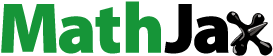
Abstract
Intra-articular injection of anti-inflammatory drugs can be a promising strategy for recovery of injured articular cartilage. We prepared a series of injectable thermo-sensitive composite hydrogels, composed of Pluronic F127, glycosaminoglycan (GAG) and bone morphogenetic protein (BMP-2), which was designed to mimic extracellular matrix (ECM). The rheological properties and dissolution rate of composite hydrogels containing chondroitin sulfate or with different hyaluronic acid molecular mass (10k, 90k, 800k) were investigated. Meanwhile, bovine serum albumin (BSA) or FITC-BSA was chosen as model drug loaded into PF/GAG hydrogels to study their sustained release behavior in vitro. The results showed that hydrogels could maintain shapes for more than 16 days and the release rate of BSA in PF/GAG composite gels was much slower than in PF127 gels, due to the affinity between BSA and GAG. Furthermore, increasing the molecular weight of hyaluronic acid correspondingly increased hydrogel dissolution rate and BSA release in the hydrogels. Subsequently, MTT experiments were performed to investigate the toxicity of the hydrogels on mouse pre-osteoblast cell MC3T3-E1. In vivo anti-inflammation results showed that PF/GAG@BMP-2 composite hydrogels had the most efficient efficacy on recovery of injured cartilage, which is induced by osteoarthritis, compared to the control groups (PF127@BMP-2 or BMP-2 saline solution).
Introduction
Osteoarthritis (OA) is a type of joint disease that results from breakdown of joint cartilage, synovial inflammation and subchondral bone sclerosis, which caused pain, complications and disability [Citation1,Citation2]. Current treatment of OA is mainly on administration of anti-inflammatory drugs, topical anti-inflammatory hydrogel, intra-articular injection of hyaluronic acid and surgical treatment for patients with severe conditions [Citation3]. However, local administration of drugs is unable to achieve long-term effect and topical hydrogels are not suitable for protein drugs. In recent years, injectable hydrogels have been widely investigated due to their properties of in situ sustained release of encapsulated drugs, improved stability and intensive adhesion for prolonged retention [Citation4–6]. Among them, thermo-sensitive in situ gels, composed of cells, anti-inflammatory drugs and growth factors, which could be injected into inflammation sites as solutions and converted to gels rapidly inside the body, has become one of the most promising hydrogels for tissue engineering, especially in bone regeneration [Citation7]. Pluronic F127, a FDA approved synthetic polymer of poly (propylene oxide) (PPO) blocks flanked by poly (ethylene oxide) (PEO) blocks, has been widely used in thermo-sensitive in situ gels [Citation5,Citation8]. The thermos-reversible property makes Pluronic F127 possible to convert its solution state to the gelation state when reaching the critical gelation temperature. However, there are still some drawbacks, which hindered their clinical use, such as burst rapid releasing and lower strength of hydrogel [Citation9], mainly due to the rapid diffusion rate of drugs in the hydrogel. On the basis, we hypothesized that if the affinity of the drugs with the gel matrix could be improved, the diffusion and dissolution of the drug from the gel matrix may be effectively delayed, thereby inhibiting the drug burst release and prolonging the release time.
Extracellular matrix (ECM) is a network structure composed of protein and polysaccharide secreted by cells, and almost all proteins exist in ECM. Cells, proteins and ECM are in a dynamic environment, and the multiple interactions among them constitute the microenvironment of cell survival in vivo. Proteins are immobilized in the extracellular matrix due to the affinity with glycosaminoglycan (such as hyaluronic acid (HA), chondroitin sulfate (CS) and heparin). The ECM seems to be a natural drug sustained release system for proteins. Yang [Citation10] have shown that the interaction between proteins and glycosaminoglycan (GAG) is mainly mediated by the charge adsorption between the amino group on the protein and the sulfonic acid or carboxyl group on the glycosaminoglycan molecule. The binding of GAG and proteins can effectively regulate the release rate of proteins and prevent proteolytic degradation of proteins in tissue fluids [Citation11]. HA is a major component of ECM in many tissues, which plays a crucial role in the development of cartilage and the maintenance of synovial fluid due to its biological properties such as non-inflammatory, biocompatibility and biodegradability [Citation12,Citation13]. Moreover, HA can provide cells with a good cartilage-forming microenvironment that helps maintain the chondrocyte phenotype due to its hydrophilic [Citation14,Citation15]. CS is sulfated glycosaminoglycan, which is found in natural cartilage and other tissues. CS has prominent features including anti-inflammatory activity, inhibition of production of cartilage degradation enzyme and a biological activity at the cellular level, making it play a pivotal role in tissue remodeling. Particularly, CS and HA have property of water retention to cartilage due to their negatively charged structure [Citation12,Citation16].
