Abstract
The water extract of Anemarrhena asphodeloides, the traditional oriental medicinal plant, mediated the eco-friendly synthesis of silver nanoparticles (Aa-AgNPs) and gold nanoparticles (Aa-AuNPs). First, its therapeutic rhizome was powdered prior to water extraction and then silver, gold nanoparticles were synthesized. Aa-AgNPs and Aa-AuNPs were found to be spherical, face-centred cubic nanocrystals with a Z-average hydrodynamic diameter of 190 and 258 nm, respectively. In addition, proteins and aromatic biomolecules were the plausible players associated with the production and stabilization of Aa-AgNPs; instead, phenolic compounds were responsible for the synthesis and stability of Aa-AuNPs. In vitro cytotoxic analysis revealed that up to 50 μg.mL−1 concentration Aa-AuNPs did not exhibit any toxicity on 3T3-L1, HT29 and MCF7 cell lines, while being specifically cytotoxic to A549 cell line. On the contrary, Aa-AgNPs displayed a significantly higher toxicity in comparison to Aa-AuNPs in all cell lines specially MCF7 cell line. Since cancer cells were more sensitive to Aa-Au/AgNPs treatments, further evaluation was done in order to determine their anticancer potential. Reactive oxygen species (ROS) generation was not affected by Aa-AuNPs, on the other hand, Aa-AgNPs treatment exhibited a higher potential to induce oxidative stress in A549 cells than HT29 and MCF7 cells. In addition, Aa-Ag/AuNPs reduced cell migration in A549 cells at 10 and 50 μg.mL−1, respectively. So far, this is the only report uncovering the ability of A. asphodeloides to synthesize silver and gold nanoparticles with anticancer potential and also indirectly enabling its large-scale utilization with value addition.
Introduction
Anemarrhena asphodeloides is a widely used medicinal plant in oriental countries and it belongs to Asparagaceae family. Many of its medicinal properties namely anti-fungal, anti-cancerous, anti-inflammatory, anti-diabetic, Anti-UV, etc. have been demonstrated [Citation1–5]. The idea of employing metal and polymer nanoparticles for combating diseases has increased in the recent past [Citation6,Citation7]. Silver nanoparticles (Aa-AgNPs) possess inherent biological activities [Citation8] and gold nanoparticles (Aa-AuNPs) have been chiefly used in drug delivery [Citation9]. However, the physical and chemical strategies employed for the mass production of nanoparticles generally leaves toxic and environmentally hazardous compounds as by-products. Hence, using microbes and plants as the source for nanoparticle mass production is demonstrated to be an eco-friendly alternative [Citation10]. Moreover, these metal NPs synthesized via biological route were reported to exhibit strong biological activities [Citation11,Citation12].
Owing to the fact that, A. asphodeloides is a medicinal plant, the nanoparticles produced form it is also expected to possess similar therapeutic properties. Furthermore, A. asphodeloides is an endemic medicinal plant, thus its benefits are restricted to a certain geographical location. Therefore, the idea of synthesizing nanoparticles could enable wide-spread usage of the plant in-directly. The water extract of A. asphodeloides mediated the synthesis of Aa-AgNPs and Aa-AuNPs. These nanoparticles were characterized by several physicochemical techniques, such as Ultraviolet–visible (UV–Vis) spectrophotometry, field emission transmission electron microscopy (FE-TEM), energy dispersive X-ray spectroscopy (EDX), selected area electron diffraction pattern (SAED), dynamic light scattering particle size analysis (DLS), X-ray diffraction (XRD) analysis and Fourier transform infrared analysis (FTIR). Furthermore, both Aa-Ag/AuNPs were evaluated for cytotoxicity towards pre-adipocyte (3T3-L1), lung adenocarcinoma (A549), colon cancer (HT29) and breast cancer (MCF7). In addition, the potential anticancer activity was studied through in vitro experiments.
Materials and methods
Materials
The dried rhizome of Anemarrhena asphodeloides was obtained from the Ginseng bank, Kyung Hee University, South Korea. Silver nitrate (AgNO3) and Gold (III) chloride trihydrate (HAuCl4.3H2O) were bought from Sigma Aldrich Co. (St. Louis, MO). Other chemicals used in this study were of analytical grade and used as received.
