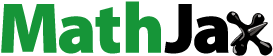
Abstract
Purpose: The purpose of this study was to investigate apoptotic activity of silver nanoparticle Clinacanthus nutans (AgNps-CN) towards HSC-4 cell lines (oral squamous cell carcinoma cell lines).
Methods: Methods involved were MTT assay (cytotoxic activity), morphological cells analysis, flow cytometry and cell cycle analysis and western blot.
Results: MTT assay revealed IC50 concentration was 1.61 µg/mL, 3T3-L1 cell lines were used to determine whether AgNps-CN is cytotoxic to normal cells. At the highest concentration (3 µg/mL), no cytotoxic activity has been observed. Flow cytometry assay revealed AgNps-CN caused apoptosis effects towards HSC-4 cell lines with significant changes were observed at G1 phase when compared with untreated cells. Morphological cells analysis revealed that most of the cells exhibit apoptosis characteristics rather than necrosis. Protein study revealed that ratio of Bax/Bcl-2 increased mainly due to down-regulation of Bcl-2 expression.
Conclusion: AgNps-CN have shown potential in inhibiting HSC-4 cell lines. IC50 was low compared to few studies involving biosynthesized of silver nanoparticles. Apoptosis effects were shown towards HSC-4 cell lines by the increased in Bax/Bcl-2 protein ratio. Further study such as PCR or in vivo studies are required.
Introduction
Nanotechnology field is one of the most active research areas in these past decades. There are lots of published articles emphasized in improvement of nanotechnology and medicinal health sciences is one of the areas that affected the most. Nanoparticles have been actively studied for its properties such as its antimicrobial and anticancer properties [Citation1]. Gold, silver and metal have been used to synthesize nanoparticles. As the areas grown, there is still a need for economical, commercially viable and environmentally clean synthesis route to synthesize it. Green synthesis is one of the methods that has been developed to synthesize silver nanoparticle in environmental friendly conditions. It uses plant extracts as reducing and capping agents [Citation2]. For example, Argemone mexicana leaves extract, seeds extract of Jatropha curcas, neem leaves tea extract and Cocous nucifera are several plants used in green synthesis of silver nanoparticle [Citation3–6]. Besides act as reduction and capping agents, bioactives such as phenolic compounds may bind to silver nanoparticles and enhance its medicinal properties. There are several studies reported phenolic compounds of the extract may enhance anticancer properties of silver nanoparticles. For example, silver nanoparticles synthesized by Commelina nudiflora caused cytotoxic activity towards human colon cancer cell lines [Citation7]. Besides that, it increased the expression of caspase-3, caspase-8 and caspase-9 which involved in apoptosis mechanism [Citation7]. Therefore, many plant crude extracts that have anticancer properties had been studied in their silver nanoparticle forms. Clinacanthus nutans is one of the plants that have been reported to possess anticancer properties [Citation8]. Recently, it has been reported C. nutans have antiproliferative effects on various cancer cell lines and exhibit apoptosis effects on liver cancer cell lines, but no study has been done to determine how silver nanoparticle of this crude extract will act against oral cancer [Citation8,Citation9].
Apoptosis is one of the most studied areas in developing anticancer drugs. Apoptosis is a programmed cell death that occurs physiologically and pathologically [Citation10]. In tumour pathogenesis, when there is defects in apoptosis mechanism, it allows neoplastic cells to avoid cell cycle arrest and cause the cells to survive over the intended lifespans, therefore provides protection from oxidative stress and tumour hypoxia as the tumour expands. In the meantime, this allows accumulations of genetic alterations that will deregulate cells proliferation, interfere with differentiation, promotes angiogenesis and lastly increase invasiveness of tumour progressions [Citation10–12]. Oral squamous cell carcinoma (OSCC) is the 6th commonest malignancy occurs in male and 10th commonest malignancy occur in females worldwide [Citation13,Citation14]. In OSCC, 50% of the tumours have dysfunctional p53 which is the most important genes in apoptosis mechanism and in check points control [Citation15]. While major therapeutics strategies for OSCC are combination of surgery and radiation therapy, but 5 years survival rate still not improve significantly [Citation16–18]. Besides that, the treatment gives negative side effects to patients in term of appearance and oral functions [Citation18]. Many researchers have developed their interest to study drugs, plants or traditional herbs as an alternate regimen for OSCC in order to increase survival rate with less or no negative side effects. Hence, this study was done to determine apoptosis effects of AgNps-CN towards HSC-4 cell lines.
Material and methods
Preparation of extracts
Clinacanthus nutans leaves were cleaned, separated and oven-dried at 45 °C. The leaves were ground into fine powder and soaked into chloroform, ethanol and distilled water at room temperature. All extracts were collected separately in clean glass bottles and filtered using Whatman no. 1 paper. Chloroform and ethanol filtrates were dried using rotary evaporator while water filtrate was freeze dried. All extracts were kept at −20 °C until further use.