Based on the hypothesis, in this study, we developed an intra-articular injection thermo-sensitive gel delivery system, which bone morphogenetic protein 2 (BMP-2) and glycosaminoglycan molecules (HA or CS) were encapsulated in it for the application as repair of cartilage damage in OA (). In bone regeneration studies, BMP-2 is one of the most promising growth factor that plays a pivotal role in bone healing process [Citation17,Citation18]. The appropriate concentration of PF127 is chosen by determining the gelation temperature and syringeability. HA and CS were introduced in the thermo-sensitive gel respectively by physical blend, and the rheological characteristics including gelation temperature and gelation time were determined. To investigate the sustained release behavior of protein drugs in composite thermo-sensitive gel and to confirm the affinity between protein drugs and glycosaminoglycan, bovine serum albumin (BSA) was chosen as model drugs. The compatibility of PF/GAG composite gels to pre-osteoblast cells was measured by MTT assay and cell proliferation experiment. Furthermore, in vivo anti-inflammation effects of the PF/GAG@BMP-2 in OA-mode rats were investigated.
Materials and methods
Materials
Pluronic®F127 (PF127) was purchased from Sigma-Aldrich (St. Louis, MO, USA). Hyaluronic acid (10k, 90k, 800k) was purchased from Meilunbio® (Dalian, China). Chondroitin sulfate was purchased from Solarbio® (Beijing, China). BMP-2 was purchased from Peprotech® (Rocky Hill, NJ, USA). BCA (Bicinchoninic acid) protein assay kit was purchased from Boster Biological Technology Co Ltd (Wuhan, China). Dulbecco’s modified Eagle’s medium (DMEM) was purchased from HyClone Inc (Logan, UT, USA). Fetal bovine serum (FBS) was purchased from Cellmax (Beijing, China).
Preparation of PF/GAG@BSA hydrogels
PF/GAG composite hydrogels were prepared according to a previously reported cold method [Citation19,Citation20]. Briefly, 2 g PF127 and 0.05 g GAG for each formulation was added into 7.95 g sterilized ultrapure water and stirred overnight at 4 °C until the homogeneous and clear solution is obtained. PF/GAG@BSA composite hydrogel was obtained by adding certain concentration of BSA solution into prepared PF/GAG solutions to a final concentration of 20 mg/g.
Characterization
Rheological properties
The gelatinization temperature of PF/GAG hydrogels were determined by rotational rheometer (Anton Paar MCR301, Graz, Austria). A coaxial cylindrical mold was used to scan the rheological parameters of different concentrations of PF127 thermo-sensitive hydrogels and different PF/GAG thermo-sensitive hydrogels under dynamic oscillation mode. The strain amplitude was 1%, the frequency was 1 Hz, the heating rate was 1 °C/min, and the scanning temperature range from 15 to 40 °C. The storage modulus (G′), loss modulus (G′′) and the complex viscosity (η) were taken as the observation index.
Gelation time
The vials with PF/GAG thermo-sensitive hydrogels were removed from 4 °C and then placed in a water bath at 37 °C rapidly. The time that hydrogels was no longer flowing after inverting the vials was recorded as the gelation time. The gelation time of different PF/GAG composite gels was compared.
Dissolution of hydrogels
The gel dissolution experiment was carried out to investigate how long the hydrogels could maintain their shapes for long-term drug release. The membrane-less model was used as described previously [Citation17]. A total of 3 g PF/GAG solution was transferred into tube at 4 °C. The height of hydrogel (H0) was determined from bottom to top of hydrogel in tube after gelation at 37 °C. Then, 5 ml of release medium (PBS, pH 7.4) pre-equilibrated at 37 °C was gently laid over the surface of the gel. The experiment was carried out in constant temperature shaking table (100 r/min, 37 °C). At predetermined times, the height of hydrogel (HT) was determined. Dissolution rate (DR) of hydrogels was determined as the following rate:
H0 = Initial height of hydrogels.
HT = Height of hydrogels at predetermined times.
The obtained results represent the average of three comparable experiments for each sample.
In vitro BSA release from BSA/PF/GAG hydrogels
BSA is widely used in tissue engineered material as a common kind of model protein [Citation21,Citation22]. In this study, BSA was selected as a model protein to determine its release rate in PF/GAG composite hydrogels. In brief, a total of 3 g BSA-loaded (20 mg/g) PF/GAG solution was transferred into tube and placed in water bath at 37 °C until the gel was formed. After gelation, 5 ml of release medium (PBS, pH 7.4) pre-equilibrated at 37 °C was gently laid over the surface of the gel. The experiment was carried out in constant temperature shaking table (100 r/min, 37 °C). At predetermined time intervals, a certain amount of release medium was taken out for determination by BCA protein quantitative kit and meanwhile an equal volume of release medium was added into the tube. Release rate (%) = The cumulative content of BSA in the release medium/The total content of BSA in the composite hydrogel.
