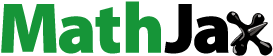
Abstract
Nanoformulations derived from fine porous ZnO quantum dot nanoparticles (QD NPs) can offer strong potential medical applications; especially in cancer therapy. ZnO QD NPs was synthesized by sol–gel hydrothermal process, fast cold quenching and further smart surface functionalization methods to obtain ultrasmall size (1–4 nm) NPs. ZnO nanopolymer, a wetting agent, PEG co-solvent and water/oil emulsion stabilizer were considered in our nanofluid formulation. The resulting nanofluid was characterized by SEM, FTIR, photoluminescence, band gap energy, zeta potential and UV–Vis spectroscopy. The cytotoxic effects on the growth of four cancer cell lines were evaluated by MTT assay. The IC50 (µg/ml) values of 30, 41, 40 and 35 for KB44, MCF-7, HT29 and HeLa cells, respectively, after 48 h of nanoformulation treatment suggested the cytotoxic effect of this nanoformulation on these cell lines in a concentration-dependent manner (p < .05). ZnO nanofluid destroyed cancer cell lines more efficiently than the normal HFF-2 (IC50 = 105 µg/ml). The reduction in cell viability in response to ZnO nanofluid treatment induced apoptosis in the cultured cells. Skin sensitization test plus antibacterial activity were also measured. Side effect tests on 70 white mice in vivo resulted in only 3–4 abnormal situations in hepatic tissue section possibly due to the idiosyncratic drug reactions.
Graphical Abstract

Introduction
Scientists have been trying to develop the best technologies and methods to improve public health in the future. Their main intention is to help sick people and reduce the length of treatment. Richard Feynman, for example developed one of the best and modern technologies in this regard so far in 1959-the knowledge of Nano. Nanotechnology and Nanobiotechnology can potentially help scientific research using distinctive properties of nanoparticles (NPs) in nanomedicine and nanopharmacology for the treatment of human diseases; especially fatal cancers. Unfortunately, conventional chemotherapeutic agents have been observed to perform poorly in tumour tissues. Because these agents have side effects, exhibit strong resistance against microbes and are mostly restricted by dose-limiting toxicity [Citation1]. Therefore, it is necessary for scientists and researchers to sufficiently compensate for these shortages and deficiencies. Accordingly, the low toxicity and biodegradability of ZnO NPs with strong positive charge surface (ZnOH2+) groups and unique zero dimension (0 D) quantum dot (QD) semiconductors with a broad range of applications could change the past landscape of pharmaceutical, biomedical and biotechnology industries [Citation2,Citation3]. They exhibit potential electrostatic interactions to promote cellular uptake through anionic phospholipids and sulphur containing compounds, such as DNA, regulate enzymes on the outer cancerous cell membrane and selectively destroy tumour cells, at least for cells of lymphocytic origin [Citation4,Citation5]. This mechanism is very vital and advantageous for biocompatible wurtzite structure of ZnO NPs, especially for appealing ZnO QD NPs candidates that include particles of (1–4) nm size, to be utilized as multi-functional nanoplatform [Citation6,Citation7]. The objective of this work is the biomedical applications of ZnO QDs (with trapping states in structure) in various fields, such as cancer diagnosis and improvement of the therapeutic potential of ZnO NPs directly related to tailoring NP size (engineering design) to reduce detrimental effects on normal body cells [Citation8]. Surface modification or functionalization of ZnO QDs are additional promising and significant strategies as these compounds are not very stable and dissolve in water, making them unfavourable for application as valuable drugs. Thus, water-soluble, hydrophilic ZnO QDs ought to be focused on in accordance with surface luminescent mechanisms [Citation9,Citation10]. Various functional clinical supplements such as polymers, liposomes, proteins, organosilanes, fatty acids (oleic acid), folic acid (FA) [Citation11], amines, inorganic acids, nanometal oxides, nanometals (Ag, Au and Fe), inorganic/organic hybrid materials, PEG (polyethylene glycol), dendrimers, chitosan encapsulated nanocarriers and organosiloxanes can be positioned on the surface of ZnO QDs and create smart surface reactive morphologies. These are the latest device and methods for tailoring hydrophilic surfaces in nanotechnology science and they are able to create what we want (energetic nature, multivalent surface groups, efficiently protected luminescent centres and individual properties) with the ability to cross the cell membranes in nano-bio interface. This research has focused on four cancer cell lines; namely mouth KB44, breast MCF7, colon HT29, and HeLa in vitro cytotoxic activity test using ZnO QD NPs in the form of ZnO nanofluid. The surface of ZnO QD NPs was modified by green fatty acid, which carbocyclic acid function lies over its surface and the nanocompound can dissolve completely in water solution (chemical capping method). The capping molecule can control the size of the particles and protect them from coagulation and aggregation in the course of fluidization. Indeed, organic fatty acid capped inorganic ZnO NPs provide new types of photophysical and photochemical properties, which lead to the organic/inorganic systems [Citation12]. Advances and challenges in therapeutic approaches of ZnO NPs were studied from several viewpoints, such as reducing systemic side effects [Citation13], anticancer treatment outcome [Citation13–15], control the release of drugs [Citation16,Citation17], toxicity and induced apoptosis [Citation18,Citation19], antimicrobial treatment results [Citation15], selectivity and efficiency in detection of cancer cells [Citation11] and histopathological changes in an animal model [Citation20]. The various morphologies of zinc oxide NPs, such as nanoplates, nanorods, nanosheets and nanoflowers at different pH values were exposed against malignant human T98G gliomas and non-malignant embryonic kidney HEK293 and MRC5 fibroblast cells as normal cells by Wahab et al. [Citation21]. Among these different morphologies, ZnO nanorods showed that they could induce higher cytotoxicity and inhibitory effects on cancer and normal cells, respectively. Here, the larger effective surface area (BET), combined with structural defects of nanorods produce hydroxyl radicals, superoxide anion, high ROS and finally H2O2 [Citation22] Therefore, it becomes obvious that type of shape, surface structure and pH play significant roles in the success of NPs against cancerous cells. The study of the effect of drug toxicity and its side effects are other important topics addressed in our research. In that connection, a sub-acute oral toxicity analysis by zinc oxide NPs anti-diabetic activity in vivo carried out by Shanker et al. is noteworthy [Citation23]. Furthermore, a study by Jacobsen et al. showed that the toxicity of zinc oxide NPs appeared to be dose dependent [Citation24].
The first aim of this novel research is to synthesize and characterize ZnO QD NPs through sol–gel, wet chemical, hydrothermal and quenching methods and to carry out surface modification via green fatty acids. Afterwards, zinc oxide/polyvinylpyrrolidone nanocomposites were fabricated as co-assistant and stabilizer for preparation and formulation of ZnO nanofluid original product providing unique chemical and physical properties. Another aim of this study was to examine the toxicity effects of ZnO nanofluid (not ZnO NPs) on four cancer cell lines in comparison with HFF-2 normal foreskin fibroblast cells after 24 and 48 h in vitro. Besides, apoptosis and necrotic induction of four cell lines were further confirmed by annexin V/PI staining. Mouse-ear swelling test (MEST) measurement was then performed for 12 d and no skin sensitization was observed in mice in vivo. Moreover, the side effects of ZnO nanofluid product were studied by exposing 70 mice in vivo. The results obtained from intraperitoneal (IP) injection toxicity and histopathological studies through mouse model showed promising results with regard to the safe and non-toxic of drug. Finally, antibacterial activity against some gram-positive bacteria was also carried out.