Green synthesis of silver nanoparticle
For synthesis of silver nanoparticle, 1 mM of silver nitrate were mixed with C. nutans leaves aqueous extract and incubated in room temperature until transformations of colour to reddish brown colour which indicated the formation of AgNps.
Cell culture
Human squamous carcinoma, HSC-4 cell lines were purchased from Riken (Wako, Japan) while normal mouse embryonic fibroblast 3T3-L1 purchased from American type culture collection (ATCC, USA). Both cell lines were cultured in RPMI 1640 medium mixed with 10% foetal bovine serum (FBS), 1% penicillin (100 U/mL) and streptomycin (100 µg/mL). Both cell lines were maintained at 37 °C in 5% humidified CO2 incubator.
Cell viability assay
Both HSC-4 and 3T3-L1 cell lines were seeded in 96 well plates with density of 1 × 105 per well. Cells were then incubated at 37 °C in 5% humidified CO2 incubator for 24 h. After that, cultured cells were treated with silver nanoparticle C. nutans (AgNps-CN) with concentration ranged from 0.75 to 3 µg/mL in triplicate and then incubated for 24 h. 3–(4,5-Dimethylthiazol-2-yl)-2,5-diphenyltetrazolium bromide (MTT) was added to each well after 24 h treatment and incubation continued for 4 h. Formazan formation were dissolved using dimethyl sulphoxide (DMSO) that were added in each well. Optical density (OD) of the solution was determined using spectrophotometer at 570 nm wavelength. Experiments were repeated three times independently and means of OD and SD for each group were calculated. IC50 was determined based on the plot graph. The absorbance (OD) value was converted to percentage of cell viability by using the following formula:
Acridine orange/propidium iodide (AO/PI) double staining morphological analysis
Approximately 1 × 106 cells were seeded in 6-well plates and incubated for 24 h. Cells were then treated with various concentration and different incubation time range from 2–8 h. Cells were then trypsinized and washed once with 1 × PBS. A total of 10 µL of cell suspension was added on glass slide and mixed with 10 µL of AO (10 µg/mL) and PI (10 µg/mL). Cells were viewed under fluorescence microscope (Olympus, Germany).
Annexin V-FITC apoptotic assay
A total of 5 × 106 HSC-4 cell lines were seeded in 6-well plate and incubated for 24 h followed by treatment with AgNps-CN with different incubation time from 2–8 h. Apoptosis was detected by BD Annexin V-FITC Assay kit. Basically, cells were washed once with 1 × PBS, trypsinized and centrifuged. Cells suspended in binding buffer and stained with Annexin V and PI for few minutes. After that, samples were analysed with flow cytometer (Becton Dickinson). Viable cells (Annexin V-, PI-), early apoptotic (Annexin V+, PI-), late apoptosis (Annexin V+, PI+) and necrotic cells (Annexin V+, PI+) were expressed in percentage.
Cell cycle distribution analysis
HSC-4 cell lines were seeded in 6-well plate with cells volume of 5 × 106 per well and incubated for 24 h followed by treatment with AgNps-CN with different incubation time ranged from 2–8 h. Then, cell cycle distribution was carried out based on protocols provided by the manufacturers (Becton Dickinson). Briefly, cells were washed with 1 × PBS and then trypsinized for 2–3 min. Cells were then centrifuged for 5 min and washed twice with cold 1 × PBS. After that, 1 ml of binding buffer was added to the cells and then centrifuged. Trypsin buffer, trypsin inhibitor, RNase inhibitor and propidium iodide were added to cells and incubated for 10 min. Samples were then read with flow cytometer (Becton Dickinson). Data provided were analysed using Modifit software. Tests were repeated three times.
Western blot analysis
HSC-4 cells were seeded in 75 cm2 cell culture flask at 800,000 cells per flask with 15 ml of complete growth media and incubated for 24 h. After treatment for 2 h, the floating and adherent cells were washed twice with cold 1 × PBS. However, adherent cells were further lysed with 200 µL of lysis buffer. The flask was then kept on ice for 5 min. The lysates were gathered at one side using cell scraper, collected with 1 ml pipette, pooled with floating cells and centrifuged at 1000 rpm for 10 min to pellet the cell debris. The clarified supernatant was collected and stored at −80 °C. Bradford Protein Assay was done to measure protein concentration.
An equal amount of 10–20 µg of protein was separated by SDS-PAGE. After gel electrophoresis, the proteins were transferred to PVDF membrane by semidry method for 30 min, blocked with 3% TBS-T for 1 h and reacted with primary antibodies for 24 h in 4 °C. Finally, the primary antibodies were reacted with secondary antibodies for 1 h. Primary antibodies mouse anti-Bax (sc-4993), mouse anti-beta-actin (sc-47778) and mouse anti-Bcl-2 (sc-7382) and secondary antibodies of goat anti-mouse (sc-516102) were purchased from Santa Cruz Biotechnology (CA). Protein visualization was then performed using Chemi-Lumi One L purchased from Nacalai Tesque (Kyoto, Japan) and Chemi DocTM MP SYSTEM (Bio-Rad, Hercules, CA) in dark room. Protein levels was quantified using Image J software, normalized to beta-actin.
