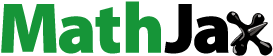
Abstract
In this study, a new support has been developed by immobilization of α-amylase onto modified magnetic Fe3O4-nanoparticles. The characterization of soluble and immobilized α-amylases with regards to kinetic parameters, pH, thermal stability and reusability was studied. The effect of polypyrrole/silver nanocomposite (PPyAgNp) percentage on weight of Fe3O4 and pH on the immobilization of α-amylase was studied. The highest immobilization efficiency (75%) was detected at 10% PPyAgNp/Fe3O4-nanocomposite and pH 7.0. Immobilization of α-amylase on PPyAgNp/Fe3O4-nanocomposite was characterized by FT-IR spectroscopy and scanning electron microscopy. The reusability of the immobilized enzyme activity was 80% of its initial activity after 10 reuses. The immobilized enzyme was more stable towards pH, temperature and metal ions compared with soluble enzyme. The kinetic study appeared higher affinity of immobilized enzyme (Km 2.5 mg starch) compared with soluble enzyme (Km 3.5 mg starch). In conclusion, the immobilization of α-amylase on PPyAgNp/Fe3O4-nanocomposite could successfully be used in industrial and medical applications.
Introduction
The use of free enzymes in biotechnological and environmental applications shows some significant problems such as inactivation, thermal instability, unfolding and no reusing. The immobilized enzymes characterized by the stability of enzyme activity, a wide pH range, higher thermal stability, easy separation from the solution and reusability [Citation1–3]. Several methods are routinely used for immobilization of enzymes encapsulation, covalent and non-covalent binding [Citation3]. Covalent binding is the efficient method because no leakage of enzyme would be possible. However, immobilization of enzymes on nanoparticles exhibited several characters such as high surface of the support, high stability in environmental conditions, diffusion limitations and less stearic hindrance [Citation4].
α-Amylase, as one of the amylases, is highly interested due to its various applications [Citation5–7]. It catalyzes the hydrolysis of starch to glucose, maltose and maltotriose [Citation8]. Amylolytic enzymes are the most commercial enzymes used in various industrial applications [Citation9,Citation10].
The immobilization of biomolecules on metal nanoparticles developed and improved several applications including diagnostics, biosensors and drug delivery [Citation11–16]. Several studies reported the immobilization of α-amylase with metal nanoparticles such as magnetic Fe3O4 nanoparticles [Citation17], silica nanoparticles [Citation18], amino-functionalized magnetite nanoparticles [Citation19], gold nanoparticles (AuNPs) and silver nanoparticles (AgNPs) [Citation20]. However, using high-intensity ultrasound, in situ generated α-amylase nanoparticles (NPs) were immobilized on polyethylene films [Citation21]. Our study aimed to immobilize α-amylase from Trichoderma harzianum onto a combination of magnetic Fe3O4-nanoparticles and PPyAgNp nanocomposites. We present our results on characterization of soluble and immobilized α-amylases with regards to kinetic parameters, pH, thermal stability and reusability.
Materials and methods
Materials
Analytical grade pyrrole, silver nitrate, Fe3O4 magnetic nanoparticles were purchased from Sigma-Aldrich (St Louis, MO, USA) and was used as received. Aqueous solutions were prepared from distilled water.
Trichoderma harzianum α-amylase
Trichoderma harzianum α-amylase was previously purified and characterized with wide substrate specificity [Citation22].
Synthesis of nanocomposite
PPyAgNp nanocomposite was synthesized by an oxidative polymerization of pyrrole in the presence of silver nitrate as the oxidant according to the reported method [Citation23]. PPyAgNp was combined in different percentages with Fe3O4 magnetic nanoparticles to get different PPyAgNP/Fe3O4 nanocomposites.
Immobilization procedure
Enzyme immobilization was carried out by end over end at 90 rpm on the PPyAgNP/Fe3O4 nanocomposites using a solution of T. harzianum α-amylase made in 50 mM sodium acetate buffer pH 5.5 or Tris-HCl pH 7.2 at room temperature during overnight. Aliquots of the supernatant were drawn up and the PPyAgNP/Fe3O4 nanocomposite was dried at room temperature to verify the advancement of the immobilization. The relative activity% of immobilized enzyme was calculated from the following formula:
α-Amylase assay
α-Amylase activity assay was carried out by DNS method [Citation24], for both soluble and immobilized enzymes. Ten milligrams of PPyAgNp/Fe3O4-nanocomposite-α-amylase was taken for routine assay of the activity of immobilized enzyme. PPyAgNp/Fe3O4-nanocomposite was removed after 10 min incubation with 1 ml starch (1%) and 1 ml DNS was added for colour development. The tube containing this reaction mixture was incubated in a boiling water bath for 10 min and then cooled in running tap water and the absorbance was recorded at 540 nm. One unit of activity was defined as the amount of enzyme required to produce 1 μmoL of maltose/min.