Cell study
MTT assay
Mouse pre-osteoblast cell line MC3T3-E1 was cultured in Dulbecco’s Modified Eagle’s Medium (DMEM) containing l-glutamine and nonessential amino acids supplemented with 10% fetal bovine serum (FBS), 100 U/mL (1%) streptomycin/penicillin and incubated at 37 °C in a 5% CO2 at 95% humidity. The extracts of hydrogels were made in cell culture medium using the ratio of 1.25 cm2/mL between the surface area of the samples and the volume of medium and incubated at 37 °C for 24 h according to the ISO Standard 10993–12 [Citation23]. Undiluted extracts were used for the MTT assay. MC3T3 cells were seeded in 96-well plate at the density of 1 × 104 cells/well in prepared cell culture medium. After culturing for 12 h in the cell incubator with 5% CO2 at 37 °C, former cell culture medium in 96-well plate was replaced with the prepared hydrogel extract medium. After culturing for 24 h, 20 μL MTT stock solution (5 mg/ml) was added in each well. After incubation for 4 h, the medium in each well was removed and 150 μL DMSO solution was added into each well to dissolve the formazan blue crystals. The optical density (OD) value at 492 nm can be read by the microplate reader. Cell viability (%) = Asample/Acontrol × 100%, where Asample and Acontrol are the absorbance values for the treated cells and the control cells, respectively.
Proliferation of MC3T3 cells on PF/GAG hydrogels
One millilitre of gamma irradiation-sterilized PF/GAG solution with gelation in a 48-well plate at 37 °C. MC3T3 cells were seeded gently onto the gel in 48-well plate at the density of 1 × 104 cells/well in prepared cell culture medium. Gels with encapsulated cells were cultured for 4 days. After washed with phosphate-buffered saline (PBS), samples were visualized with fluorescence microscope equipped with a digital camera. The proliferation properties and morphologies of cell growth in PF/GAG gels were imaged using laser confocal microscopy (Nikon A1R MP, Tokyo, Japan).
In vivo anti-inflammation study
Induction of osteoarthritis
Twenty-four male rats (230 g ± 20 g, 8 weeks) were used for the experiment following internationally accredited guidelines with ethics approval from the Institutional Animal Care and Use Committee of College of Pharmacy in Qingdao University.
The rats were randomly divided into 8 groups: control group (CG), OA group (OAG); OA + BMP-2 positive control group (BG); OA + PF127@BMP-2 group (BPG); OA + PF/HA10@BMP-2 group (BPHG1); OA + PF/HA90@BMP-2 group (BPHG2); OA + PF/HA800@BMP-2 group (BPHG3); OA + PF/CS@BMP-2 group (BPCG). OA was induced by injection of 50 μl of 3 mg sodium iodoacetate (MIA) through the supra-patellar ligament and into the bilateral knee joint cavity in left leg of rats with 29 G needle [Citation24]. Rats injected with an equivalent volume of saline were the control group.
Joint histological analysis
One microlitre of BMP-2 solution (1 μg/μl) was added into 50 μl of composite thermo-sensitive gels to a final concentration of 20 mg/ml. One week after induction of osteoarthritis, PF/GAG@BMP-2 composite gels were injected into left knee of rats.
Three weeks after administration of BMP-2/PF/GAG hydrogels, rats were sacrificed by intra-peritoneal injection of excess chloral hydrate. The entire knee joints including the articular capsule were fixed with 10% paraformaldehyde at 4 °C for 48 h. Then they were decalcified in EDTA decalcification solution for over 2 months and the solution was changed every 4 days. After a series of concentration of ethanol dehydration, tissues were embedded in paraffin. Standardized 5 μm sagittal sections were cut from the joint for histological analysis. The sections were stained with Safranin-O/Fast Green via standard procedures. The severity of cartilage degradation in femur and tibia was assessed by the Modified Mankin score system [Citation25]. The evaluation parameters were as follows: (1) cartilage structure (0–6), (2) cartilage cells (0–3), (3) Safranin-O/Fast Green staining (0–4).
Statistical analysis
Data was expressed as mean ± standard deviation. Statistical analysis was performed by Students’ t-test with statistical significance assigned for p values of <.05.