Statistical analysis
Results obtained were expressed as mean ± standard deviation (SD) of at least three independent experiment. Data were analysed with one-way ANOVA followed by Tukey’s post hoc test. Difference considered statistically significant at p ≤ .05.
Results
Characterization of silver nanoparticle
Particle size of silver nanoparticles ranged from 10 nm to 80 nm and shape of nanoparticles were spherical shape in aggregated form with zeta potential value of −42.8 mV [Citation19].
Cell viability assay
Cytotoxic activity of AgNps-CN against HSC-4 and 3T3-L1 cell lines were assessed using MTT assay. Based on , silver nanoparticle C. nutans showed cytotoxicity towards HSC-4 cell lines with IC50 of 1.61 ± 0.14 µg/mL. AgNps-CN were tested against 3T3-L1 to confirm that it is not cytotoxic towards normal cells and as shown in , there is no cytotoxic activity even at the highest concentration (3 µg/mL), cells viability is around 93.17 ± 1.43%. showed cytotoxicity activity of C. nutans crude extracts against HSC-4 cell lines. Crude extracts such as water, ethanol (100%, 70% and 50%), and chloroform had been used to treat the HSC-4 cell lines for 24 h with concentration ranged from 50 to 500 µg/mL. No IC50 can be determined for most of the C. nutans crude extracts.
Figure 1. Cytotoxic effects of AgNps-CN and AgNps blank towards HSC-4 cell lines and 3T3-L1 cell lines. Noted that there is no cytotoxic effects towards normal cell lines (3T3-L1) even at the highest concentration (3 µg/mL) while IC50 for HSC-4 cell lines is 1.61 ± 0.14 µg/mL whereas blank AgNps does not cause any cytotoxic activity towards HSC-4 cell lines. Data expressed as mean ± SD of three independent experiments.
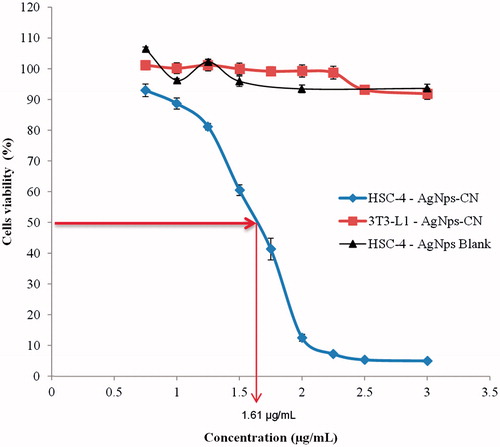
Table 1. Cytotoxic activity of C. nutans crude extracts aqueous, ethanol 50%, ethanol 70%, ethanol 100% and chloroform against HSC-4 cell lines. Cells viability were expressed in percentages.
AO/PI double staining morphological analysis
AO/PI double staining can distinguish stages of apoptosis and differentiate between viable, apoptosis and necrosis. Viable cells shows round and green nuclei and different stages of apoptosis will have different morphology. This can be seen in when cells were treated with 1–2 µg/mL of AgNps-CN for 2–8 h where different concentrations and incubations time cause changes in HSC-4 cells morphology. After 2 h of treatment with 1–2 µg/mL of AgNps-CN, cells start to show early apoptosis morphological characterization such as nuclear fragmentation, chromatin condensation and membrane blebbing. After 4 h of treatment with 2 µg/mL AgNps-CN, late apoptosis morphological characteristic such as bright orange chromatin can be seen. Similar observations were found when cells treated for 8 h with 1.5 µg/mL AgNps-CN.
Figure 2. Morphological study of HSC-4 cell lines after treated with different concentration of AgNps-CN with different treatment time. (a) HSC-4 cell lines after 2 h treatment time, (b) HSC-4 cell lines after 4-h treatment time, (c) HSC-4 cell lines after 8 h treatment time. Treated cells exhibited early and late apoptosis features such as nuclear fragmentation (NF), membrane blebbing (MB), chromatin condensation (CC) and bright orange chromatin (BOC).