Preparation of crude extract
The dried rhizome of A. asphodeloides was powdered by using a blender and was extracted using water. About 10 g of the powder was boiled at 100 °C in 200 ml of sterile water for 30 min. The collected extract was centrifuged at 10,000 rpm for 10 min after filtering for the removal of debris. The resulting supernatant was used as the source to synthesize both Aa-Ag/AuNPs.
Synthesis of silver and gold nanoparticles
Of, 1 mM silver nitrate (AgNO3) and gold (III) chloride trihydrate (HAuCl4⋅3H2O) were employed for A. asphodeloides mediated eco-friendly production of Aa-AgNPs and Aa-AuNPs, respectively. The synthesis was tested across three temperature ranges namely room temperature (RT), 40 and 80 °C. Then, the resultant mixture was centrifuged at 17,000 rpm for 20 min to precipitate.
Aa-AgNPs and Aa-AuNPs were additionally purified by repeated centrifugation and dissolution in sterile water thrice, to get rid of water-soluble impurities. Finally, the resulting purified Aa-Ag/AuNPs was utilized for physicochemical characterization and for evaluating cytotoxicity on various human cell lines.
Physicochemical characterization of Aa-Ag/AuNPs
Physicochemical characterizations were conducted out in-order to uncover the physical and chemical characteristics of the synthesized Aa-Ag/AuNPs, similar to our previous report with minor modifications [Citation13]. To confirm the formation of Aa-Ag/AuNPs, the corresponding reaction mixture was scanned between 300 and 800 nm using UV–Vis spectrophotometer (2100 Pro; Amersham Biosciences Corp., Piscataway, NJ). Then, their stability was analysed by re-checking the absorbance after incubating at room temperature for a period of 1 month. Ultrospec (FE-TEM), EDX, and elemental mapping were performed with a JEM-2100 F (JEOL, Tokyo, Japan) operated at 200 kV to uncover the physical properties and purity of both the nanoparticles. They were oven dried at 60 °C on a carbon-coated copper grid before the microscopic analysis. To dissect out the hydrophobic diameter and polydispersity index of the Aa-Ag/AuNPs, dynamic light scattering analysis was carried out with a particle size analyser (Photal, Otsuka Electronics Co., Osaka, Japan) at 25 °C, with pure water (refractive index of 1.3328; viscosity of 0.8878; dielectric constant of 78.3) as the reference dispersive medium. For the X-ray diffraction (XRD) and FTIR analyses, purified and air dried were used. Three dimensional configuration and crystallinity of these nanoparticles were determined using X-ray diffractometer, D8 Advance (Bruker, Billerica, MA), with a set of defined configurations (40 kV; 40 mA; CuKα radiation 1.54 Å; a scanning rate of 6 °/min; step size 0.02; over the 2θ range of 20–80°). Aa-Ag/AuNPs were analysed using FTIR spectrophotometer (PerkinElmer Inc., Waltham, MA) in the range of 450–4000 cm−1 at a resolution of 4 cm−1 to identify the functional groups present on their surface.
Evaluation of in vitro cytotoxicity on normal and cancer cell lines
Pre-adipocyte cells (3T3-L1), human lung adenocarcinoma cells (A549), colon cancer cells (HT29) and breast cancer cells (MCF7) were cultured at 37 °C in a humidified incubator with a 5% CO2 atmosphere. The cytotoxicity of both Aa-Ag/AuNPs and a commercial silver nanoparticles (cAgNPs) was determined by the MTT (3, 4, 5-dimethylthiazol-2-yl)-2–5-diphenyletrazolium bromide) assay. Briefly, the cells were seeded at a concentration of 1 × 105 cells/ml in a 96 well plate (NEST®, Brooklawn, NJ). After 24 h, the cells were subjected to treatment for 48 h. Following the treatment, 10 µl of MTT (5 mg/ml) was added to each well and incubated for 3 h. Subsequently, the media containing treatment and MTT reagent was removed by aspiration, replaced with 100 µl of DMSO and incubated for 30 min. Finally, the amount of formazan formed by viable cells was measured using multi-model plate reader (Bio-Tek Instrument, Winooski, VT) at a test, reference wavelength of 570 and 630 nm, respectively [Citation11].