Results and discussions
Synthesis and characterization of PF/GAG
Rheological properties
Rheological properties [Citation26] play a critical role in the process of injectable hydrogel in vitro and in vivo. In order to determine an appropriate concentration for viscosity, strength and gelation temperature [Citation9,Citation27] of injectable thermo-sensitive hydrogels, the complex viscosity (η) of hydrogels with different concentrations of PF127 and GAG were investigated. The changes of complex viscosity (η) PF127 with the increase of temperature were shown in . Before the gel was formed, the viscosity of the solution increased obviously with the temperature raised. When the gel was formed, the viscosity increased sharply and then tended to be constant gradually. The gelation temperature (Tgel) was described as the temperature at which the storage modulus (G′) and loss modulus (G′′) crossover occurred in the process of heating up. As shown in , the gelation temperature of 20% PF127 thermo-gel was 25 °C, and the viscosity (η) increased sharply at this temperature in which storage modulus (G′) and loss modulus (G′′) crossover occurred. Therefore, the temperature in which sudden surge of viscosity occurred was also considered as the Tgel in this study. As shown in and , with the increase of the concentration of PF127 solution, the Tgel was decreasing while the viscosity was increasing. When the concentration was less than 16%, PF127 solution does not have the gelation characteristics. Besides, appropriate viscosity of composite hydrogels should be selected for satisfactory syringe-ability, and the strength should be strong enough to maintain its shape to delay drug release in situ. When the concentration reached higher than 22%, the viscosity of PF127 solution was already not suitable for injection. The Tgel of 18% PF127 was 30 °C, which was closer to the temperature of human body. However, the time for stage changed from solution state to gel state at body temperature was also depended on the concentration of PF127. Increasing the concentration of PF127 would effectively avoid the drug burst caused by the unprompted transformation of gel state from solution state at the injection site. Therefore, 20% PF127 was chosen to apply in this study. The Tgel of 20% PF127 was 25 °C, which ensured that the gel had the maximum viscosity and strength on the premise of good needle penetration in solution state.
Figure 1. (A) The complex viscosity (η′) of PF127 at different concentrations (17%, 18%, 20%, 22% and 25%, respectively) altered with a temperature increase. (B) The total viscoelasticity including the storage modulus (G′), the loss modulus (G′′) and the viscosity (η) of 20% PF127 thermo-sensitive hydrogel. (C–D). The complex viscosity (η′) of composite gel (introduction of (C) hyaluronic acid with different molecular weights and (D) a variety of glycosaminoglycan) with a temperature increase.
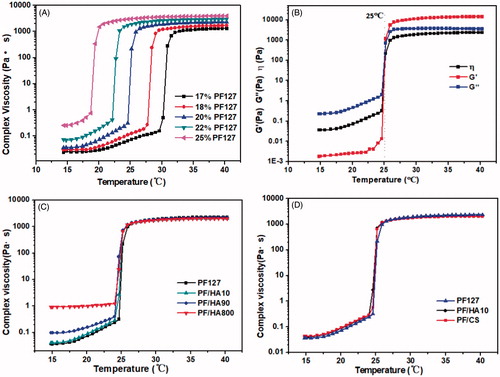
Table 1. The trend of Tgel with the change of concentration of PF127.
After the concentration of PF127 was determined, different glycosaminoglycan (GAG) molecules (0.5%, 1.0% and 1.5% (w/w)) were introduced in the composite gel, respectively. The results showed that only 0.5% had good syringe-ability which increased the concentration of GAG, andthe viscosity of hydrogel was too high for injection.
As shown in , introduction of hyaluronic acid with different molecular weights and CS has less effect on Tgel of the composite gel. Only in the solution state, the viscosity of the composite gel increased gradually with the increase of the molecular weight of hyaluronic acid. After the gel formation, their viscosity and strength tendency were similar to PF127 gel. It should be noted that the introduction of hyaluronic acid with molecular weight of 10 K and chondroitin sulfate in composite gel has similar rheological properties, including Tgel and viscosity (). The results indicated that the presence of GAG (hyaluronic acid and chondroitin sulfate) in PF127 hydrogel could enhance the viscosity of the composite hydrogel in solution state for injection.
Gelation time
Gelation time also plays an important role in the design of the injectable composite hydrogels. Hydrogels should form slowly enough so that cells and biomolecules can be mixed homogenously inside it, and they should remain fluid during injections and surgical procedures. However, once injected, they should gel quickly so that they do not leave the injection site [Citation15,Citation28]. As shown in , the gelation time of all PF/GAG composite hydrogels were controlled within 90 s. Compared with PF127 gels, the gelation time of PF/GAG was much longer and by increasing the molecular weight of hyaluronic acid, the gelation time of the resulted PF/HA has correspondingly increased.