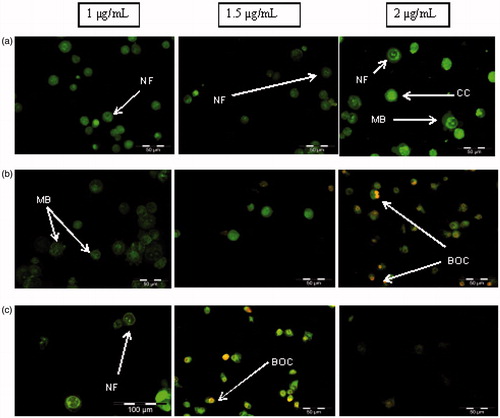
Annexin V-FITC apoptotic assay
This assay was done to understand the mechanism of cell death induced by silver nanoparticle C. nutans towards HSC-4 cell lines. Increased in treatment concentration intensified early and late apoptosis cell population. AgNps-CN actions towards HSC-4 cell lines were found to be concentration and time dependent. This can be seen in where increased concentration will cause cell population to move from viability to early apoptosis and then late apoptosis. Different incubation time also effects cell population in each phase. After 2 h of treatment, only 4.4% of cells entered early apoptosis in untreated cells but, 7.81% ± 1.21 (1 µg/mL), 21.43% ± 4.77 (1.5 µg/mL), 25.87% ± 3.77 (2 µg/mL), 37.59% ± 1.64 (3 µg/mL) entered early apoptosis stage. Increased treatment time caused cells to enter late apoptosis stage after 4 h of treatment, 58.93% ± 14.95 (1 µg/mL), 82.23% ± (1.5 µg/mL), 84.87% ± 1.34 (2 µg/mL), 89.16 ± 6.93 (3 µg/mL) cells entered late apoptosis stage.
Figure 3. Annexin V-PI flow cytometry analysis of HSC-4 cell lines treated with various concentration of AgNps-CN at 2–8 h of treatment time. Different stages of cells were shown such as viable cells, early apoptosis stage and late apoptosis stage. Data presented were expressed as mean ± SD. *p < .05 compared to untreated cells. Diagram (b), (c) and (d) represents flow cytometry data of HSC-4 cell lines when treated with 3 µg/mL of AgNps-CN at different incubation treatment time. (a) Untreated HSC-4 cell lines, (b) HSC-4 cell lines after 2 h treatment, (c) HSC-4 cell lines after 4 h treatment and (d) HSC-4 cell lines after 8 h treatment, (e) Analysis of Annexin V-PI after treated with 0 µg/mL, 1 µg/mL, 1.5 µg/mL, 2 µg/mL and 3 µg/mL of AgNps-CN for 2, 4 and 8 h.
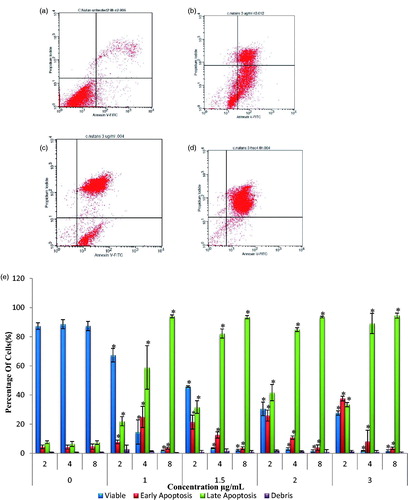
Cell cycle analysis
Cell cycle arrest has traditionally been known to closely associated with apoptosis. When there is DNA damage in cells, checkpoint in each phase of the cell cycle responsible to arrest the growth of the cells until the damage were repaired or if it cannot be repaired, cells will undergo apoptosis process. Therefore, this assay was done to understand whether apoptosis mechanism caused by AgNps-CN towards HSC-4 cell lines is by induction of cell cycle arrest or not, and which phase does AgNps-CN cause cell cycle arrest to occur.
Cells incubated with AgNps-CN for 2 to 4 h resulted in increased cells percentage at G1 phase. When treated with 1 µg/mL, there was significant increase (p < .05) in G1 phase’s cell population at 2- and 4-h incubation time from 58.63% ± 1.15 to 85.83% ± 2.68, respectively. The increment was accompanied by significant decrease (p < .05) in population of HSC-4 cells at G2 phase (8.99% to 0.33%) and S phase (32.38% to 13.8%). The same trend (increased in G1 phase population) was observed in HSC-4 cells treated with 1.5 µg/mL AgNps-CN with decreased in the population of G2 phase (3.72% to 0.07%) and S phase (18.23 to 8.15%). show cells percentage differences in each phase of cell cycles with different incubation time and concentration.
Figure 4. Cell cycle analysis of HSC-4 cells treated with 1–2 µg/mL at 2 and 4 h incubation time. Different phases of cell cycle which are G0/G1 phase, S phase and G2 phase. Noted that there is significant (p < .05) decrease in cells percentages in G2 and S phase after 4 h of AgNps-CN (1 µg/mL) of treatment compared to control groups. Most of the cells were arrested at G1 phase after 4 h of AgNps-CN treatment. Data were expressed as mean ± SD. *p < .05 when compared to untreated group.