Methods
Synthesis and formulation of original water-based ZnO nanofluid product including three steps
Synthesis of the polar and hydrophilic surface through the modified ZnO QD NPs by green soya fatty acid: (nanoproduct 1)
ZnO QD NPs were prepared through chemical hydrolysis (sol–gel, wet chemical) and hydrothermal methods using zinc acetate dihydrate (Sigma Chemical Co., St. Louis, MO) and soya fatty acid water-alcohol solution. A mixture of hexamethylenetetramine and triethanolamine in water–ethanol solution was used for amine hydrolysis under vigorous stirring in hot (80 °C) and cold (0 °C) fast-quenching conditions in the presence of various surfactant templates to obtain polar surface area and very fine nanoparticles. The pH was then adjusted to 8 and stirring was continued under reflux for 9 h. The obtained product was placed in an oven (80 °C) for 6 d under hydrothermal process and the solvent was then completely evaporated [Citation9,Citation10,Citation25–29]. The resulting soya fatty acid-capped ZnO is highly reactive with energetic surfaces. This strategy could potentially create new ZnO nanofluid formulation as drug carriers for targeted cancer therapy.
Preparation of heterostructural zinc oxide/polyvinylpyrrolidone nanocomposites by coprecipitation and hydrothermal, quenching method: (nanoproduct 2)
For this nanoproduct, the surface of wurtzite ZnO NPs was modified by incorporation of polyvinylpyrrolidone food grade polymer (PVP) as the substrate and capping molecules. In fact, PVP acts as a co-assistant and stabilizer in the formulation of the original product. An appropriate amount of zinc acetate dihydrate (Merck Chemical Co., Darmstadt, Germany) was dissolved in a mixture of distilled water, absolute ethanol, dimethylformamide (DMF) solvent and an additive, such as polyvinylchloride (PVC) as a stabilizer and softener under vigorous magnetic stirring at 60–70 °C. Next, 3–4 g of PVP polymer was added under ultrasound conditions and mixing was continued. The hot solution was suddenly cooled down in an ice bath at 0 °C and hydrolysed by warm 30% alcoholic KOH solution for 1–2 h (pH 9–10). The reaction mixture was then refluxed at 70–80 °C for 5–7 h to complete the reaction and then, hydrothermal process was maintained at 80 °C overnight. Having evaporated the solvent, the product was washed several times with cold alcohol-water mixture and followed by drying at 85 °C in an oven [Citation9,Citation25–27,Citation30]. The energetic strong surface of ZnO NPs can thus be modified and adopted using PVP matrix substrate for biological-based applications nanoformulation. At the end of the preparation, the nanopolymer precursor was dissolved in 20 ml of mono propylene glycol solvent (MPG) (Kimyagaran Emrooz Co., Tehran, Iran) as a dispersant plus 5 ml of Span 80 (sorbitan monooleate, Merck Chemical Co., Darmstadt, Germany) as an emulsifier at room temperature. The PVP-capped ZnO NPs are more stable and have a greater potential than the non-capped ZnO NPs.
Formulation of ZnO nanofluid as the original nanoproduct
Of, 1–3 g of brownish colloidal nanoproduct 1 was dissolved in 10 ml of distilled water under magnetic stirring at 30–40 °C for 30 min. Afterwards, 2–3 g of nanoproduct 2 was dispersed into 10 ml of distilled water and then was added to nanoproduct 1 solution under vigorous stirring at 40–50 °C on a heater stirrer for 15 min. Next, 3–5 ml of non-ionic surfactant ethoxylated alcohol (from Kimyagaran Emrooz Company, Tehran, Iran) as the wetting agent was also added dropwise to the obtained hydrophilic nanofluid and mixed completely. The resulting nanoformulation was kept in an ultrasound bath at 30–40 °C for 2 h. Subsequently, 1–3 g PEG (polyethylene glycol 6000) dissolved in 20 ml of distilled water was employed as a binding agent and a very effective co-solvent to achieve smart energetic surface of nanoparticles in the formulation. Furthermore, 2–3 ml of a typical water in oil emulsifier agent, such as sorbitan monooleate (Merck Chemical Co., Darmstadt, Germany) was slowly added to the yellowish final solution in order to have homogeneous nanofluid formulation at the optimized pH value of 7–8.
Cell viability assay by MTT method
Cell lines and cell culture studies
The human nasopharynx carcinoma cell line (KB44), human breast cancer cell line (MCF-7), HeLa cell line, human colon adenocarcinoma cell line HT29 and normal human foreskin fibroblast cells (HFF-2) were purchased from the Pasteur Institute of Iran. Cells were cultured in high glucose Dulbecco’s modified Eagle’s medium (DMEM, Thermo Fisher Scientific, Waltham, MA) with 10% foetal bovine serum (FBS, Thermo Fisher Scientific, Waltham, MA) at 37 °C, 5% CO2, and 90% humidified the air in a CO2 incubator (BINDER, Tuttlingen, Germany). The supplemented medium was refilled every other day and the cells were sub-cultured to reach the optimal confluence.
Anti-proliferation assay
The rate of cell viability or percent of cytotoxicity was assessed by the MTT assay according to a protocol published previously [Citation31]. Briefly, 1 × 104 cells were seeded in 96-well plates in 100 µl supplemented DMEM the day before adding the compounds. Different ZnO nanofluid concentrations (10, 50, 100, 200 and 500 μM) were added the next day to each cell population following the refreshing of the culture medium. Then, 48 h after treatment, the MTT powder was dissolved in PBS to reach a final concentration of 5.0 mg/ml. The solution was then diluted 10 times with PBS and added to each well (100 µl of solution). Afterwards, the solution was transferred to an incubator where it was kept for 4 h and subsequently replaced with formazan. DMSO (200 µl) was then added to each chamber and mixed gently. At last, the prepared solution was kept at RT for 20 min to dissolve the formazan and the absorbance was recorded at 550 nm using an ELISA microplate reader (BioTek, Winooski, VT). The percentage of cell viability was calculated using the following formula:
In order to determine the dose of ZnO nanofluid, which could result in proliferation inhibition in 50% of cells (IC50), GraphPad Prism version 6 (GraphPad Software, Inc, San Diego, CA) software was used for each of the cell lines separately.
Statistical analysis
All experiments were conducted in triplicate and results were presented as mean ± SD.
Two-tailed t-test and ANOVA were applied to determine the differences between viability of the various cell lines treated with nanoparticles using GraphPad Prism version 5 software (GraphPad-Prism Software Inc., San Diego, CA). The obtained results with p values <.05 were considered statistically significant.
Detection of apoptosis and necrosis and morphological analysis after ZnO nanoproduct treatment
The apoptotic and necrotic cells were identified using annexin-V-FLUOS Staining Kit (Roche Diagnostic, Indianapolis, IN). In brief, the treated cells were collected after 48 h, washed with PBS and resuspended in 100 μl of binding buffer solution. Then, 2 μl of annexin V-FITC and 2 μl of PI were added to each sample. The stained cells were kept in the dark for 20–30 min at room temperature. The percentage of apoptotic/necrotic cells was obtained using flow cytometry (PAS II; Partec GmbH, Münster, Germany). For each experiment, positive and negative controls were applied to set the gating parameters. Forward scatter (FSC) and side scatter (SSC-A) plots were used to set the gates to determine the target population. At least 20,000 events were collected for each sample. Besides, the morphological changes of the treated cells were evaluated by light microscopy method. The assays were carried out three times independently.
Animal study in vivo (protocols for sensitization or toxicological test)
Mouse-ear swelling test (MEST) to measure the sensitivity of ZnO nanofluid as sensitizers nanoproduct in vivo
To know how this nanoproduct affects human tissue; especially skin and better identify potential dermal delayed contact reaction, an allergy test called MEST was carried out on laboratory animals as described by Gad et al. in 1985, 1986 and 1994 [Citation32]. The team developed a test in mice to evaluate contact sensitization by quantitatively and objectively measuring mouse ear thickness. The results of this test were very promising.