Table 2. The gelation time of different formulations by introduction of a variety of glycosaminoglycan in PF127 gel.
Dissolution rate
The fast solubility and the long-term residue maintaining site applied in vivo are the most critical problems [Citation29]. Accordingly, appropriate viscosity and strength of composite hydrogels are required to maintain the gel state. The membrane-less model was used to simulate the in vivo condition. The dissolution behavior of PF127 and PF/GAG composite gels with various molecular weight of HA was shown in . As shown, by increasing the molecular weight of hyaluronic acid correspondingly increased the dissolution of the gel (57.7% for PF/HA10, 61.5% for PF/HA90 and 66.1% for PF/HA800). The dissolution rate of PF/CS was 60.1%, which was between PF/HA10 and PF/HA90. The PF127 gel without GAG remained the slowest dissolution rate (50.7%). There were no significant differences in dissolution rate among the PF/GAG hydrogels before 9 days (37.7% for PF127, 38.5% for PF/HA10, 40.9% for PF/HA90, 42.3% for PF/HA800 and 39.8% for PF/CS). The dissolution rate of PF/GAG increased sharply probably due to the structure of GAG that accelerated the erosion of water molecules to three-dimensional network of gel’s structure.
In vitro release behavior of PF/GAG@BSA
To evaluate the in vitro release of PF/GAG composite hydrogel, BSA was chosen as model drug, which was loaded into PF/GAG to form PF/GAG@BSA. The in vitro release rate of BSA from PF/GAG@BSA was shown in . During the first three days, the release rate of BSA in PF127 gel (15.9%) was faster than that in PF/HA composite gels (12.4% for PF/HA10, 13.9% for PF/HA90 and 15.0% for PF/HA800). However, with the release time increased, the release rate of BSA in PF/HA gradually accelerated, while the release rate remained constant in PF127 gel. After 16 d release, the release rate of BSA in PF/HA composite gels (38.4% for PF/HA10, 42.5% for PF/HA90 and 46.5% for PF/HA800) was higher than that in PF127 gel (36.3%). Furthermore, increasing the molecular weight of hyaluronic acid correspondingly increased BSA release in the hydrogel. Similarly, the initial release rate of BSA in PF/CS composite gel was slower (13.2%), but as time increased, the final rate (35.0%) was higher than PF127 gel (36.3%) (). It may be due to the affinity between the BSA and glycosaminoglycan, allowing the BSA to release more slowly in PF/GAG. However, the dissolution of gel gradually dominated the release rate and the hydrophilicity of GAG aggravated the dissolution of gel. Ultimately, the higher dissolution rate led to higher BSA release rate of the gel.
Figure 3. Comparison of release rate of BSA in PF/GAG composite gels (introduction of (A) hyaluronic acid with different molecular weights and (B) a variety of glycosaminoglycan). (C) In vitro release property of FITC-BSA in PF/GAG composite gels using molecular imaging. (D) Comparison of fluorescence intensity in each sample at various time points.
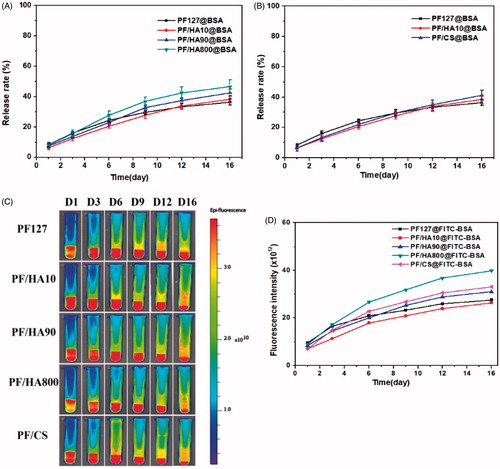
To further explore the release behavior of BSA in vitro, we recorded the release behavior of FITC-BSA at different time points by using in vivo imaging system, and the fluorescence intensity was quantified (). Consistent with the results of the above BSA release, PF/GAG composite hydrogels have better sustained release ability and inhibition of burst release on BSA in the early release stage, but with the increase of hydrogel dissolution, the release rate of BSA in PF/GAG composite hydrogels also accelerated.
