidation of hemoglobin and the enhancement produced by nitroblue tetrazolium. J Biol Chem. 1994;269:24845–24853.
- Chang TM. Stabilisation of enzymes by microencapsulation with a concentrated protein solution or by microencapsulation followed by cross-linking with glutaraldehyde. Biochem Biophys Res Commun. 1971;44:1531–1536.
- Winslow RM. Blood substitutes. Adv Drug Deliv Rev. 2000;40:131–142.
- Rameez S, Alosta H, Palmer AF. Biocompatible and biodegradable polymersome encapsulated hemoglobin: a potential oxygen carrier. Bioconjugate Chem. 2008;19:1025–1032.
- Silverman TA, Weiskopf RB. Hemoglobin-based oxygen carriers: current status and future directions. Transfusion. 2009; 49:2495–2515.
- Nicholson SK, Tucker GA, Brameld JM. Effects of dietary polyphenols on gene expression in human vascular endothelial cells. Proc Nutr Soc. 2008;67:42–47.
- Pimenova T, Pereira CP, Gehrig P, et al. Quantitative mass spectrometry defines an oxidative hotspot in hemoglobin that is specifically protected by haptoglobin. J Proteome Res. 2010;9:4061–4070.