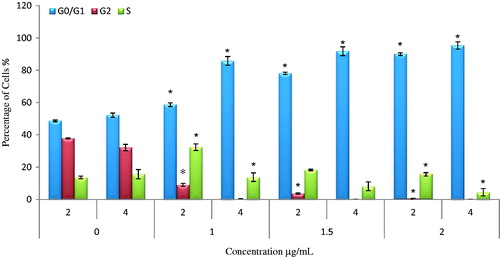
Western blot analysis
Bax/Bcl-2 protein ratio analysis was determined from western blot analysis to understand how these 2 proteins involved in induction of apoptosis. From , expression of Bax of HSC-4 cells treated with 1.5 and 2.0 µg/mL of AgNps-CN for 2 h were significantly up-regulated by 1.14- and 1.17-fold while expression of Bcl-2 protein were significantly down-regulated by nearly 2-fold and 5-fold when treated with 1.5 and 2.0 µg/mL of AgNps-CN. The ratio of Bax to Bcl-2 increased approximately 1.77- and 6.55-fold at 1.5 and 2.0 µg/mL, respectively, at 2 h.
Discussion
Green synthesis silver nanoparticles have drawn attention to many researchers. This may due to its rapid, environmental friendly and cost-effective properties. The crucial factor to produce silver nanoparticle through green synthesis methods is its silver ions which obtains from silver nitrate (AgNO3) and biological agents that act as reduction and stabilization agents. In this study, C. nutans was used as the biological agents. Appearances of reddish brown colour in silver nitrate solution indicated the formation of silver nanoparticles. This method has been widely used to determine the formation of silver nanoparticles. Many factors contribute to anticancer properties of silver nanoparticle especially the size of silver nanoparticle and capping agents involved [Citation20]. Particle size of silver nanoparticle synthesized in this study ranged from 10 to 80 nm with zeta potential at −42.8 mV [Citation19]. Heydari et al. (2015) reported high degree stability of silver nanoparticle was when zeta potential value is higher than 25 mV or less than −25 mV [Citation21]. This may suggest that silver nanoparticles formed has a high degree of stability. Other than that, strong repulsive force may be formed between the particles when there is high negative charge which may prevent particles aggregations.
AgNps-CN showed direct concentration-dependent relationship whereby cytotoxicity increased when concentration was increased. This can be seen in as increased in AgNps-CN concentration decreased percentage of cells viability. IC50 of AgNps-CN towards HSC-4 cell lines was 1.61 ± 0.14 µg/mL. Recently, Palaniselvam et al. (2015) suggested when HCT-116 treated with silver nanoparticles, percentage viability of cells affected and showed 50% cell death at 50 µg/mL [Citation7]. Study by Sankar et al., (2013) reported anticancer effects of silver nanoparticle against human lung cancer cell lines (A549) with 50% of cell death at 100 µg/mL [Citation22]. Various concentrations from 5 µg/mL-500 µg/mL of silver nanoparticle can be seen to exhibit cytotoxicity effects against various cultured cancer cells such as MCF-7, Hela, HepG2 and HT29 cell lines [Citation23,Citation24]. Therefore, AgNps in this study exhibited higher potential compared to other studies mentioned above with lower IC50 and 90% of cell death were found when treated at 3 µg/mL. Besides that, AgNps-CN also showed more cytotoxicity effects on other cell lines such as MCF-7 and HT29 compared to C. nutans extracts after 24 h [Citation9]. This may due to smaller particle size (<100 nm), which will act differently compared to bulk (>100 nm), because of differences in physiochemical properties and surface volume ratio. Improved cytotoxic effects of AgNps-CN compared to other AgNps may due to the presence of C. nutans bioactive compounds such as C-flavones glycosyl, stigmasterol, lupeol, β-sitosterol, belutin and myricyl alcohol that may act as capping agent in green synthesis of AgNps [Citation20,Citation25,Citation26].
AgNps-CN also showed better cytotoxic activity against HSC-4 cell lines compared to C. nutans crude extracts. Previous study showed C. nutans chloroform extract was cytotoxic to certain cancer cell lines such as human erythroleukemia cell lines (K-562), human Burkitt’s lymphoma cell lines (Raji), while C. nutans ethanol extract has been reported to induce apoptosis against liver cancer cell lines [Citation8,Citation9]. On the other hand, this study illustrated different finding where C. nutans showed no cytotoxic activity towards HSC-4 cell lines even though it has been treated at the highest concentration (500 µg/mL). Most plants crude extracts do not fall within the criteria set by America National Cancer Institute (IC50<20 µg/mL) [Citation8]. The different in the cytotoxic activity between C. nutans crude extracts and AgNps-CN may be due to different mechanism of action. It has been reported antitumor activity of C. nutans ethanol crude extract is due to up-regulating the immune response. It has been reported C. nutans increased the number of IFN- γ+ T cells and decreased in interleukin 4 (IL-4+) T cells which cause indirect antitumor activity while AgNps exhibited more direct activity which increased ROS, mitochondrial membrane permeability, DNA damage and disrupt DNA function which lead to cell cycle arrest and apoptosis [Citation9].