Cell viability test
MTT experiment was performed to investigate the toxicity of the hydrogels on mouse pre-osteoblast cell line MC3T3-E1. As shown, the viability of cells in PF/GAG hydrogels (81.1% for PF/HA10, 90.7% for PF/HA90, 111.1% for PF/HA800 and 97.3% for PF/CS) were obviously higher than that in PF127 hydrogel (67.4%) (). Introduction of HA and CS could promote the viability of cells for being components of the extracellular matrix with excellent biocompatibility. PF/HA800 which contains the highest molecular weight of HA was more amicable to cells due to its higher compatibility and viscosity. Furthermore, in order to determine the ability of GAG that could effectively improve cell survival, MTT assays were also performed on PF/HA composite gels, which contains varying concentrations of hyaluronic acid (0.5%, 1.0% and 1.5%). The results demonstrated that more the concentration of hyaluronic acid, the better the compatibility of the hydrogel with the cells ().
Proliferation properties
To further detect the adhesion and proliferation of cells on the PF/GAG hydrogels, MC3T3-E1 cells were directly seeded on the hydrogels. After 3 days, a small amount of proliferation of cells occurred on PF127 hydrogel, but there were also many death cells (). However, dead cells were rarely seen during proliferation in PF/HA and PF/CS hydrogels (There were most live cells in PF/HA800 hydrogel where dead cells were rarely seen (). Gradually reduced living cells existed in PF/HA hydrogels with the decrease of molecular weight (). In PF/CS hydrogel, the live cells were slightly less than in PF/HA800 (). The results indicated that HA with higher molecular weight were more amicable to cells and promoted cell proliferation probably due to an increase of polysaccharide units in the structure, which obtained better biocompatibility. As another major component of ECM, CS also has good biocompatibility and the ability to promote cell metabolism, which made PF/CS also an appropriate injection hydrogel for cell proliferation.
Figure 5. Microphotos (A) and cell counts (B) of MC3T3-E1 cells cultured on different PF/GAG hydrogels after 5 days. a: PF127 hydrogel; b: PF/HA10 hydrogel; c: PF/HA90 hydrogel; d: PF/HA800 hydrogel; e: PF/CS hydrogel. Black solid arrowhead indicates live cells; Black hollow arrowhead indicates dead cells.
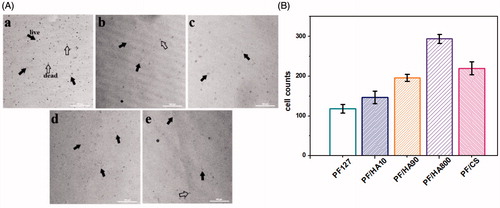
Joint histological analysis
Previous studies have proved that BMP-2 plays an important role in the repair of cartilage damage. In addition, the amount of BMP-2 needs to be controlled. A small amount of use will rapidly dilute and degrade in vivo, which cannot achieve the effect of promoting bone repair. However, massive use could cause hematoma, ectopic bone formation, tumor and excessive ossification [Citation30]. Josephine Fang [Citation31] substantiated that less than 1 µg of BMP-2 was sufficient to promote bone repair. Based on previous studies, 1 µg of BMP-2 was used in the 50 µl thermo-sensitive hydrogel in our study.
The injury of articular cartilage can be caused by osteoarthritis, and its histologic changes are mainly embodied in the corrosion of the cartilage surface, loss of extracellular matrix, chondrocytes death, and the accumulation of inflammatory cells. In this study, all treatment groups had therapeutic effect on damaged cartilage compared with OAG. () Besides, more severe injury occurred in BG group than other treatment group (, p < .01), demonstrating that the strategy of in situ BMP-2-loaded hydrogel had the effect of local sustained drug release and better therapeutic effect. Among all treatment groups, PF/GAG group showed much better recovery effect than PF127 hydrogels without GAG, and BPCG showed the best recovery efficacy of injured cartilage, probably because chondroitin sulfate was known to promote cartilage regeneration and anti-osteoarthritis effects. In other groups with PF/HA, the therapeutic effect of BPHG3 was also remarkable. Meanwhile, the PF/HA group with low-molecular weight of hyaluronic acid (BPHG1 and BPHG2) showed less recovery effect (, p < .01). HA plays an important endogenous role in the protection of articular cartilage and high-molecular weight hyaluronic acid in hydrogel showed a better therapeutic effect in this study, probably due to its preferable viscoelasticity, lubricating property and capacity in inhibition of inflammatory.
Figure 6. (A) Histological observation of Safranin O-Fast Green stained sagittal sections (magnification ×40 and ×200, respectively) of OA-injured knee joint treated with different conditions. CG: control group; OAG: OA group; BG: OA + BMP-2 positive control group; BPG: OA + PF127@BMP-2 group; BPHG1: OA + PF/HA10@BMP-2 group; BPHG2: OA + PF/HA90@BMP-2 group; BPHG3: OA + PF/HA800@BMP-2 group; BPCG: OA + PF/CS@BMP-2 group. Structures of joint were labeled (F: femur, T: tibia). The black square area was amplified in zoom image. Black solid arrowhead indicates normal chondrocytes; Black hollow arrowhead indicates damaged cartilage. (B) Mankin score for OA-injured knee joint treated with different conditions. Significant differences between experimental groups were identified via students’ t-test. (*p < .01).