Structure characterization
The attenuated total reflectance-Fourier transform infrared (ATR-FTIR) spectra for PPyAgNp/Fe3O4-nanocomposite samples were performed on a PerkinElmer spectrum 100 FT-IR spectrometer (Walthan, USA). The morphology and size of the PPyAgNp/Fe3O4-nanocomposite were characterized at 100 kV by a JEOL 2010 TEM (Michigan, USA).
Reusability of immobilized enzyme
After each assay the immobilized enzyme preparation was taken out, washed with 50 mM Tris-HCl buffer, pH 7.0 and stored overnight at 4 °C. The immobilized enzyme recovered by this procedure was used repeatedly. The activity determined for the first time was considered as control (100%) for the calculation of remaining percentage activity after each use.
Enzyme characterization
Estimates of optimal temperature and pH for soluble α-amylase and immobilized α-amylase were made by using a temperature ranged from 30 °C to 80 °C and a pH ranged from 4.0 to 8.5. The thermal stability was investigated by measuring the residual activity of soluble α-amylase and immobilized α-amylase after one hour of incubation at different temperatures. The Km values were determined from Lineweaver–Burk plots by using different concentrations of starch as substrate (1.5–3.5 mg).
Effect of metal ions
The effects of various metal ions on enzyme activity of soluble and immobilized α-amylases were determined by pre-incubating the enzyme with 5 mM metal ions for 15 min and then assaying the enzyme activity. The activity in absence of metal ions is taken as 100%.
Statistical analysis
The statistical analyses were performed by a one-way ANOVA and the Student's t-test. The results expressed as means ± SE difference were considered significant when p < .05.
Results and discussion
Since amylase enzyme contains thiol and/or disulfide and amino acid groups among its chemical structure, thus it was hypothesized that having a composition of PPy and AgNp would furnish a good enzyme immobilization matrix by virtue of the positive charges of PPy [Citation23], which help binding with enzyme by ionic bonds and AgNp with its propensity to bind with thiol and/or disulfide groups [Citation25]. Generally, Fe3O4 magnetic nanoparticles have been used in protein/enzyme immobilization [Citation26]. Also the use of magnetite in the composition is beneficial for easy magnetic separation. In the present study, PPyAgNp was mixed with Fe3O4 magnetic nanoparticles to become PPyAgNP/Fe3O4 nanocomposite () in order to immobilize Trichoderma harzianum α-amylase. The immobilization of enzyme on magnetic Fe3O4-nanoparticles combined with different concentrations of PPyAgNP at different pH was carried out. The highest immobilization efficiency (75%) was detected at 10% PPyAgNP and pH 7.0 (). The loss of the activity of immobilized enzyme by increasing the PPyAgNP concentration could be attributed to the presence of multipoint attachments of the enzyme to the nanocomposite. The rate of the enzyme immobilization depends on the enzyme concentration. shows the rate of immobilization increased with increasing α-amylase concentration. The highest rate of immobilization was detected at 30 unit of enzyme (75% residual activity).
Figure 2. The effect of T. harazianum α-amylase concentration on the rate of immobilization on PPyAgNp/Fe3O4-nanocomposite. Each point represents the mean of three experiments ± SE.
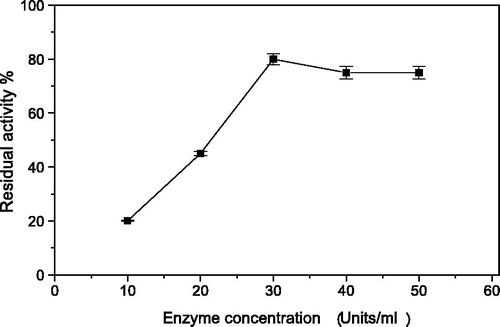
Table 1. Effect of mixing PPyAgNp with magnetic Fe3O4 nanoparticles and pH on the immobilization efficiency of T. harzianum α-amylase.