showed cytotoxicity comparison between AgNps-CN and commercial AgNps (blank AgNps). At the highest concentration tested (3 µg/mL), percentage viability of HSC-4 cell lines when treated with AgNps-CN is much lower compared to blank AgNps. This finding suggested lower concentration of AgNps-CN was required to inhibit HSC-4 cell lines growth as compared to blank AgNps. The same finding was found when AgNps-CN was treated on normal cells (3T3-L1). Based on , at the highest concentration of AgNps-CN (3 µg/mL), percentage viability of HSC-4 cell lines was much lower than 3T3-L1 cell lines (5.03% and 91.85%, respectively). This finding showed AgNps-CN caused no cytotoxic effect towards 3T3-L1 cell lines. This study was in concordance with study done by Singh et al., (2017) which reported IC50 of AgNps synthesis by Panax ginseng leaves towards lung cancer cell lines (A549) and melanoma cancer cell lines (B16BL6) was 2 µg/mL and 100 µg/mL, respectively. This study also found their AgNps did not cause cytotoxicity towards 3T3-L1 cell lines [Citation27]. Based on these results, the rest of parameters were performed only on cancer cells (HSC-4 cell lines).AgNps were said to possess anticancer properties via apoptosis and increased in reactive oxygen species. Therefore, apoptosis assay such as double staining with acridine orange/propium iodide (AO/PI) and Annexin V-FITC were done to study the mode of cell death for OSCC when treated with AgNps. In AO/PI double staining, both dyes entered DNA with difference in appearance of colour and PI were taken up by non-viable cells. Viable cells will have bright coloured nuclei mainly due to AO. In early apoptosis, cells exhibited few morphological changes such as blebbing of plasma membrane, nucleus condensation and colour appearance. This can be seen in whereby cells in early apoptosis stage exhibited membrane blebbing and chromatin condensation with bright coloured nuclei. Late apoptosis stage can be differentiated with necrotic stage based on cells appearance. In late apoptosis stage, cells appeared as bright orange at the area of condensed chromatin while in necrotic stage, cells projected uniform orange colour. This can be seen after cells treated with AgNps-CN for 4 h. This proves that AgNps induced apoptosis towards HSC-4 cell lines, not necrosis. During apoptosis, cellular content does not leak out due to intact plasma membrane, therefore did not caused inflammation at nearby cells and tissues. Different condition was observed with necrotic cells as plasma content leaked due to membrane disrupted and lead to inflammation to nearby cells and tissue. Therefore, apoptosis is more preferable compared to necrosis since various treatments for cancer such as chemotherapy drugs targeted to induce apoptosis towards cancer cells.
Annexin V is Ca2+ dependent phospholipid-binding protein that has high affinity for phosphatidylserine (PS). Losing in plasma membrane asymmetry in early stage of apoptosis will cause translocation of PS to outer leaflet of plasma membrane which exposed it to Annexin V. Binding of Annexin V conjugated with FITC allow this assay to detect early apoptosis features. Based on , treatment with AgNps at different incubation time and concentration induced apoptosis towards HSC-4 cell lines as the cells population changes from viable to early apoptosis and finally to late apoptosis stage. This can be seen when HSC-4 cell lines were treated with 1 µg/mL AgNps-CN. After 2 h of treatment, the percentage of viable cells were 67.27% with 7.81% of cells in early apoptosis stage. After 4 h, the number of cells in viable stage decreased significantly (14.61%) with the number of cells in early apoptosis stage increased significantly (25%) while the cells at late apoptosis stage is 58.93%. After 8 h of treatment, the difference can be seen when the number of viable cells only left with 1.99%, while 3.54% cells still in early apoptosis stage and 94% of cells already entered late apoptosis or secondary necrosis stage. Changes in stages of cells from viable to early and finally to late apoptosis or secondary necrosis proved AgNps-CN induced apoptosis towards HSC-4 cell lines not necrosis or any other mode of cell death. Same finding was reported by Mousavi et al., (2018) whereby IC50 of AgNps-Artemisia turcomanica leaves increased early and late apoptosis stage and at the same time decreased the percentage of viable cells from 92.26% to 51.16% when compared to untreated cells [Citation28].