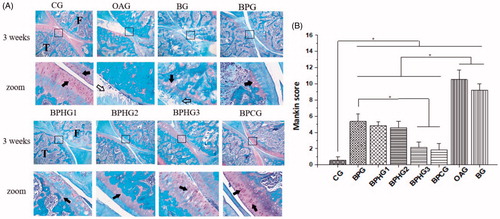
Conclusion
We have developed a thermo-sensitive hydrogel based on ECM for sustained protein drug release and recovery of injured cartilage. The syringe-ability was determined by appropriate viscosity of hydrogel in solution state, which was dependent on the concentration of PF127 and glycosaminoglycan. The protein drug showed a sustained release profile in the early stage from the composite hydrogel due to a certain affinity with glycosaminoglycan that prevented the initial burst release. Furthermore, the PF/GAG composite hydrogel had improved biocompatibility, which was confirmed by cell viability and proliferation of pre-osteoblastic MC3T3-E1 cells. In vivo results indicated that PF/GAG@BMP-2 composite hydrogel had an effect on recovery of injured cartilage, which was induced by osteoarthritis, and introduction of GAG showed better recovery efficacy. Therefore, we believe that this novel BMP-2 loaded injectable and thermo-sensitive hydrogel is promising in tissue engineering and potential for clinical recovery of injured cartilage.
Abbreviations | ||
PF127 | = | Pluronic F127 |
GAG | = | glycosaminoglycan |
HA | = | hyaluronic acid |
CS | = | chondroitin sulfate |
ECM | = | extracellular matrix |
OA | = | osteoarthritis |
BSA | = | bovine serum albumin |
BMP-2 | = | bone morphogenetic protein-2 |
Tgel | = | gelation temperature |
MIA | = | sodium iodoacetate |
Disclosure statement
No potential conflict of interest was reported by the authors.
Additional information
Funding
References
- Mei L, Shen B, Ling P, et al. Culture-expanded allogenic adipose tissue-derived stem cells attenuate cartilage degeneration in an experimental rat osteoarthritis model. PLoS One. 2017;12:e0176107.
- Kim SJ, Kim JE, Kim SH, et al. Therapeutic effects of neuropeptide substance P coupled with self-assembled peptide nanofibers on the progression of osteoarthritis in a rat model. Biomaterials. 2016;74:119–130.
- Cole BJ, Karas V, Hussey K, et al. Hyaluronic acid versus platelet-rich plasma: a prospective, double-blind randomized controlled trial comparing clinical outcomes and effects on intra-articular biology for the treatment of knee osteoarthritis. Am J Sports Med. 2017;45:339–346.
- He J, Jiang B, Dai Y, et al. Regulation of the osteoblastic and chondrocytic differentiation of stem cells by the extracellular matrix and subsequent bone formation modes. Biomaterials. 2013;34:6580–6588.
- Jung YS, Park W, Park H, et al. Thermo-sensitive injectable hydrogel based on the physical mixing of hyaluronic acid and Pluronic F-127 for sustained NSAID delivery. Carbohydr Polym. 2017;156:403–408.
- Zhu M, Wang J, Li N. A novel thermo-sensitive hydrogel-based on poly(N-isopropylacrylamide)/hyaluronic acid of ketoconazole for ophthalmic delivery.. Artif Cells Nanomed Biotechnol. 2017 [cited Aug 21]; [6 p.]. DOI:10.1080/21691401.2017.1368024
- Biazar E, Heidari Keshel S, Tavirani MR, et al. Bone reconstruction in rat calvarial defects by chitosan/hydroxyapatite nanoparticles scaffold loaded with unrestricted somatic stem cells. Artif Cells Nanomed Biotechnol. 2015;43:112–116.
- Peng S, Lin JY, Cheng MH, et al. A cell-compatible PEO-PPO-PEO (Pluronic(R))-based hydrogel stabilized through secondary structures. Mater Sci Eng C Mater Biol Appl. 2016;69:421–428.
- He C, Kim SW, Lee DS. In situ gelling stimuli-sensitive block copolymer hydrogels for drug delivery. J Control Release. 2008;127:189–207.
- Yang J, Chi L. Characterization of structural motifs for interactions between glycosaminoglycans and proteins. Carbohydr Res. 2017;452:54–63.
- Radhakrishnan J, Subramanian A, Krishnan UM, et al. Injectable and 3D bioprinted polysaccharide hydrogels: from cartilage to osteochondral tissue engineering. Biomacromolecules. 2017;18:1–26.