Initially, the test was performed on 12 Wistar rats whose health was checked a week before performing the test to make sure they were healthy.
On the test day, the abdominal surface of the rats was shaved. Then, 20 μl of Freund’s complete adjuvant (FCA) and 100 μl of ZnO nanofluid product were then injected in the form of intradermal (ID) in two areas of their abdomen. On the 1 and 3 d, two of the Wistar rats were lost for some unspecified reasons.
On the 5th day, the remaining ten Wistar rats normally lived while the effects of allergy were observed on only one of them.
On the 10th day, a challenge test was performed on the ear of the remaining ten Wistar rats. In this test, 20 μl of FCA was injected into their left ear, followed by injecting 20 μl of FCA and 20 μl of ZnO nanofluid product into their right ear in the form of ID.
On the 11th day, a re-challenge test was performed on the rats and again the mixture of 20 μl of FCA and 20 μl of ZnO nanofluid product was injected intradermally in their abdominal surface.
On the 12th day, two rats were observed to develop allergy signs and the remaining eight survived without any signs or symptoms on their ear or abdominal surface.
The sizes of the remaining Wistar rats’ ears were measured using a caliper on the last day (numbers in millimetre) (mm). The numbers are shown in . The findings were promising because less than 10% change was observed in Wistar rats. The difference between the thicknesses of the left ear (control) was not significant and the ZnO nanofluid was not allergenic. It is necessary to repeat this test in a higher number in future.
Table 1. IC50 values of ZnO nanofluid in KB44, MCF-7, HeLa cell line, HT29 and HFF-2 cell lines after 24 and 48 h (in bold) of exposure.
Investigating the side effects of the ZnO nanofluid using the animal model in vivo
At first, different concentrations of 10, 25, 100, 200, 1000 and 50,000 µl/ml of ZnO nanofluid product were prepared in double distilled-sterilized water. Along with determining the various concentrations of the ZnO nanofluid, number of 70 white popular strain mice of the (Balb/c) laboratory weighing 28 g each (24 males and 46 females) were assigned to this study. In order to investigate the ZnO nanofluid size distribution, these mice were kept in a large fiberglass cage for 10 d to be blended. Afterwards, they were divided into 14 groups of 5 (each group including three females and two males) and kept in small fiberglass cages. Two control groups and 12 groups of treated with ZnO nanofluid product were investigated simultaneously. Every day, plenty of food, pellet and water were provided for them. Animal cages were cleaned every day and the conditions for keeping animals, including temperature and humidity were also controlled. For one month, morphological coordinates of each day, including weight, nutrition, body temperature, bioavailability and body colour were monitored and recorded. Afterwards, blood samples were taken from tail of each mouse for haematological examination and blood cell count, including lymphocytes, monocytes and neutrophils, were tested using solvent counterparts. In order to investigate the status of the internal organs of all studied mice, both control groups and groups treated with ZnO nanofluid, were killed by phenol (as a pharmacological laboratory manner by employing a toxic substance. Balb/c was killed using phenol solution by inhalation process) and all were dissected. After anatomical examinations and observation, the condition of the internal organs including liver, kidney, lung, spleen, heart and brain and recording their coordinates, samples were taken from each of the six organs mentioned. Then, they were placed in 10% formaldehyde solution for pharmacological survey. This new research has been done on animal labs as Balb/c mice in ZFP (Zist Faravard Pars, Tehran, Iran) company based on moral code and regards (30 December 2017, company registration number: 207,481). Additionally, morphological review and animal tissue organs were carried out at this centre while no investigation has been taken on human specimens.
Antibacterial activity tests for ZnO nanofluid product in vitro
The bacteria species worked on included Bacillus anthracis (RTCC1036), Bacillus cereus (RTCC1040), Staphylococcus aureus (RTCC1885), Staphylococcus epidermidis (RTCC1898), Streptococcus pyogenes (PTCC1447) and Listeria monocytogenes (RTCC1293), which were all gram-positive bacteria. These microorganisms were purchased from the Iranian Research Organization for the Science and Technology (IROST) and Razi Institute in Tehran, Iran. The culture media used included Muller Hinton agar, Hinton and BHI broths, which were all purchased from Merck Chemical Co (Darmstadt, Germany). Antibiotic disks and powders used as controls were Gentamycin (GM) (120 µg/ml) and Nalidixic acid (NA) disks (30 µg/ml), which were obtained from Padtan Teb Company, Tehran, Iran.
Well diffusion method
The steps of well diffusion method are as follows:
Preparation of subculture of bacteria in BHI broth medium for fresh bacteria suspension and then matching with McFarland (barium sulphate standard 0.5) turbidity standard (approximately 1.5 × 108 CFU/ml).
Preparation of Muller Hinton agar media under sterile conditions.
Preparation of lawn culture bacteria on agar plate by swab sterile.
Digging holes on culture media by a Pasture pipette and cover end of the holes by a thin layer of molten media to block spreading of ZnO nanofluid to the bottom of the plate.
Addition of 100 µl of ZnO nanofluid (three different concentrations of 8, 10 and 20 µg/ml) to each hole by a sampler.
Incubation of plates overnight at 37 °C for 24 h in an incubator and study the result by measuring the diameter of non-growth zones and comparison with the control [Citation9,Citation26].
Minimum inhibitory concentration method (MIC)
The steps of inhibitory concentration method are as follows:
Preparation of Muller Hinton broth in test tubes (1 ml in each test tube).
Providing 12 sets of media in test tubes and adding 1 ml of 100 µg/ml ZnO nanofluid suspension in the first tube and make serial dilutions in other tubes.
Overnight incubation at 37 °C for 24 h in an incubator and checking the turbidity of the media for bacteria growth in test tubes. Evaluating which concentration of ZnO nanofluid has had an antibacterial effect in comparison with the controls [Citation9,Citation26].
Minimum bactericidal concentration method (MBC)
The steps of minimum bactericidal concentration method are as follows:
Choosing the last tube of non-growth bacteria in serial dilution of MIC method and culturing it on Muller Hinton agar plate at 37 °C for 24 h to observe colony forming of bacteria (CFU/ml). Minimum bactericidal concentration means no bacteria or less than 5 CFU/ml on the agar plate. The results of all tests were compared with those of the controls [Citation9,Citation26].
Results of efficient cancer treatment in vitro, MEST in vivo, antibacterial activity in vitro and side effects in vivo
This research has four separate general parts, a, b, c, and d, respectively.
Part (a): the effects of ZnO nanofluid on cell viability
The MTT assay aimed to determine IC50 of ZnO nanofluid in KB44, MCF-7, HeLa, HT29 and HFF-2 cells. The cytotoxicity effect of ZnO nanofluid on all the mentioned cell lines was observed for 24 and 48 h. The results of MTT assay showed significant cytotoxicity in a dose-dependent manner in all cell lines (). The cell proliferation data also showed that ZnO nanofluid has a higher cytotoxic effect on KB44 cell lines than other cancer cells with an IC50 value of 30 μg/ml within 48 h of treatment (). The optimal dose and period of exposure were used in annexin V/PI staining method to confirm apoptosis. The IC50 values revealed that ZnO nanoproduct has a higher cytotoxic effect on the cancerous cell lines compared with the normal HFF-2 diploid cells ().
Figure 1. The effects of ZnO nanofluid on the cell proliferation of five different cell lines in comparison with control cells (untreated cells) within 24 and 48 h after exposure. Growth inhibition was determined using MTT assay and each data is presented as mean ± SD of three experiments. All the graphs show inhibition of cell growth in a dose-dependent manner.