Apoptosis and cell cycle arrest has a very close relationship as apoptosis usually occurs when cells proliferation has been arrested at certain cell cycle checkpoints [Citation11]. Checkpoints involved were G1/S phase, S phase and finally at G2/M phase. At each of the checkpoint, it will determine whether the cells can continue to complete cell division or arrest growth of the cells to repair cellular damage. If the cellular damage cannot be repaired, the cells will undergo apoptosis. There are two major protein families that are involved in each of the checkpoint which are cyclin-dependent protein kinases (CDKs) and cyclins. CDKs function is to allow cell to progress or get through cell cycles. Each checkpoint have different CDKs and cyclins involved. For example, in G1 phase, proteins involved were CDK-4 and CDK-6 [Citation29]. From G1 to S phase, cyclin E will activate CDK 2 and cause cyclin A to bind with CDK 2 at S phase. Previous study by Uchida et al. (2012) reported progression of OSCC may be due to overexpression of CDCA3 during oral carcinogenesis that caused decreased in expression of cyclin dependent kinase inhibitor which lead to prevention of the cell cycle growth arrest at G1 phase [Citation30]. From this cell cycle analysis, AgNps-CN induced apoptosis through G1 cell cycle arrest. Based on , there was increased in cells percentage in G1 phase after treated with AgNps-CN compared to control group. Increased in treatment incubation period also lead to increase in cells percentage in G1 phase. Percentage of cells in S and G2 phase was significantly decreased (p < .05) after 4 h treatment at 1 µg/mL AgNps-CN compared to control groups. Therefore, it can be hypothesized that growth of cells were arrested at G1 phase and lead to apoptosis. This study was in concordance with study done by Prabhu et al. (2013), which reported AgNps caused colon cancer cells (HCT-15) growth arrested at G0/G1 phase after treated with 20 µg/mL at 48 h incubation time [Citation23]. Study done by Palaniselvam et al. (2015) reported AgNps inhibit growth of colon cancer cells (HCT-116) and increased in proportion of Sub-G1 phase in all treated samples compared to control group [Citation7].
Bcl-2 family members which consists of pro- and anti-apoptotic proteins have long been reported to play a pivotal role in regulation of apoptosis [Citation31]. As most of the Bcl-2 family members located at the outer mitochondria membrane, they are especially important in apoptosis intrinsic pathway. Pro-apoptotic protein usually results in increase of mitochodrial permeability which lead to release of pro-apoptotic molecules such as cytochrome-C which cause activation a series of caspase which lead to cell undergoes apoptosis and vice versa for anti-apoptotic proteins. It is not the absolute quantity but rather ratio of pro- and anti-apoptotic protein that plays an important role in regulation of cell death and cell viability. When there is disruption in the balance of these two groups of proteins, it will cause dysregulation of apoptosis in the affected cells. In the present study, it was found that AgNps-CN significantly increased (p < .05) the ratio of Bax/Bcl-2 of HSC-4 cell lines. Increased in the ratio mainly due to down-regulated of Bcl-2 protein with almost 5-fold after treated with 2 µg/mL AgNps-CN for 2 h. This suggested AgNps-CN induced apoptosis towards HSC-4 cell lines through intrinsic pathway by suppressing the release of Bcl-2 protein that leads to increase permeability of mitochondrial membrane. Further study with other pro- and anti-apoptotic protein that involve in extrinsic pathway of apoptosis may be needed to understand the complete mechanism of HSC-4 cell lines when treated by AgNps-CN.
Conclusions
AgNps-CN have shown significant cytotoxic effects against HSC-4 cell lines. Besides that, the presence of apoptotic features such as membrane blebbing, nuclear fragmentation, chromatin condensation and increase in the ratio of Bax/Bcl-2 protein conclude that AgNps-CN induced apoptosis via intrinsic pathway against HSC-4 cell lines. Further study such as in vivo assay may be needed to further confirm anti oral cancer effects of AgNps-CN.
Acknowledgement
The authors would like to thank Universiti Putra Malaysia (UPM) and Universiti Teknologi MARA (UiTM) for providing the laboratory facilities.
Disclosure statement
The authors declare no conflict of interests.
Additional information
Funding
References
- Sharma VK, Yngard RA, Lin Y. Silver nanoparticles: green synthesis and their antimicrobial activities. Adv Colloid Interf Sci. 2009; 145:83–96.
- Sun Q, Cai X, Li J, et al. Green synthesis of silver nanoparticles using tea leaf extract and evaluation of their stability and antibacterial activity. Colloids Surf A Physicochem Eng Asp. 2014;444: 226–231.
- Singh A, Jain D, Upadhyay M, et al. Green synthesis of silver nanoparticles using argemone mexicana leaf extract and evaluation of their antimicrobial activities. Dig J Nanomater Bios. 2010;5: 483–489.
- Bar H, Bhui DK, Sahoo GP, et al. Green synthesis of silver nanoparticles using seed extract of Jatropha curcas. Colloids Surf A Physicochem Eng Asp. 2009;348:212–216.
- Verma A, Mehata MS. Controllable synthesis of silver nanoparticles using Neem leaves and their antimicrobial activity. J Radiat Res Appl Sci. 2016;9:109–115.
- Ahmed S, Ahmad M, Swami BL, et al. A review on plants extract mediated synthesis of silver nanoparticles for antimicrobial applications: a green expertise. J Adv Res. 2016;7:17–28.
- Kuppusamy P, Ichwan SJ, Al-Zikri PNH, et al. In vitro anticancer activity of Au, Ag nanoparticles synthesized using Commelina nudiflora L. aqueous extract against HCT-116 colon cancer cells. Biol Trace Elem Res. 2016;173:297–305.
- Yong YK, Tan JJ, Teh SS, et al. Clinacanthus nutans extracts are antioxidant with antiproliferative effect on cultured human cancer cell lines. Evidence-Based Complement Altern Med. 2013;2013:462751.