- Hu Y, Chen J, Fan T, et al. Biomimetic mineralized hierarchical hybrid scaffolds based on in situ synthesis of nano-hydroxyapatite/chitosan/chondroitin sulfate/hyaluronic acid for bone tissue engineering. Colloids Surf B Biointerfaces. 2017;157:93–100.
- Collins MN, Birkinshaw C. Hyaluronic acid based scaffolds for tissue engineering–a review. Carbohydr Polym. 2013;92:1262–1279.
- Liu C, Liu D, Wang Y, et al. Glycol chitosan/oxidized hyaluronic acid hydrogels functionalized with cartilage extracellular matrix particles and incorporating BMSCs for cartilage repair. Artif Cells Nanomed Biotechnol. 2018 [cited Feb 5]; [12 p.]. DOI:10.1080/21691401.2018.1434662
- Jalani G, Rosenzweig DH, Makhoul G, et al. Tough, in-situ thermogelling, injectable hydrogels for biomedical applications. Macromol Biosci. 2015;15:473–480.
- Dinescu S, Galateanu B, Albu M, et al. Biocompatibility assessment of novel collagen-sericin scaffolds improved with hyaluronic acid and chondroitin sulfate for cartilage regeneration. BioMed Res Int. 2013;2013:598056.
- Seo B-B, Koh J-T, Song S-C. Tuning physical properties and BMP-2 release rates of injectable hydrogel systems for an optimal bone regeneration effect. Biomaterials. 2017;122:91–104.
- Eğri S, Eczacıoğlu N. Sequential VEGF and BMP-2 releasing PLA-PEG-PLA scaffolds for bone tissue engineering: I. design and in vitro tests. Artif Cells Nanomed Biotechnol. 2017;45:321–329.
- Huang L, Shen M, Li R, et al. Thermo-sensitive composite hydrogels based on poloxamer 407 and alginate and their therapeutic effect in embolization in rabbit VX2 liver tumors. Oncotarget. 2016;7:73280–73291.
- Yu ZG, Geng ZX, Liu TF, et al. In vitro and in vivo evaluation of an in situ forming gel system for sustained delivery of Florfenicol. J Vet Pharmacol Ther. 2015;38:271–277.
- Fu C, Bai H, Hu Q, et al. Enhanced proliferation and osteogenic differentiation of MC3T3-E1 pre-osteoblasts on graphene oxide-impregnated PLGA–gelatin nanocomposite fibrous membranes. RSC Adv. 2017;7:8886–8897.
- Zhao C, Pandit S, Fu Y, et al. Graphene oxide based coatings on nitinol for biomedical implant applications: effectively promote mammalian cell growth but kill bacteria. RSC Adv. 2016;6:38124–38134.
- Ma X, He Z, Han F, et al. Preparation of collagen/hydroxyapatite/alendronate hybrid hydrogels as potential scaffolds for bone regeneration. Colloids Surf B Biointerfaces. 2016;143:81–87.
- Woo YJ, Joo YB, Jung YO, et al. Grape seed proanthocyanidin extract ameliorates monosodium iodoacetate-induced osteoarthritis. Exp Mol Med. 2011;43:561–570.
- Kim JE, Lee SM, Kim SH, et al. Effect of self-assembled peptide-mesenchymal stem cell complex on the progression of osteoarthritis in a rat model. Int J Nanomed. 2014;9(Suppl 1):141–157.
- Nivaggiol T, Alexandridis P, Hatton TA. Fluorescence probe studies of pluronic copolymer solutions as a function of temperature. Langmuir. 1995;11:730–737.
- Zhou Y, Fan X, Zhang W, et al. Stimuli-induced gel-sol transition of supramolecular hydrogels based on β-cyclodextrin polymer/ferrocene-containing triblock copolymer inclusion complexes. J Polym Res. 2014;21:359–368.
- Epstein-Barash H, Stefanescu CF, Kohane DS. An in situ cross-linking hybrid hydrogel for controlled release of proteins. Acta Biomater. 2012;8:1703–1709.
- Dumortier G, Grossiord JL, Agnely F, et al. A review of poloxamer 407 pharmaceutical and pharmacological characteristics. Pharm Res. 2006;23:2709–2728.
- Carragee EJ, Hurwitz EL, Weiner BK. A critical review of recombinant human bone morphogenetic protein-2 trials in spinal surgery: emerging safety concerns and lessons learned. Spine J. 2011;11:471–491.
- Fang J, Yang Z, Tan SJye, et al. Injectable gel graft for bone defect repair. Regen Med. 2014;9:41–51.