Reactive oxygen species generation (ROS)
The potential to induce oxidative stress was evaluated using 2′,7′–dichlorofluorescin diacetate (DCFDA) reagent. Briefly, A549, HT29 and MCF7 cell lines were seeded in a 96-well plate, after overnight incubation the media was replaced by DCFDA-media at 25 mM and incubate during 30 min at 37 °C. Next, DCFDA-media was removed and media with treatment was added and incubated for 48 h. After treatment time finish, media was kept in 96-well dark plate and fluorescence was read using multi-model plate reader [Citation11].
Wound-healing assay
In order to evaluate the potential effect of Aa-Au/AgNPs on cell migration, A549 cells were used as a model in the presence of epithelial growth factors (EGF) stimulation. The cells were seed in a 12-well plate and incubate until 100% growth was reached. Priory to cell scratch, the media was replaced with 1 × PBS. The scratch was made using a 10 µl tip, then cells were washed twice with 1 × PBS and treated in presence of EGF at 40 ng/mL, a control without treatment and EGF was kept as reference. Pictures were taken at 0 and 18 h of treatment. Migration ratio was determinate by TScratch software (Swiss Federal Institute of Technology, ETH Zurich, Zurich, Switzerland) [Citation14].
Statistical analysis
The differences in the results were considered significant at p ≤ .05. The analysis was performed by using Graph Pad version 6.04 software (GraphPad Software Inc., La Jolla, CA). Results are expressed as mean ± SEM. The statistical significance of differences between values was evaluated by one-way ANOVA.
Results and discussion
The overall graphical representation of the A. asphodeloides mediated green synthesis of Aa-Ag/AuNPs and its biological effects are illustrated in .
Figure 1. The overall graphical representation of the Anemarrhena asphodeloides mediated eco-friendly synthesis of silver (Aa-AgNPs) and gold (Aa-AuNPs) nanoparticles.
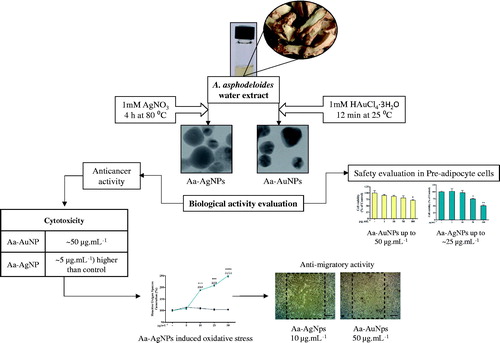
Determination of optimal conditions for the synthesis of Aa-Ag/AuNPs
For the production of both Aa-Ag/AuNPs, the reaction parameters were optimized in terms of temperature and time. In regard with Aa-AgNPs, incubation at 80 °C for 4 h showed the best results. On the other hand, in the case of Aa-AuNPs, synthesis at room temperature for 12 min was chosen. Also, temperature did not play a major role in the synthesis of Aa-AuNPs (). Similar results were reported previously for the production of Aa-AgNPs and Aa-AuNPs from Siberian ginseng [Citation15]. The mass production of both Aa-Ag/AuNPs for physicochemical characterization the standardized parameters were used, evaluation of cytotoxicity and anticancer potential was done at in vitro level.
Synthesis and physicochemical characterization
The water extract of A. asphodeloides displayed the potential to form Aa-Ag/AuNPs and was indicated by colour change in the reaction mixture upon the addition of appropriate salts at 1 mM concentration. Aa-AgNPs were synthesized when incubated at 80 °C for 4 h which was reflected in the reaction mixture by the colour change from light yellow to dark brown. Moreover, prominent peak at 413 nm during time-dependent UV spectroscopy over the range of 300–800 nm is associated with the surface plasmon resonance band of Aa-AgNPs (). Similarly, Aa-AuNPs were produced at room temperature in about 12 min and a characteristic peak at 550 nm corresponds to the surface plasmon resonance band of Aa-AuNPs (). In addition, the UV–Vis spectrum of both the nanoparticles displayed no significant changes after a month-long incubation, confirming their stability.