- Huang D, Guo W, Gao J, et al. Clinacanthus nutans (Burm. f.) Lindau ethanol extract inhibits hepatoma in mice through upregulation of the immune response. Molecules. 2015;20: 17405–17428.
- Wong RS. Apoptosis in cancer: from pathogenesis to treatment. J Exp Clin Cancer Res. 2011;30:87.
- Hassan M, Watari H, AbuAlmaaty A, et al. Apoptosis and molecular targeting therapy in cancer. BioMed Res Int. 2014;2014:150845.
- Lowe SW, Lin AW. Apoptosis in cancer. Carcinogenesis. 2000;21: 485–495.
- Mehrotra R, Yadav S. Oral squamous cell carcinoma: etiology, pathogenesis and prognostic value of genomic alterations. Indian J Cancer. 2006;43:60.
- Tsai LL, Yu CC, Chang YC, et al. Markedly increased Oct4 and Nanog expression correlates with cisplatin resistance in oral squamous cell carcinoma. J Oral Pathol Med. 2011;40:621–628.
- Hsu S, Singh B, Schuster G. Induction of apoptosis in oral cancer cells: agents and mechanisms for potential therapy and prevention. Oral Oncol. 2004;40:461–473.
- Andreadis C, Vahtsevanos K, Sidiras T, et al. 5-Fluorouracil and cisplatin in the treatment of advanced oral cancer. Oral Oncol. 2003;39:380–385.
- Tong D, Poot M, Hu D, et al. 5-Fluorouracil-induced apoptosis in cultured oral cancer cells. Oral Oncol. 2000;36:236–241.
- Lee YR, Wu WC, Ji WT, et al. Reversine suppresses oral squamous cell carcinoma via cell cycle arrest and concomitantly apoptosis and autophagy. J Biomed Sci. 2012;19:9.
- Lim V, Che Mood CNA, Samsurrijal SF, et al. Green synthesis of silver nanoparticles from leaf and stem extracts of Clinacanthus Nutans: characterisation, antimicrobial and cytotoxicity studies. Paper Presented at: World Congress and Expo on Materials Science and Polymer Engineering 2015; Nov 28–30; Dubai.
- Srikar SK, Giri DD, Pal DB, et al. Green synthesis of silver nanoparticles: a review. GSC. 2016;6:34.
- Heydari R, Rashidipour M. Green synthesis of silver nanoparticles using extract of oak fruit hull (Jaft): synthesis and in vitro cytotoxic effect on MCF-7 cells. Int J Breast Cancer. 2015;2015:846743.
- Sankar R, Karthik A, Prabu A, et al. Origanum vulgare mediated biosynthesis of silver nanoparticles for its antibacterial and anticancer activity. Colloids Surf B Biointerfaces. 2013;108:80–84.
- Parveen A, Rao S. Cytotoxicity and genotoxicity of biosynthesized gold and silver nanoparticles on human cancer cell lines. J Clust Sci. 2015;26:775–788.
- Lalitha P. Apoptotic efficacy of biogenic silver nanoparticles on human breast cancer MCF-7 cell lines. Prog Biomaterials. 2015;4: 113–121.
- Sakdarat S, Shuyprom A, Pientong C, et al. Bioactive constituents from the leaves of Clinacanthus nutans Lindau. Bioorg Med Chem. 2009;17:1857–1860.
- Alam A, Ferdosh S, Ghafoor K, et al. Clinacanthus nutans: a review of the medicinal uses, pharmacology and phytochemistry. Asian Pac J Trop Med. 2016;9:402–409.
- Singh P, Singh H, Ahn S, et al. Pharmacological importance, characterization and applications of gold and silver nanoparticles synthesized by Panax ginseng fresh leaves. Artif Cells Nanomed Biotechnol. 2017;45:1415–1424.
- Mousavi B, Tafvizi F, Zaker Bostanabad S. Green synthesis of silver nanoparticles using Artemisia turcomanica leaf extract and the study of anti-cancer effect and apoptosis induction on gastric cancer cell line (AGS). Artif Cells Nanomed Biotechnol. 2018 [cited Jan 23]. DOI:10.1080/21691401.2018.1430697
- Meyerson M, Harlow E. Identification of G1 kinase activity for CDK6, a novel cyclin D partner. Mol Cell Biol. 1994;14:2077–2086.
- Uchida D, Kawamata H, Omotehara F, et al. Role of HGF/c‐met system in invasion and metastasis of oral squamous cell carcinoma cells in vitro and its clinical significance. Int J Cancer. 2001;93:489–496.
- Foo JB, Yazan LS, Tor YS, et al. Induction of cell cycle arrest and apoptosis by betulinic acid-rich fraction from Dillenia suffruticosa root in MCF-7 cells involved p53/p21 and mitochondrial signalling pathway. J Ethnopharmacol. 2015;166:270–278.