The (ATR-FTIR) spectra of magnetite nanoparticles Fe3O4, 10% PPyAgNp/Fe3O4 (w/w) and 10% PPyAgNp/Fe3O4-α-amylase samples are shown in . All samples exhibited similar absorption peaks with observed differences. Before α-amylase immobilized onto 10% PPyAgNp/Fe3O4 (w/w), characteristic absorption peaks centred at 557 cm−1 are due to Fe-O vibrations of Fe3O4 [Citation27]. After α-amylase immobilized onto 10% PPyAgNp/Fe3O4 (w/w), a slight change is observed around 557 cm−1. Since α-amylase is a protein enzyme containing glucosidic moiety, thus its C–O stretching vibration would appear with strong intensity as clearly observed at 1070 cm−1 in 10% PPyAgNp/Fe3O4 α-amylase sample, indicating the success of α-amylase immobilized onto 10% PPyAgNp/Fe3O4 (w/w).
Figure 3. FT-IR spectra of Fe3O4-nanoparticles, PPyAgNp/Fe3O4-nanocomposite and PPyAgNp/Fe3O4-nanocomposite-α-amylase.
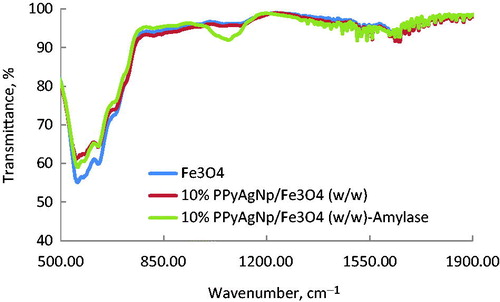
The SEM images of Fe3O4 magnetic nanopraticles, 10% PPyAgNp/Fe3O4 (w/w) and 10% PPyAgNp/Fe3O4 (w/w)-α-amylase samples are shown in . It is observed that the morphology of 10% PPyAgNp/Fe3O4 (w/w) sample is brighter and compact compared with that of Fe3O4 magnetic nanopraticles, indicating the presence of PPyAgNp. Also, SEM images show a typical agglomeration of magnetic nanoparticles with a better surface coverage and appearance of large particles in case 10% PPyAgNp/Fe3O4 (w/w)- α-amylase samples compared with those of Fe3O4 and 10% PPyAgNp/Fe3O4 (w/w), indicating the success of α-amylase immobilization.
Figure 4. SEM images of Fe3O4-nanoparticles, PPyAgNp/Fe3O4-nanocomposite and PPyAgNp/Fe3O4-nanocomposite-α-amylase.
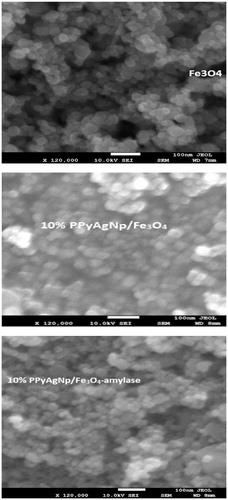
In industrial applications, the reuse of immobilized enzymes is very efficient compared with free enzymes. The immobilized α-amylase on 10% PPyAgNp/Fe3O4-nanocomposite was reused 10 repeats and retained 80% of its initial activity (). Similarly, the immobilized α-amylases on magnetic Fe2O3 nanoparticles and amino-functionalized magnetite nanoparticles were reusable for 8 and 9 consecutive use while retaining 83% and 68% of its initial activity, respectively [Citation17,Citation19].
Figure 5. Reuse of PPyAgNp/Fe3O4-nanocomposite-α-amylase. The reaction mixture of each repeat includes: 10 mg starch, 30 units of immobilized enzyme, 50 mM Tris-HCl buffer pH 7.0, incubation temperature at 50 °C and incubation time for 10 min. Each point represents the mean of three experiments ± SE.
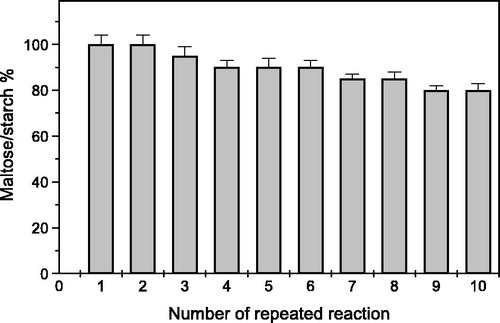
The pH optimum of soluble α-amylase and immobilized α-amylase on 10% PPyAgNp/Fe3O4-nanocomposite was detected at 6.0 and 6.5, respectively (). The same pH optimum (pH 6.5) of α-amylase immobilized on nanoparticles such as nanoCaCO3 [Citation28], nano-polyethylene film [Citation21] and amino-functionalized magnetite nanoparticles were reported [Citation19].