Most of the previous studies employ TEM to determine the shape of the synthesized nanoparticles owing to its greater resolution than SEM [Citation13]. Thus, FE-TEM analysis was conducted and both Aa-Ag/AuNPs were found to be mostly spherical (). Also, EDX and elemental mapping confirmed the purity and elemental composition of both the nanoparticles (). Optical absorption band peaks at ∼3 and 2.3 keV in the EDX spectrum are attributed to the Aa-AgNPs and Aa-AuNPs, respectively. Similar results were reported in the past [Citation16]. The Z-average hydrodynamic diameters of Aa-AgNPs and Aa-AuNPs were about 190 and 258 nm, respectively, as determined by dynamic light scattering particle size analysis (). In addition, the polydispersity index was found to be 0.27 for both the nanoparticles.
Figure 3. FE-TEM uncovered that both Aa-AgNPs (i(a,c)) and Aa-AuNPs (ii(a,c)) are predominantly spherical. SAED pattern indicated the crystallinity of both Aa-AgNPs (i(b)) and Aa-AuNPs (ii(b)). Furthermore, the purity of Aa-AgNPs (i(d,e)), Aa-AuNPs (ii(d,e)) were determined by elemental mapping and EDX analyses. Scale bar: (i(a)) and (ii(a)) – 5 nm; (i(c)) and (i(d)) – 80 nm; (ii(c)) and (ii(d)) – 70 nm.
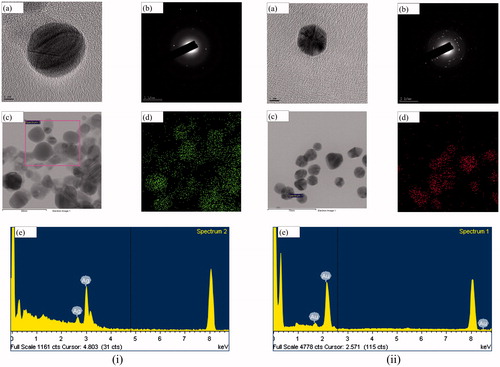
Figure 4. DLS analysis indicated the particle size distribution based on intensity of both Aa-AgNPs (a) and Aa-AuNPs (b).
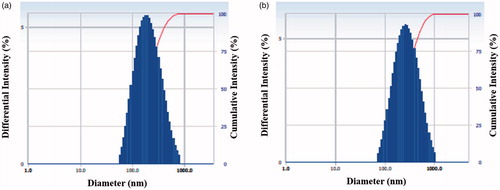
Fascinatingly, the XRD pattern of Aa-AgNPs revealed the presence of both silver and silver chloride nanocrystals. Aa-AgNP peak is correlated with 2θ = 38.16° [111] and the peaks 2θ = 28.04° [111], 32.379 [200], 46.33° [220] and 76.97° [420] correspond to crystalline silver chloride nanoparticle. The average crystallite size of Aa-AgNPs is found to be 20.87 nm using Scherrer equation (). Similar results were documented by others [Citation16,Citation17]. On the other hand, Aa-AuNPs exhibited characteristic peaks at 2θ = 38.20° (111), 44.37° (200), 64.95° (220) and 77.84° (311) with the crystallite size of 10.88 nm (). This is in line with the results for standard gold metal (Joint Committee on Powder Diffraction Standards – JCPDS, USA). Furthermore, XRD analyses indicated that both Aa-Ag/AuNPs are crystalline face-centred cubic nanostructures. Besides, the SAED pattern also reconfirmed the crystalline nature (). The inconsistency between the Z-average hydrodynamic diameter from the dynamic light scattering analysis particle size analysis and average crystallite diameter obtained from the Scherrer equation is because the earlier is inclusive of the plant biomolecules coating upon the nanoparticles while the latter is purely their core diameter [Citation16,Citation17].
Figure 5. The crystallinity of both Aa-AgNPs (a) and Aa-AuNPs (b) were confirmed by XRD analysis. In addition, the biological molecules attached to Aa-AgNPs and Aa-AuNPs were determined by FTIR (c). The FTIR pattern of the extract is also indicated.