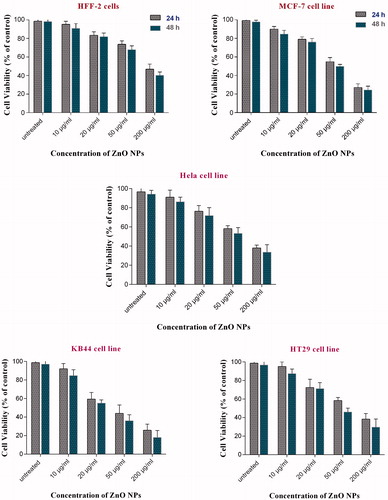
Figure 2. Cytotoxicity effects of ZnO nanofluid on KB44, MCF-7, HeLa, HT29 and normal human foreskin fibroblast (HFF-2) cells treated with various concentrations during 48 h. As shown in the graph, ZnO nanofluid growth inhibition works in significantly lower concentrations in all cancer cell lines compared to normal cells (HFF-2) (p values <.01).
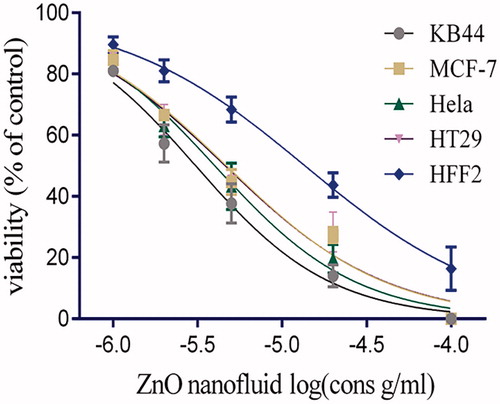
Apoptosis and necrosis analysis
Phosphatidylserine (PS) is a component of cell membrane usually restricted to internal membrane of the normal cells. PS translocation from internal pamphlet to the external one is considered as a sign of apoptosis. In addition, annexin, which is a structural protein, could bind specifically to PS on dividing cell membranes. This process allows the apoptotic cell to be discriminated from normal one using flow cytometry technique. In this study, flow cytometry results showed that KB44 cell lines underwent significant apoptotic changes (>70%) following ZnO nanofluid administration within 24 h in comparison to MCF-7 cell lines (>70%) ( and ). Annexin V/PI apoptosis detection assay results are presented in a scatter plot form, denoting the percentage of cells, which are necrotic (upper left), viable (lower left), apoptotic (lower right) and late apoptotic (upper right). Quantitative analysis of the cells at the early and late stages of apoptosis revealed that the proportion of early and late stage apoptotic KB44 cells are significantly high 24 h after treatment (18 and 30%, respectively) while most of the mentioned population come to necrotic stage after 48 h (). Apoptosis process in ZnO nanofluid treated MCF-7 cells showed approximately the same percentage of early and late apoptotic cells (15 and 22%, respectively) within 24 h while the proportion of MCF-7 cells went much higher after 48 h (up to 60%) (). Interestingly, HT29 cell line showed about 20% of the necrotic cell with the lowest proportion of early and late stage population (less than 5%) after 24 h (). Finally, annexin V/PI staining of HeLa cell lines showed that the proportion of early and late apoptotic cells are 19 and 13%, respectively, during 24 h, while the population of late apoptotic cells reaches about 22% after 48 h (). Moreover, our achievements using ZnO nanofluid containing fine ZnO QD NPs induced apoptosis mainly through increased intracellular ROS production. In this regard, some changes took place in their cell membrane morphologies, such as induced collapse of membrane, cell decomposition and death, eventually.
Figure 3. Flow cytometry analysis is demonstrating the percentage of apoptotic and necrotic cells using annexin V/PI staining comparing untreated cells as normal control. (A) KB44 cell lines treated with 50 μg/ml ZnO nanofluid, exposure induced apoptosis in 48% (apoptotic/necrotic) cells after 24 h, whereas near 70% of the cells reach necrosis state after 48 h; (B) MCF-7 cell lines treated with 50 μg/ml ZnO nanofluid, which induced apoptosis in 35% of the cells (apoptotic/necrotic) after 24 h while this phenomenon reached to more than 90% after 48 h; (C) HT29 cells treated with 40 μg/ml ZnO nanofluid. This dose of the compound drove 27% of the cells to necrosis 24 h after exposure whereas 46% of the cells can be observed in apoptotic/necrotic state after 48 h; (D) HeLa cell lines treated with 40 μg/ml ZnO nanofluid. This treatment induced apoptosis of nearly 32% of the cells (apoptotic/necrotic) after 24 h, and this population remained approximately the same after 48 h.
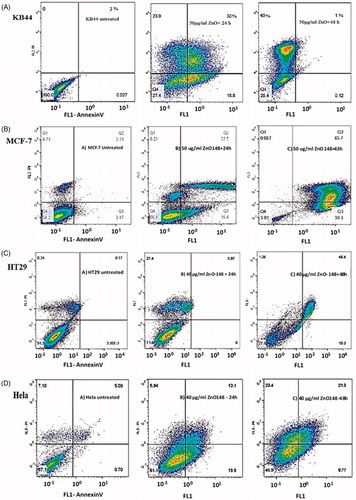
Figure 4. Microscopic images of MCF-7 cells after treatment with 40 μg/ml of ZnO nanofluid for 24 and 48 h’ exposure, showing cell shrinkage and rounding within 48 h compared with untreated control cells (A); KB44 cell line after treatment with 30 μg/ml of ZnO nanofluid at the same incubation periods showing condensation in their nuclei and cell shrinkage within 24 h (B); HeLa cell lines after treatment with 40 μg/ml of ZnO nanofluid showing distinct morphological changes including rounding and shrinkage within 24 h. These changes increased to nuclear condensation after 48 h (C); HT29 cell lines (40 µg/ml) indicating the morphological hallmark of the apoptosis including shrinkage, nuclear condensation and formation of pyknotic bodies (D).
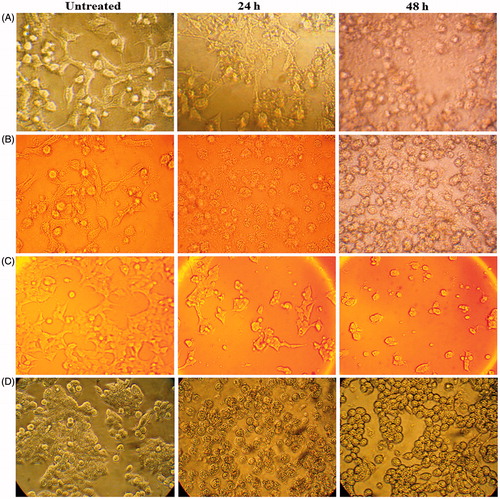
The morphological changes and microscopic images of MCF7, KB44, HeLa cell and HT29 cancer cell lines after treatment with 40, 30, 40 and 35 µg/ml ZnO nanofluid products can be observed in . The damaged or destroyed textures after inducing antitumor ZnO nanofluid are clearly indicated as deformation and agglomeration pictures. These tests showed that this nanomedicine has high ability and potential for mouth, breast, HeLa and colon cancer cell lines compared to the other drugs used in hospitals today. The green nanofluid is very stable, soluble in water and cheap.
Part (b): MEST sensitization design in vivo
An investigation was conducted for evaluating the ability of a MEST procedure as a useful model for identifying strong contact sensitizers, prediction of contact sensitization induced by the ZnO synthesized nanofluid, and the results during 12 d in vivo, respectively, as shown in . Two of the mice died on the 1 and 7 d for no logical reason. However, the rest of them remained healthy and no allergy and sensitivity was observed due to ZnO nanofluid injection in behind of ears of the mice.