Figure 6. Optimum pH of soluble and immobilized T. harazianum α-amylase on PPyAgNp/Fe3O4-nanocomposite. Each point represents the average of two experiments.
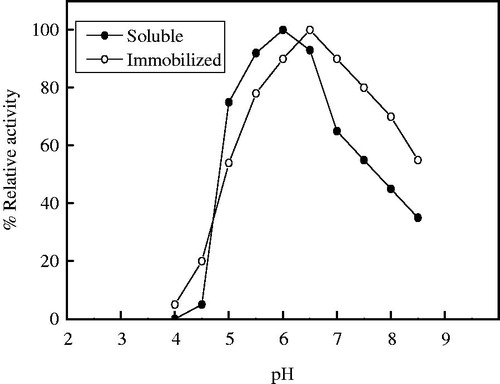
The optimum temperature of soluble α-amylase and immobilized α-amylase on 10% PPyAgNp/Fe3O4-nanocomposite was detected at 40 °C and 50 °C, respectively (). At 80 °C, the results appeared that the immobilized enzyme retained 40% of its initial activity, while the soluble enzyme retained 20%. The results showed that optimum temperature for the immobilized enzyme shifted towards higher temperatures. The same results reported that the temperature optimum of free α-amylase shifted from 40 °C to 50 °C after immobilization of α-amylase on magnetic Fe2O3 nanoparticles [Citation17]. The slow degradation of the substrate was detected at higher temperature because the structure of enzyme is altered [Citation29]. After immobilization an increase in temperature optimum of immobilized enzymes revealed that the enzyme might be more rigid to structural changes induced by heat [Citation30,Citation31]. On the contrary, the low and the same optimum temperature of free and immobilized α-amylase on nano-polyethylene film was 30 °C [Citation21]. The maximum activity was also observed at 40 °C for both free and immobilized α-amylase amino-functionalized magnetite nanoparticles [Citation19]. The study of thermal stability is shown in . Up to 50 °C the two enzymes were thermal stable after incubation for one hour, whereas at 80 °C the soluble and immobilized α-amylases lost 85% and 70% of its activity, respectively.
Figure 7. Optimum temperature of soluble and immobilized T. harazianum α-amylase on PPyAgNp/Fe3O4-nanocomposite. Each point represents the average of two experiments.
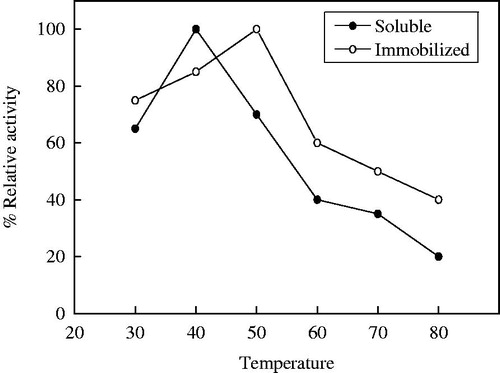
Figure 8. Thermal stability of soluble and immobilized T. harazianum α-amylase on PPyAgNp/Fe3O4-nanocomposite. Each point represents the average of two experiments.
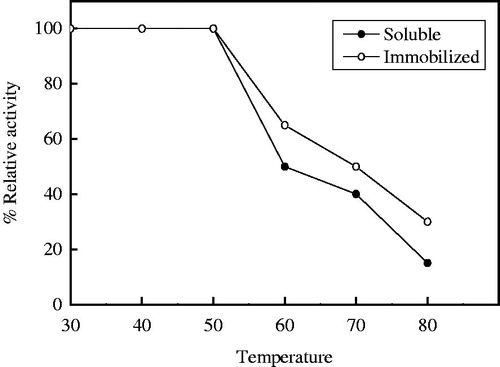
Various substrate analogues were efficiently degraded by immobilized enzyme compared with soluble enzyme (). Therefore, 10% PPyAgNp/Fe3O4-nanocomposite is the best support, which makes the substrate bind to the active site of the enzyme easily. The Km values of the soluble and immobilized α-amylase were 3.5 mg and 2.5 mg starch, respectively (). Similarly, the Km of α-amylase immobilized on amino-functionalized magnetite nanoparticles and nano-polyethylene film was lower than that of soluble enzyme [Citation19–21].