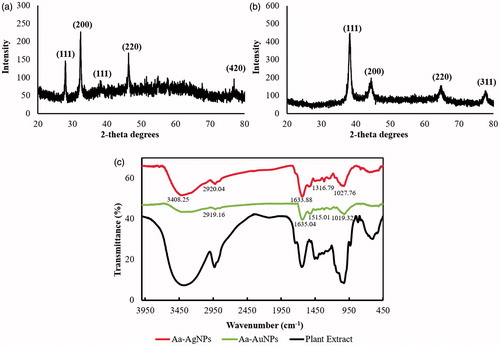
For Aa-AgNPs, FTIR analysis indicated prominent bands at 3408.25, 2920.04, 1633.88, 1316.79 and 1027.76 cm−1, whereas for Aa-AuNPs, bands were observed at 2919.16, 1635.04, 1515.01 and 1019.32 cm−1 (). –N–H and OH–O stretching create peaks between 3500 and 3000 cm−1. Characteristic peak at 1633.85 cm−1 indicates amide one bond of the polypeptides [Citation18]. Wavenumbers 1650–1450 cm−1 reveals the presence of an aromatic ring and elongation of the aromatic C=C bonds. The peaks between 1100 and 1010 cm−1 represent C–O and C–H chemical bonds [Citation19]. The peaks in the range of 1500 and 1400 cm−1 are attributed to the deformation vibration of the C–C bonds in the phenolic groups [Citation20]. FTIR analysis of the Aa-AgNP uncovers that proteins and other aromatic biomolecules might be responsible for its synthesis and stabilization, whereas phenols could be the likely key players involved in the conversion of HAuCl4⋅3H2O to Aa-AuNPs and also aids in maintaining the stability of Aa-AuNPs.
Cytotoxicity and anticancer potential of Aa-Au/AgNPs
In-vitro cytotoxicity of Aa-Ag/AuNPs was evaluated against 3T3-L1, A549, HT29 and MCF7 cells. Also, Aa-AgNPs were compared with cAgNPs in cancer cells. Results showd that Aa-AuNPs exhibited no cytotoxicity in 3T3-L1, HT29 and MCF7 cell lines up to 50 μg.mL−1 (). However, A549 cells were found to be sensitive to Ag-AuNPs treatment from 50 μg.mL−1 (). Further evaluation of Aa-AgNPs treatment showed that 3T3-L1 cell viability was significantly decreased from 50 μg.mL−1 concentration (). Previously, eco-friendly Aa-AgNPs have been reported to induce higher toxicity in cancer cells than other cAgNPs [Citation21]. Thus, comparison of Aa-AgNPs and cAgNPs was done. As expected, Aa-AgNPs induced a higher toxicity to A549, HT29 and MCF7 than the cAgNPs (). Results also exhibit that MCF7 cells were more sensitive to Aa-AgNPs treatment followed by HT29 and A549 cell lines. Previous studies have shown that Aa-AuNP does not exhibit a significant cytotoxic potential to cancer cells and is suggested to be used for drug delivery or imaging [Citation22]. This result opens the opportunity for Aa-AuNPs to be used to treat cancer lines since A549 lung cancer cells shown to be more sensitive to the treatment than normal cells. Thus, further evaluation of oxidative stress and cell migration were carried out for both, Aa-Au/AgNPs.
Figure 6. Cytotoxicity of Gold (Aa-AuNPs). Cell viability of (a) 3T3-L1, Pre-adipocyte cells; (b) A549, lung cancer cells; (c) HT29, colon cancer cells; (d) MCF7, breast cancer cells was measured after 48 h of treatment by MTT assay. Data are shown as mean ± SEM (n = 3). *p < .05, **p <.01 and ***p < .001 vs. Control.
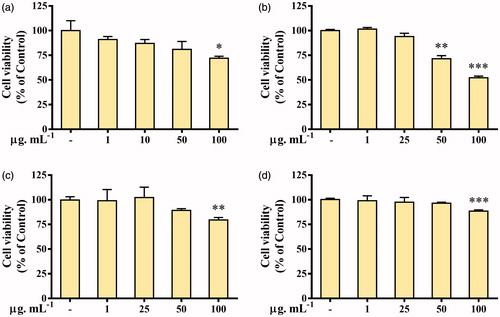
Figure 7. Cytotoxicity of green Silver (Aa-AgNPs) Nanoparticles and commercial Silver (cAgNPs) Nanoparticles. Cell viability was measured after 48 h of treatment by MTT assay in (a) 3T3-L1, Pre-adipocyte cells; (b) A549, lung cancer cells; (c) HT29, colon cancer cells; (d) MCF7, breast cancer cells. Data are shown as mean ± SEM (n = 3). *p < .05, **p < .01 and ***p < .001 vs. Control. ###p < .001 Aa-AgNPs vs. cAgNPs.