Table 2. Investigation of allergic symptoms in Wistar rats.
The MEST test for evaluation of the sensitivity of ZnO nanofluid formulated in distilled water-based revealed no side effects, such as skin rashes, itching, burn, filthy scorch and allergic reactions after an appropriate time of effectiveness where the nanofluid was injected to the left rear of a mouse ear. In addition, most of the mice were alive. This is very good news for designing and performing the clinical tests on animals, which are planned to be considered in our next research. Therefore, very promising in vivo results related to the effective compatibility and differential cytotoxicity in anticancer strategy have been obtained. Moreover, the positive points of MEST method (as a sensitivity test on Wistar rats) are highlighted towards biomedical applications.
Part (c): the final presentation of six types of biological activity of microorganisms obtained by ZnO nanofluid product
Biological reactions against six significant types of gram-positive bacteria related to GM and NA control samples by well diffusion, MIC and MBC methods are presented in .
Table 3. The assay of ZnO nanofluid as bacterial drugs against gram-positive bacteria activity.
displays that ZnO nanofluid is most effective on S. pyogenes in well method with an inhibition zone of 50 mm, MIC of 1.56 and MBC of 6.25 µg/ml. In addition, ZnO nanofluid is most effective on B. anthracis in well method with an inhibition zone of 35 mm, MIC of 6.25 and MBC of 25 µg/ml. ZnO nanofluid is most effective on L. monocytogenes in Well method with an inhibition zone of 30 mm, MIC of 6.25 and MBC of 25 µg/ml. It is also reliably effective on S. aureus and B. cereus in well method with an inhibition zone of 25, MIC of 12.5 and MBC of 50 µg/ml and on S. epidermidis with an inhibition zone of 20, MIC of 25 and MBC of 100 µg/ml, accordingly. Totally, the results of the recent research show that ZnO nanofluid is able to provide a much better effect than GM and NA as controls. In addition, the major influences of nanofluid product on bacteria, which cause important hospital infections, such as pneumonia, endocarditis, gangrene, meningitis, urinary tract infection and gastroenteritis, have been observed.
Selected images of ZnO nanofluid’s effects on important gram-positive bacteria
Four different pictures containing three concentrations (8, 10 and 20 µg/ml, shown by arrows) of ZnO nanofluid product by well method are shown in with the various inhibition zones by four anti-bacterial. The best concentration for all tests was 20 µg/ml, which is shown by arrows.
Figure 5. The best results of inhibition zone of antibacterial with the best concentration (20 µg/ml) of ZnO nanofluid (between 8 and 10 µg/ml concentrations) by well method with inhibition zone in the pictures: (A) B. anthracis (35 mm), (B) S. aureus (25 mm), (C) S. pyogenes (50 mm) and (D) L. monocytogenes (30 mm). All test results were compared with those of GM and NA controls.
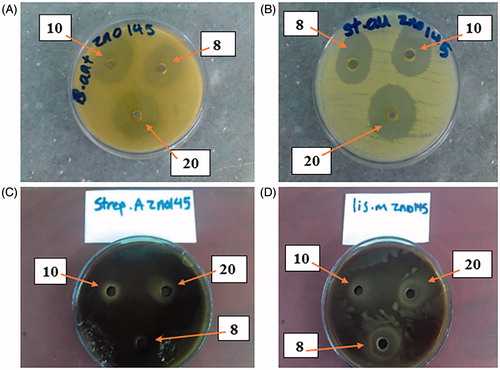
Part (d): the results of side effect of ZnO nanofluid
In morphological studies, animals treated with ZnO nanofluid product were completely normal in terms of weight, temperature (fever), body colour, nutrition and motility (biological activity).
In hematologic studies, all treated mice were completely normal in comparison with the control group and their average blood neutrophil, lymphocyte and monocyte count were 50, 34, and 16%, respectively. In anatomical studies, all treated mice were completely normal in size, colour and appearance of the kidneys, heart, brain and lungs. But the size and colour of the liver and spleen in the treated mice had been changed only at the highest concentration (50,000 µg/ml) of ZnO nanofluid (2 of 5 mice showed slightly large swelling of the liver and had enlarged spleens). Precisely, of the 70 mice tested, only 3–4 of them exhibited allergic to high doses of ZnO nanofluid. While the rest remained healthy and even got pregnant and gave birth to healthy babies. In the pathological studies, a photoreactor photogrammed from all tissues of spleen, lung, heart and brain of treated mice compared with the control groups showed completely normal and there were no especial abnormalities. In the study of the teratogenic effects of ZnO nanofluid for all mice treated at all concentrations, the female mice were all pregnant and their delivery was completely normal and no malformations were observed in their newborns in comparison with the control groups. Finally, according to the significant findings of this new study, different concentrations (10–50,000 µg/ml) of ZnO nanofluid used have no adverse effects on lungs, spleen, heart, brain tissues and blood. However, the liver and kidney tissues were affected by unknown reasons at high doses of ZnO nanofluid due to an allergic reaction to medication. In this regard, shows the morphological patterns of kidney, spleen, cerebellum, (1) as control groups and (2) after the intratracheal instillation of ZnO nanofluid. depicted the morphological patterns of heart, liver and hung before (1) as references and after (2) the intratracheal instillation of ZnO nanofluid. The histological section of the mice liver that has degeneration and slightly changes in texture is indicated in , describing the mice photos before the injection of ZnO nanofluid (1– as references) and after receiving the drug (2), both displayed in two magnifications of 200× and 400× Correspondingly, it can be seen eight extra images of the histological sections in Supplementary material that present in 400× magnifications, shown as Figures S1–S8.
Figure 6. Different morphological patterns of kidney, spleen and cerebellum. (1) References and (2) display after injection of ZnO nanofluid.
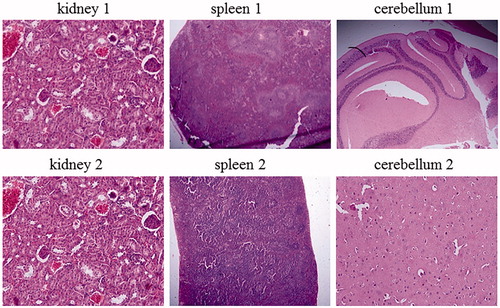
Discussion
Characterization techniques of ZnO QD NPs in nanofluid product
SEM images of typical unmodified ZnO NPs vs. energetic surface modification of ZnO NPs
SEM (Philips, XL30) images of fine spherical shaped, simple ZnO NPs with hexagonal crystallographic phase without any surface modification are shown in . shows the various SEM morphologies of interesting ZnO QD NPs immersed in the fatty acid layers and surface functionalized by green fatty carboxylic acid. In addition, shows the SEM images of ZnO over PVP homogeneous nanocomposites as nano spherical morphologies. The latter nanocomposites play very important roles in co-assisting ZnO QD NPs in attacking process and make them stable for long periods of time.
Figure 9. SEM images of spherical shape ZnO NPs with unmodified surfaces by a carboxylic acid agent (A). SEM images of ZnO QD NPs in different shapes (nanospherical and nanorods) in eco-friendly green fatty carboxylic acid layers. They can generate smart surfaces for targeted attacking ZnO NPs to bacterial cell walls or cell membrane of cancerous cell lines, and this is a fantastic point for diffusion of NPs as the strong attacker (B). SEM images of very beautiful and attractive ZnO NPs as unique synergistic agents in nanofluids which their surfaces were modified by PVP food polymer (C).