Figure 9. Km of soluble and immobilized T. harazianum α-amylase on PPyAgNp/Fe3O4-nanocomposite. Each point represents the average of two experiments.
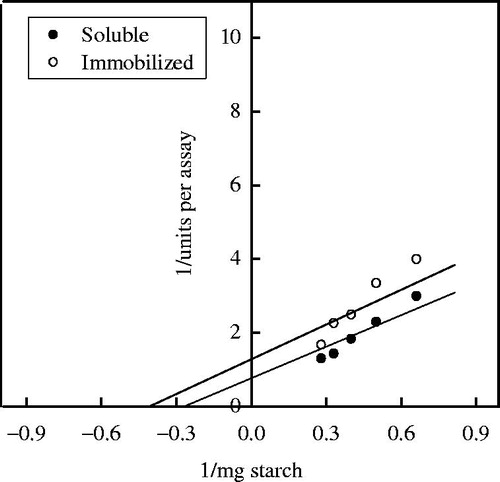
Table 2. The substrate specificity of soluble and immobilized α-amylases on PPyAgNp/Fe3O4-nanocomposite.
The influence of metal cations on the activity of soluble and immobilized enzymes was examined (). Ni2+, Ca2+ and Co2+ had no effect on immobilized α-amylase activity, and Co2+ also had no effect on soluble α-amylase The other tested metals had partial inhibitory effect on soluble and immobilized α-amylases except that of Hg2+ which was designated as very strong inhibitor for the activity of the soluble enzyme (82% inhibition) compared with the immobilized enzyme (61% inhibition). The activity of α-amylase immobilized on magnetic Fe2O3 nanoparticles and amino-functionalized magnetite nanoparticles was influenced by few metal cations [Citation19–21]. The immobilization process improved the resistance of enzyme toward the inhibition caused by metal ions. In industrial processes which applied the enzyme, the metal cations are often present in crude materials.
Table 3. The effect of 5 mM metal ions on the soluble and immobilized α-amylases on PPyAgNp/Fe3O4-nanocomposite.
Conclusions
The study developed a new support, 10% PPyAgNp/Fe3O4-nanocomposite, for immobilization of α-amylase. The immobilized enzyme was reused 10 times retaining 80% of its initial activity. The affinity of immobilized enzyme towards starch was higher compared with soluble enzyme. The immobilized enzyme hydrolyzed substrate analogues with high efficiencies. The immobilization of enzyme on nanoparticles could successfully be used in industrial and medical applications.
Disclosure statement
The authors report no conflicts of interest
References
- Batra R, Gupta MN. Enhancement of enzyme activity in aqueous-organic solvent mixtures. Biotechnol Lett. 1994;16:1059–1064.
- Straathof AJ, Panke S, Schmid A. The production of fine chemicals by biotransformations. Curr Opin Biotechnol. 2002;13:548–556.
- Wang Z-G, Wang J-Q, Xu Z-K. Immobilization of lipase from Candida rugosa on electrospun polysulfone nanofibrous membranes by adsorption. J Mol Catal B: Enzym. 2006;42:45–51.
- Liu W, Wang L, Jiang R. Specific enzyme immobilization approaches and their application with nanomaterials. Top Catal. 2012;55:1146–1156.
- Gupta R, Gigras P, Mohapatra H, et al. Microbial α-amylases: a biotechnological perspective. Process Biochem. 2003; 38:1599–1616.
- Kandra L. α-Amylases ofmedical and industrial importance. J Mol Struc. 2003;666:487–498.
- Pandey A, Nigam P, Soccol CR, et al. Advances in microbial amylases. Biotechnol Appl Biochem. 2000;31:135–152.
- Reddy NS, Nimmagadda A, Sambasiva Rao KRS. An overview of the microbial α-amylase family. Afr J Biotechnol. 2003;2:645–648.
- Sidhu GS, Sharma P, Chakrabart T, et al. Strain improvement for the production thermostable α-amylase. Enzyme Microb Technol. 1997;21:525–530.
- Walsh G. Industrial enzymes: an introduction. In: Walsh G, edior. Biochemistry and biotechnology. New York: Wiley; 2002. p. 454–465.