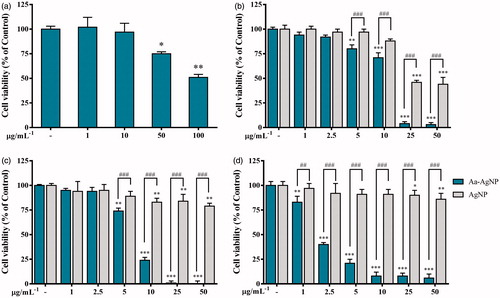
Reactive oxygen species (ROS) levels have been used as an indicator of oxidative stress in vitro studies and its redox status has been found to differ between normal and cancer cells [Citation23]. It is known that the regulation of oxidative stress is of importance during anticancer therapies. Also, studies have shown that high levels may constitute a barrier to tumourigenesis and DNA damage to cancer cells [Citation24]. In this study, evaluation of ROS levels in A549, HT29 and MCF7 in the presence of Aa-AuNPs treatment shown that ROS generation was not modified (data not shown). On the other hand, Aa-AgNPs significantly increased ROS levels in A549 cells than the cAgNPs treatment (), HT29 response to the treatment was only significant for Aa-AgNPs at the highest concentration, indicating that oxidative stress might not be a key during Aa-AgNPs treatment in colon cancer cells (). shows that cAgNPs treatment enhanced ROS levels in a dose-dependent manner when Aa-AgNPs only enhanced these levels at 5 μg.mL−1 in MCF7 cell line. Due to the high sensitivity of MCF7 cells to Aa-AgNPs treatment during the cytotoxicity test, it could be concluded that there were no increased ROS levels from 10 μg.mL−1 as there was no activity from the cells. Previous studies have shown the potential of Aa-AgNPs to induce oxidative stress in cancer cells, especially those synthesized from plant extracts [Citation25,Citation26]. Therefore, it is suggested that the potential of Aa-AgNPs to induce ROS generation in A549 and MCF7 cells might be responsible for their cytotoxic effect and may be an indicator of the anticancer effect of Aa-AgNPs in these two cell lines.
Figure 8. Oxidative stress potential of green Silver (Aa-AgNPs) Nanoparticles and commercial Silver (cAgNPs) Nanoparticles. Reactive oxygen species generation was measured after 48 h of treatment in (a) A549, (b) HT29 and (c) MCF7 cells. Data are shown as mean ± SEM (n = 3). *p < .05, ***p < .001 vs. Control. ###p < .001 Aa-AgNPs vs. cAgNPs.
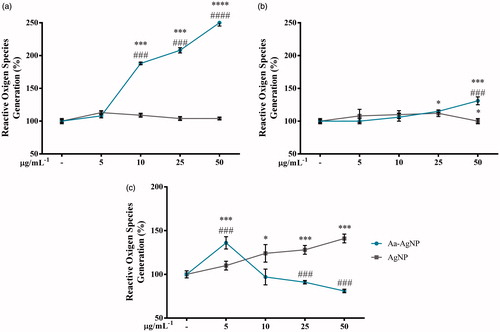
During the treatment, it has been found that one of the complications that interfere with the success of the therapy is metastasis. Since metastasis allows the growth of secondary tumours through the migration of cancer cells to other organs, the evaluation of the anti-migratory profile of a new drug is required to guarantee the success during the cancer therapy [Citation27]. Previous studies have found that A549 lung cancer cells in the presence of EGF during the wound-healing assay work an excellent model for cell migration evaluation [Citation28]. However, the concentration of the cell in the wound-healing assay was significantly lower than that of the control group (). The anti-migration potential of eco-friendly Aa-AgNPs has already been reported [Citation11,Citation21]. Fascinatedly, in this study for the first time it was found a Aa-AuNP that exhibits not only the potential to induce higher toxicity to cancer cells than normal cells, but also can reduce the migration of cancer cells. Thus, Aa-Au/AgNPs might have the anticancer potential to be used during the treatment of lung cancer therapy.