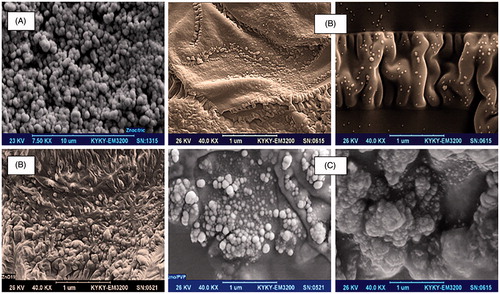
FTIR and photoluminescence (PL) of ZnO QD NPs in nanofluid
FTIR spectrum of ZnO QD NPs (Bruker Co. model Tensor 27, Billerica, MA) in KBr matrix and five photoluminescence (Varian model Carry Eclipse, Agilent Technologies, Santa Clara, CA) spectra in various colour wavelengths, namely 227 (red curve), 259 (green curve), 280 (blue curve), 330 (orange curve) and 370 nm (black curve), are shown in . Structural defects (oxygen vacancies and zinc-oxygen interstitials) are also indicated by lots of max and min. In the fluorescence spectroscopy peaks, the blue bands were due to structural defects in the ZnO NPs and the green bands were caused by the oxygen transition vacancy (the gap between the electron transfer from conduction and valance bands in semiconductor NPs and production of oxygen radicals owing to quantum confinement effect, which can exhibit various colours) [Citation7,Citation33].
Figure 10. FTIR spectrum (A) and five photoluminescence (PL) (B) at various wavelengths of ZnO QD NPs.
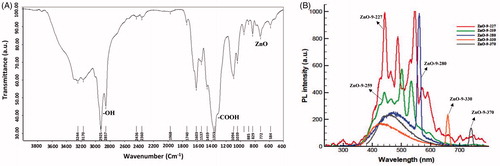
The clear frequency of ZnO NPs located at 555 cm−1 corresponds to hexagonal ZnO bond. The sharp peak associated with carboxylic acid and zinc carboxylate (COO−) groups is observed at 1353 cm−1. Photoluminescence spectra of ZnO QD NPs in five different coloured curves and various wavelengths are represented. Blue, red and green fluorescence emissions can be found because of many unique trapping states. Besides, electron–hole pairs, crystal defects, high-superficial surface energy, reactive sites due to smart surface modification and specific structural characteristics and physicochemical properties are especial characteristic for QD NPs. Semiconductor ZnO QD NPs usually show inimitable optical properties, and fluorescence spectroscopy was used to assess the strong photoluminescence (PL) emission properties of fine ZnO NPs and potential applications in biological fluorescent labels. The nanoproduct in this study illustrates minimum and maximum absorptions in the ultraviolet light at 282.8 and 297.7 nm, respectively, at 252 nm wavelength of light emitted to the ZnO nanofluid as shown in (). This significantly influences the toxicological effects of ZnO nanofluid.
Figure 11. Photoluminescence (PL) emission at 252 nm wavelength of light emitted to ZnO nanofluid (A), trap state shifts and broadening peaks measured under UV radiation. ZnO QD NPs exhibited high absorption peaks at 194.3 and 205.0 nm and a few low ladder-shaped peaks in UV–visible in (B). Therefore, this nanoplatform exhibited strong blue fluorescence.
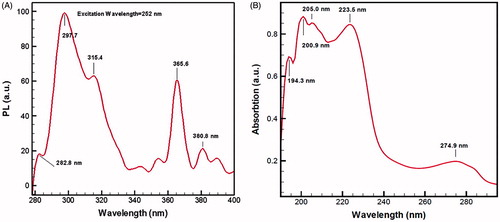
UV–Vis absorption spectroscopy of ZnO water-based nanofluid
UV–Vis absorption spectrum of ZnO nanofluid shown in has shifted to broad strong peak at 194.3 nm (blue emission) due to oxygen vacancies and atomic active sites on modified surface defects. Therefore, the mean particle size of around 1–4 nm and high optical band gap energy of 4.8 eV for ZnO QD NPs surrounded by green soya fatty acid and 5.32 eV for ZnO nanofluid formulation was determined in this research. Accordingly, wider band gap energy plays a key role in the production of oxygen radicals (ROS), great diffusion ability, structural defects and reactive surface sites, which lead to biological purposes and toxicological response of semiconductors as ZnO NPs in cancer therapy [Citation10,Citation34]. Undoubtedly, ZnO nanofluid has shown to produce large surface area, high-surface energy (UV absorption), 5.32 eV band gap energy, defect structures, oxygen vacancies on modified smart surface and generation of free radicals after interaction of NPs with tumour membrane cells in nano-bio interface. In addition, positive zeta-potential (ζ) for ZnO QDs NPs was measured to be +50 mV and the cancerous cell membrane is also highly electronegative [Citation1]. Therefore, the ZnO QD NPs can penetrate into the bacteria wall and produce high attraction and absorption forces between opposite charges by strong electrostatic interaction and electrovalence bonding. Owing to the generating of stable bridges hydrogen bonding within amino acids of proteins. Consequently, ZnO NPs can form greater reactive oxygen species (ROS) with subsequent oxidative damage to cellular structures. ROS generation and subsequent effects can finally result in apoptosis [Citation18,Citation22,Citation35] and eventually cell death, as some of the changes took place in their membrane morphologies, such as induce collapse of membrane and cell decomposition.
Determination of surface tension and reduction of interfacial tension for ZnO nanofluid
Surface tension (ST) and interfacial tension (IFT) are two effective factors in fluid science. The energy difference in the vicinity of the interface between two immiscible fluids plays a very important role in the distribution and movement functions [Citation36]. These are biochemical reactions occurring at the surface and interface. It was found that human biological nanofluids also have a significant effect on the reduction of IFT and ST, which can facilitate the movement of nanofluid and NPs within the cell membrane and provide faster movement in the blood flow. These nanofluids are capable of adsorption at fluid interfaces and are useful tools for monitoring treatment efficacy for clinical applications. Accordingly, they can be applied to diagnose cancer between a sick and healthy person (reference control). Nanoscientists are racing to develop special tests to identify obtained changes in the ST behaviour of human biological fluids, such as changes in the cell surface contact and control of the cell shape through measuring these two factors [Citation37]. In , ST (between ZnO nanofluid and air using a Wilhelmy plate method) and IFT reduction (between ZnO nanofluid and normal decane using a du Noüy ring method (Sigma 701 model, St. Louis, MO)) were determined in comparison with distilled water, air and normal decane references at 25 °C. It is notable that this method will bring new insights and is an important advantage in the prevention of the onset of cancer disease because the majority of the tumours are resistant to current drugs.
Table 4. Surface and interfacial tension of nanofluid and references.
The findings have shown that ZnO nanofluid with unique formulation could reduce ST and IFT between air and water and water/n-decane system. The possible reason of the negative IFT between n-decane and nanofluid interface can be the non-equilibrium effect between the two stable phases. The presence of a strong surfactant in the nanoformulation, and or the presence of very active NPs in the nanofluid can also cause Brownian motion. Under these conditions, active surface NPs are able to create very large surface-to-volume ratio due to full energetic surface modified, including high-interfacial potential and tendency to combine with surfactants in order to generate significant changes in the IFT and ST [Citation38].
Biological application of ZnO nanofluid as an antibiotic agent in vitro
Since there are many bacteria, which cause infections in human, research regarding methods for treatment of diseases that resist to antibiotics and hospital infections is of great significance. In this study, the formulated ZnO NPs show excellent antimicrobial activity compared with the controls towards pathogenic bacteria. Staphylococcus aureus is one of the most important causes of acquired infections in hospitals and has become one of the most important health problems in the world. Although S. epidermidis is normal flora in human, it can be opportunistic in sensitive immune systems [Citation39]. Based on the obtained results, ZnO nanofluid reliably affects S. aureus with a non-growth zone of 25 mm in well method and MIC and MBC values of 12.5 and 50 µg/ml, respectively, compared to healthy controls. Also, ZnO nanofluid efficiently affects S. epidermidis with a non-growth zone of 20 mm in well method and MIC and MBC values of 25 and 100 µg/ml, respectively, comparison with the controls.