- Chau C-F, Wu S-H, Yen G-C. The development of regulations for food nanotechnology. Trends Food Sci Technol. 2007;18:269–280.
- Das M, Mohanty C, Sahoo SK. Ligand-based targeted therapy for cancer tissue. Expert Opin Drug Deliv. 2009;6:285–304.
- Furno F, Morley KS, Wong B, et al. Silver nanoparticles and polymeric medical devices: a new approach to prevention of infection. J Antimicrob Chemother. 2004;54:1019–1024.
- Roe D, Karandikar B, Bonn-Savage N, et al. Antimicrobial surface functionalization of plastic catheters by silver nanoparticles. J Antimicrob Chemother. 2008;61:869–876.
- Daraee H, Eatemadi A, Abbasi E, et al. Application of gold nanoparticles in biomedical and drug delivery. Artif Cell Biomed Biotechnol. 2016;44:410–422.
- Haghiralsadat F, Amoabediny G, Helder MN, et al. A comprehensive mathematical model of drug release kinetics from nano-liposomes, derived from optimization studies of cationic PEGylated liposomal doxorubicin formulations for drug-gene delivery. Artif Cell Biomed Biotechnol. 2018;46:169–177.
- Khan MJ, Husain Q, Azam A. Immobilization of porcine pancreatic α-amylase on magnetic Fe2O3 nanoparticles: applications to the hydrolysis of starch. Biotechnol Bioproc E. 2012;17:377–384.
- Soleimania M, Khania A, Najafzadeh K. α-Amylase immobilization on the silica nanoparticles for cleaning performancetowards starch soils in laundry detergents. J Mol Catal B: Enzym. 2012;74:1–5.
- Akhond M, Pashangeh K, Karbalaei-Heidari HR, et al. Efficient immobilization of porcine pancreatic α-amylase on amino-functionalized magnetite nanoparticles: characterization and stability evaluation of the immobilized enzyme. Appl Biochem Biotechnol. 2016;180:954–968.
- Saware K, Aurade RM, Jayanthi PDK, et al. Modulatory effect of citrate reduced gold and biosynthesized silver nanoparticles on α-amylase activity. J Nanopart. 2015;2015:829718.
- Meridor D, Gedanken A. Preparation of enzyme nanoparticles and studying the catalytic activity of the immobilized nanoparticles on polyethylene films. Ultrasonics Sonochem. 2013;20:425–431.
- Mohamed SA, Azha EI, Ba-Akdah MM, et al. Production, purification and characterization of α-amylase from Trichoderma harzianum grown on mandarin peel. Afr J Microbiol Res. 2011;5:930–940.
- Omastová M, Mosnácková K, Fedorko P, et al. Polypyrrole/silver composites prepared by single-step synthesis. J Stejskal, Synth Metals. 2013;166:57–62.
- Miller GL. Use of dinitrosalicylic acid reagent for the determination of reducing sugar. Anal Chem. 1959;31:426–429.
- Ernest V, Gajalakshmi S, Mukherjee A, et al. Enhanced activity of lysozyme-AgNP conjugate with synergic antibacterial effect without damaging the catalytic site of lysozyme. Artif Cells Nanomed Biotechnol. 2014;42:336–343.
- Liu Y, Jia S, Wu Q, et al. Studies of Fe3O4-chitosan nanoparticles prepared by co-precipitation under the magnetic field for lipase immobilization. Catal Comm. 2011;12:717–720.
- Kumari M, Pittman CU, Jr, Mohan D. Heavy metals [chromium (VI) and lead (II)] removal from water using mesoporous magnetite (Fe3O4) nanospheres. J Coll Interf Sci. 2015;442:120–132.
- Demir S, Gok SB, Kahraman MV. α-Amylase immobilization on functionalized nano CaCO3 by covalent attachment. Starch/Stärke. 2012;64:3–9.
- Singh V, Kumar P. Carboxy methyl tamarind gum–silica nanohybrids for effective immobilization of amylase. J Mol Catal B: Enzym. 2011;70:67–73.
- Magri ML, Miranda MV, Cascone O. Immobilization of soybean seed coat peroxidase on polyaniline: synthesis optimization and catalytic properties. Biocatal Biotrans. 2005;23:339–346.
- Lee PM, Lee KH, Siaw SY. Covalent immobilization of aminoacrylase to alginate for L-phenylalanine production. J Chem Technol Biotechnol. 1993;58:65–70.