Figure 9. Aa-AgNPs reduces EGF-enhanced migration of A549 lung cancer cells. (a) Gold (Aa-AuNPs) Nanoparticles and (b) Silver (Aa-AgNPs) Nanoparticles analysis of migration ratio (%) within 24 h in wound healing assay. Data are shown as mean ± SEM (n = 3). ###p < .01 vs. Control. **p < .01 vs. EGF-Control.
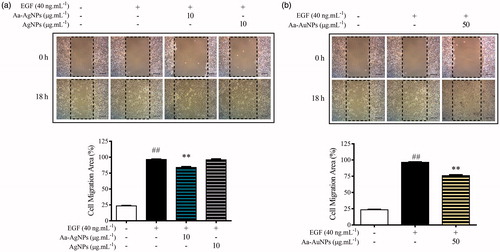
Discussion
Cancer is one of the leading and most incurable diseases worldwide. Among all types of cancers, lung, breast and colon cancers are included in the major types of cancer-related mortality. In 2016, 1,685,210 new cancer cases were projected in the USA by the National Cancer Institute, the Centres for Disease Control and Prevention and the North American Association of Central Cancer Registries. Also, the death rates were reported to increase due to liver cancer and colon cancer is expected to constitute about 8% of deaths due to cancer in the USA [Citation29]. Therefore, the discovery of a cure is of great need. So far, many studies showed how successful various anticancer drugs are to induce toxicity [Citation30], however, the poor specificity had led to the induction of bone marrow suppression, gastrointestinal tract lesions, hair loss, nausea and the development of clinical resistance since cytotoxicity agents act on cancer cells as well as healthy cells [Citation31,Citation32]. Thus, new approaches are constantly emerging in the pharmacological research area.
Over several decades, noble metallic NPs have induced incredible attention in the researchers and hence became the subject of extensive research. Owing to significant properties and remarkable applications of metal NPs, the scientific community has focused on the sensible synthetic techniques [Citation33–35]. Of late, the idea of using metal NPs in the medical field is of great interest, largely due to the constant search for an alternative to chemotherapy and radiotherapy owing to their devastating side effects [Citation34]. Thus, various experimental approaches often categorized as physical and chemical techniques that have been revealed for the synthesis of metal NPs, but unfortunately, ultimately lead to the significant ecological threat. Consequently, green methodologies are always considerable over the use of hazardous chemicals and toxic by-products corresponding to physiochemical techniques.
Nontoxic and available reducing agents for the synthesis of NPs, such as natural plants have opened up new avenues to eco-friendly, simple, rapid and cheap methodologies with biocompatible, scalable and medically applicable nature [Citation36]. To the date, the use of the various medicinal plants to the development of NPs is on focus since not only are used as a reducing agent but also, by creating a capping during the synthesis process some of the pharmacological properties of these plants are transferred. Panax ginseng (P. ginseng) root extract was used for the development of two potential antibacterial and antimicrobial metal NPs [Citation12] and leaves of P. ginseng and Dendropanax morbifera were next used for the synthesis of gold and silver nanoparticles that exhibited similar properties and anticancer properties [Citation37,Citation38]. Similar studies opened a new era of NPs, one that allows the use of plants, such as Pleuropterus multiflorus [Citation21], Cardamom fruits [Citation16], Acanthopanacis cortex [Citation39], Chaenomeles sinensis [Citation40], Prunus serrulata [Citation41] and other plants, that despite their numerous beneficial properties, its usage is limited to specific areas in which are endemic to. The use of the above plants and Anemarrhena asphodeloides, which is used in this study ( and ), have successfully given as a result the synthesize of a variety of metal NPs that exhibited low toxicity to normal cells but at the same time induce toxicity to cancer cells. Furthermore, Aa-AgNPs and Eleutherococcus senticosus mediated Aa-AgNPs induced oxidative stress better than a cAgNPs (, [Citation42]). In addition, Aa-AgNPs and AgNPs from Dendropanax morbifera and P. ginseng leaves showed the ability to reduce cell migration in EGF-enhanced A549 lung cancer cells at concentrations from 10 to 25 µg.mL−1 (, [Citation11,Citation43]). These results in addition to the previous reports that linked the activation of Caspase-3/MAPK pathways as main regulators of the apoptotic effect of the phyto-mediated synthesized Aa-AgNPs [Citation11,Citation42], suggest that the synthesis method used for the creation of Aa-NPs synthesized in this study just led to the creation of a new promising anticancer agent as it was done in previous studies.