As we know, group A beta-haemolytic of Streptococcus causes most common diseases, such as rheumatic fever, endocarditis and meningitis. Streptococcus pyogenes referred as flesh-eating bacteria, is another important species and responsible for widespread variety of human diseases [Citation40]. According to pathogenic potential and increasing resistance of this family to antibacterial agents, smart ZnO nanofluids can be a promising platform and new pathway to this big problem for curing patient in the future.
In this research, ZnO nanofluid most successfully affected S. pyogenes infections with a non-growth zone of 50 mm in well method and MIC and MBC values of 1.56 and 6.25 µg/ml, respectively, compared to the references. Anthrax is a common disease between humans and animals, such as cattle and sheep and is considered an important bacterium in the development of cutaneous anthrax and pulmonary infections, which can result in pneumonia, blood infection and finally death. Regarding these results, this nanoformulation product strongly affects B. anthracis, with a non-growth zone of 35 mm in well method and MIC and MBC values of 6.25 and 25 µg/ml, respectively. Furthermore, food poisoning is one of the most important diseases caused by a B. cereus gram-positive bacterium, which generates toxins, and is commonly found in many raw and processed foods or in soil and vegetables. This nanofluid product created the best-affected B. cereus with a non-growth zone of 25 mm in well method and MIC and MBC values of 12.5 and 50 µg/ml, respectively. Listeriosis, which is a common disease between humans and animals, is also naturally caused by food derived from animal milk and eggs [Citation41,Citation42]. Results have shown the very good effect of ZnO nanofluid on L. monocytogenes, with a non-growth zone of 30 mm in well method and MIC and MBC values of 6.25 and 25 µg/ml, respectively, relative to controls (25 and 0, respectively). ZnO nanofluid product can then be a promising antibiotic for the treatment of typical cases of listeriosis. In a recent research by Behzad et al. the effects of ZnO nanofluid and trachyspremum copticum oil (a kind of spice) on foodborne pathogens, such as B. cereus, S. aureus, Escherichia coli (E. coli) and Salmonella enterica, were examined in 2016. The diameters of inhibition zones in disk method varied in the ranges of 28–33 and 18–37 mm for concentrations of 1000, 500 and 300 µg/ml of ZnO nanofluid for B. cereus and S. aureus, respectively. MIC values for ZnO nanofluid against B. cereus and S. aureus were 250 µg/ml and MBC values were 250 and 500 µg/ml, respectively [Citation43]. Obviously, our new antibacterial achievements granted by employing least concentrations of ZnO nanofluid formulation have shown the best antibacterial effect on the mentioned bacteria species in comparison with controls.
Considering the remarkable effects of the antibacterial formulation of zinc oxide QD NPs as active nanofluids on gram-positive bacteria, especially in hospital infections. However, this new nanoformulation can give great help in medical equipment, such as tubes, catheter, hospital dresses and hygienic materials related to the patient and can also be used as an alternative for antibiotics in tablets and ointments as antibacterial agents in medicine.
Side effect of ZnO nanofluid
As a final interpretation, according to the results of this study, low doses of ZnO nanofluid in cancer treatment cause no side effect as toxicity in liver. Additionally, the photomicrographs of tissue sections show no abnormal texture building in major organs, except 3–4 out of 70 mice where hepatocytes indicate slightly degeneration in hepatic tissue section, which may be due to the specific reaction of their body structure and sensitivity of them to the high doses of the nanofluid drug allergy. Prosperously, after 60 d of nanofluid treatment, most of the treated mice did not show any reaction and female mice were able to become pregnant and give birth to healthy children, even in high doses.
Conclusions
In this nanomedical research, it was attempted to produce new green, and eco-friendly water-based ZnO nanofluid containing ZnO QD NPs, co-assistant nanopolymer, wetting agent ethoxylates, binding agents and finally water in oil emulsifiers, formulated in polar solvents and distilled water for the first time. This nanofluid product is yellow coloured and very soluble in distilled water and can thus be fed orally to patients, preventing the psychological patient’s stress from the injection. The nanofluid was characterized by SEM, FTIR, photoluminescence (PL), wide band gap energy (5.32 eV) and UV–Vis blue shift spectroscopy. The results showed very strong penetration and high-diffusion capability of the nanofluid into the membrane of cancerous cells (KB44, MCF-7, HT29 and HeLa cell lines) through strong electrostatic interactions and stable chemical hydrogen bonding with the amino acids of proteins. IC50 (μg/ml) values were measured for these cell lines within 24 and 48 h and reported as 30, 41, 40 and 35 μg/ml for 48 h in comparison with normal HFF-2 diploid cells (p < .01). Apoptosis and necrosis analysis of these cell lines were conducted by annexin V/PI staining using flow cytometry after exposure with 40–50 μg/ml of ZnO nanofluid within 24 and 48 h. The results also suggest that the major mechanism of cell death mediated by ZnO nanofluid is generation of ROS and oxidative stress induction. On the other hand, our goal was to consider the medical impact and response of ZnO nanofluid to the animal model. Related to specifying nanoproduct interaction and allergic reactions with the body, the MEST was also carried out with 12 Wistar rats, in which less than 10% changes on Wistar rats was observed. The photomicrographs of tissue sections were found to show no abnormal texture building, except for liver and kidney tissue section. They indicate slightly degeneration in texture of their morphologies which may be due to high doses of the ZnO nanofluid drug allergy. Totally, this green and safe nanoproduct can be suggested as a new generation of antibacterial and antifungal platform in the future because of its higher functional potential than clotrimazole, which is currently used in clinics now. Since most of the cancerous patients have many problems in their mouth cavity due to candidiasis via Candida albicans PTCC 5027 fungi, it is necessary that the physicians and pharmacists must know new pharmacological activity, toxicity potential and side effects regarding newly synthesized drugs. Hence, herein we may claim that this new multi-purpose ZnO nanofluid formulation can be introduced to the nanomedical global market after completing its clinical trial.
Supplementary_material.pdf
Download PDF (1.2 MB)Disclosure statement
The authors report no conflicts of interest and are responsible for the content and writing of the manuscript.
References
- Chen T, Zhao T, Wei D, et al. Core–shell nanocarriers with ZnO quantum dots-conjugated Au nanoparticle for tumor-targeted drug delivery. Carbo Poly. 2013;92:1124–1132.
- Samir TM, Mansour MM, Kazmierczak SC, et al. Quantum dots: heralding a brighter future for clinical diagnostics. Nanomedicine (Lond). 2012;7:1755–1769.
- Chinnathambi S, Chen S, Ganesan S, et al. Silicon quantum dots for biological applications. Adv Healthcare Mater. 2014;3:10–29.
- Bisht G, Rayamajhi S. ZnO nanoparticles: a promising anticancer agent. Nanobiomedicine. 2016;3:1–11.
- Rasmussen JW, Martinez E, Louka P, et al. Zinc oxide nanoparticles for selective destruction of tumor cells and potential for drug delivery applications. Expert Opin Drug Deliv. 2010;7:1063–1077.
- Malhotra SPK, Mandal TK. Biomedical applications of zinc oxide nanomaterials in cancer treatment: a review. SCIREA J Chem. 2016;1:67–89.
- Gulia S, Kakkar R. ZnO quantum dots for biomedical applications. Adv Mat Lett. 2013;4:876–887.
- Bedi PS, Kaur A. An overview on uses of zinc oxide nanoparticles. World J Pharm Sci. 2015;4:1177–1196.
- Fakhroueian Z, Dehshiri AM, Katouzian F, et al. In vitro cytotoxic effects of modified zinc oxide quantum dots on breast cancer cell lines (MCF7), colon cancer cell lines (HT29) and various fungi. J Nanopart Res. 2014;16:2483–2497.
- Abdolmohammadi MH, Fallahian F, Fakhroueian Z, et al. Application of new ZnO nanoformulation and Ag/Fe/ZnOnanocomposites as water-based nanofluids to consider in vitro cytotoxic effects against MCF-7 breast cancer cells. Artif Cells Nanomed Biotechnol. 2017;45:1769–1777.
- Ma YY, Ding H, Xiong HM. Folic acid functionalized ZnO quantum dots for targeted cancer cell imaging. Nanotechnology. 2015;26:3057027.
- Vidhya K, Saravanan M, Devarajan VP, et al. Green synthesis and anti-cancer activity of multifunctional ZnO:Mn-natural biomolecule quantum dots system. Malaya J Bios. 2014;1:267–272.
- Akhtar MJ, Ahamed M, Alhadlaq HA, et al. Targeted anticancer therapy: overexpressed receptors and nanotechnology. Clin Chim Acta. 2014;436:78–92.
- Namvar F, Rahman HR, Mohamad R, et al. Cytotoxic effects of biosynthesized zinc oxide nanoparticles on murine cell lines. Evid Based Complementary Altern Med. 2015;2015:1–11.
- Singh H, Du J, Yi TH. Green and rapid synthesis of silver nanoparticles using Borago officinalis leaf extract: anticancer and antibacterial activities. Artificial Cells Nanomed Biotechnol. 2017;45:1310–1316.
- Cai X, Luo Y, Zhang W, et al. pH-Sensitive ZnO quantum dots-doxorubicin nanoparticles for lung cancer targeted drug delivery. ACS Appl Mater Interfaces. 2016;8:22442–22450.
- Ye DX, Ma YY, Zhao W, et al. ZnO-based nanoplatforms for labeling and treatment of mouse tumors without detectable toxic side effects. ACS Nano. 2016;10:4294–4300.
- Wang C, Hu X, Gao Y, et al. ZnO nanoparticles treatment induces apoptosis by increasing intracellular ROS levels in LTEP-a-2 cells. Bio Med Res Int. 2015;2015:1–9.
- Selvakumari D, Deepa R, Mahalakshmi V, et al. Anti cancer activity of ZnO nanoparticles on MCF7 (breast cancer cell) and A549 (lung cancer cell). ARPN J Eng Appl Sci. 2015;10:5418–5421.
- Noori A, Karimi F, Fatahian S, et al. Effects of zinc oxide nanoparticles on renal function in mice. Int J Biosci. 2014;5:140–146.
- Wahab R, Kaushik N, Khan F, et al. Self-styled ZnO nanostructures promotes the cancer cell damage and suppresses the epithelial phenotype of glioblastoma. Sci Rep. 2016;6:1–13.
- Sirelkhatim A, Mahmud S, Seeni A, et al. Physico-chemical characteristics of ZnO nanoparticles- based discs and toxic effect on human cervical cancer HeLa cells. AIP Conf Proc. 2014;1621:670.
- Shanker K, Naradala J, Mohan GK, et al. A sub-acute oral toxicity analysis and comparative in vivo anti-diabetic activity of zinc oxide, cerium oxide, silver nanoparticles, and Momordica charantia in streptozotocin-induced diabetic Wistar rats. RSC Adv. 2017;7:37158–37167.
- Jacobsen NR, Stoeger T, Brule SVD, et al. Acute and subacute pulmonary toxicity and mortality in mice after intratracheal instillation of ZnO nanoparticles in three laboratories. Food Chem Toxicol. 2015;85:84–95.
- Moussodia RO, Balan L, Merlin C, et al. Biocompatible and stable ZnO quantum dots generated by functionalization with siloxane-core PAMAM dendron. J Mater Chem. 2010;20:1147–1155.
- Fakhroueian Z, Harsini FM, Chalabian F, et al. Influence of modified ZnO quantum dots and nanostructures as new antibacterials. Adv Nanopart. 2013;2:247–258.
- Katouzian F, Fakhroueian Z, Bidhendi SM. The interesting of antifungal effects of novel in vitro fabrics of stabilized ZnO nanofluids. Adv Nanopart. 2015;5:206–223.
- Yang W, Wang L, Lu X, et al. Enhancing luminescence of ZnO quantum dots by PEG and oleic acid via a sol–gel method. J Mater Sci Mater Electron. 2015;26:1113–1118.
- Rissi NC, Hammer P, Chiavacci LA. Surface modification of ZnO quantum dots by organosilanes and oleic acid with enhanced luminescence for potential biological application. Mater Res Express. 2017;4:015027.
- Guo L, Yang S. Synthesis and characterization of Poly (vinylpyrrolidone)-modified zinc oxide nanoparticles. Chem Mater. 2000;12:2268–2274.
- Siddiqui MA, Singh G, Kashyap MP, et al. Influence of cytotoxic doses of 4-hydroxynonenal on selected neurotransmitter receptors in PC-12 cells. Toxicol In Vitro. 2008;22:1681–1688.
- Gad SC. The mouse ear swelling test (MEST) in the 1990s. Toxicol. 1994;93:33–46.
- Sirelkhatim A, Mahmud S, Seeni A, et al. Preferential cytotoxicity of ZnO nanoparticle towards cervical cancer cells induced by ROS-mediated apoptosis and cell cycle arrest for cancer therapy. J Nanopart Res. 2016;18:219.
- Li CY, Zhang ZC, Mao JY, et al. Preparation of Tradescantia pallida-mediated zinc oxide nanoparticles and their activity against cervical cancer cell lines. Trop J Pharm Res. 2017;16:494–500.
- Ostrovsky S, Kazimirsky G, Gedanken A, et al. Selective cytotoxic effect of ZnO nanoparticles on glioma cells. Nano Res. 2009;2:882–890.
- Esmaeilzadeh P, Fakhroueian Z, Nadafpour M, et al. Application of ZnO nanostructures in improvement of effective surface parameters in EOR Process. J Nano Res. 2014;26:9–16.
- Azarbayjani AF, Jouyban A. Surface tension in human pathophysiology and its application as a medical diagnostic tool. Bioimpacts. 2015;5:29–44.
- Karimi A, Fakhroueian Z, Bahramian A, et al. Wettability alteration in carbonates using zirconium oxide nanofluids: EOR implications. Energy Fuels. 2012;26:1028–1036.
- Kluytmans J, van BA, Verbrugh H. Nasal carriage of Staphylococcus aureus: epidemiology, underlying mechanisms, and associated risks. Clin Microbiol Rev. 1997;10:505–520.
- Facklam R. What happened to the streptococci: overview of taxonomic and nomenclature changes. Clin Microbiol Rev. 2002;15:613–630.
- Tayel AA, EL-Tras WF, Moussa S, et al. Antibacterial action of zinc oxide nanoparticles against foodborne pathogens. J Food Safety. 2011;31:211–218.
- Mirhosseini M, Firouzabadi FB. Antibacterial activity of zinc oxide nanoparticle suspensions on food-borne pathogens. Int J Dairy Technol. 2012;65:291–295.
- Behzad J, Sani AM. Antimicrobial effect of nanofluid including zinc oxide (ZnO) nanoparticle and trachyspermum copticum essential oils on food borne pathogens. Biotechnol Ind J. 2016;12:1–9.