Conclusion
This study demonstrates a facile, straight-forward, efficient and eco-friendly route for the biological synthesis of Aa-AgNPs and Aa-AuNPs from the Oriental medicinal plant,
A. asphodeloides. FE-TEM indicated Aa-Ag/AuNPs are mostly spherical and XRD revealed their crystallinity. Most importantly, FTIR analyses unravelled that majorly proteins and other aromatic compounds of A. asphodeloides might be responsible for the synthesis and for maintaining stability of Aa-AgNPs, whereas phenols are most likely involved in the formation and stabilization of Aa-AuNPs. In-vitro cytotoxicity analysis by MTT indicated that both Aa-Au/AgNPs do not exhibit significant toxicity to normal 3T3-L1 pre adipocyte cell line. On contrary, A549 cell line was the most sensitive to Aa-AuNPs treatment and MCF7 cell line to Aa-AgNPs treatment. Also, Aa-AuNPs had shown the potential to be used as anticancer agent during lung cancer treatment. Additionally, Aa-AgNPs exhibited the potential to be used not only to induce oxidative stress but also to inhibit cell migration. Further experiments are required to confirm the plausible anti-cancer effect and the mechanism associated with it for both, Aa-Au/AgNPs. Finally, this study would enable wide-spread utilization of the functional Aa-AgNPs and Aa-AuNPs, which indirectly promotes the usage of the pharmacologically active, endemic A. asphodeloides.
Disclosure statement
The authors declare that they have no conflict of interest.
Additional information
Funding
References
- Iida Y, Oh KB, Saito M, et al. Detection of antifungal activity in Anemarrhena asphodeloides by sensitive BCT method and isolation of its active compound. J Agric Food Chem. 1999;47:584–587.
- Takeda Y, Togashi H, Matsuo T, et al. Growth inhibition and apoptosis of gastric cancer cell lines by Anemarrhena asphodeloides Bunge. J Gastroenterol. 2001;36:79–90.
- Kim JY, Shin JS, Ryu JH, et al. Anti-inflammatory effect of anemarsaponin B isolated from the rhizomes of Anemarrhena asphodeloides in LPS-induced RAW 264.7 macrophages is mediated by negative regulation of the nuclear factor-κB and p38 pathways. Food Chem Toxicol. 2009;47:1610–1617.
- Hoa N, Phan D, Thuan N, et al. Insulin secretion is stimulated by ethanol extract of Anemarrhena asphodeloides in isolated islet of healthy Wistar and diabetic Goto-Kakizaki Rats. Exp Clin Endocrinol Diabetes. 2004;112:520–525.
- Kim HS, Song JH, Youn UJ, et al. Inhibition of UVB-induced wrinkle formation and MMP-9 expression by mangiferin isolated from Anemarrhena asphodeloides. Eur J Pharmacol. 2012;689:38–44.
- Pérez ZEJ, Mathiyalagan R, Markus J, et al. Ginseng-berry-mediated gold and silver nanoparticle synthesis and evaluation of their in vitro antioxidant, antimicrobial, and cytotoxicity effects on human dermal fibroblast and murine melanoma skin cell lines. Int J Nanomedicine. 2017;12:709.
- Mathiyalagan R, Subramaniyam S, Kim YJ, et al. Ginsenoside compound K-bearing glycol chitosan conjugates: synthesis, physicochemical characterization, and in vitro biological studies. Carbohydr Polym. 2014;112:359–366.
- Wong KK, Liu X. Silver nanoparticles—the real “silver bullet” in clinical medicine? Med Chem Commun. 2010;1:125–131.
- Ghosh P, Han G, De M, et al. Gold nanoparticles in delivery applications. Adv Drug Deliv Rev. 2008;60:1307–1315.
- Singh P, Kim YJ, Zhang D, et al. Biological synthesis of nanoparticles from plants and microorganisms. Trends Biotechnol. 2016;34:588–599.
- Castro-Aceituno V, Ahn S, Simu SY, et al. Anticancer activity of silver nanoparticles from Panax ginseng fresh leaves in human cancer cells. Biomed Pharmacother. 2016;84:158–165.
- Singh P, Kim YJ, Wang C, et al. The development of a green approach for the biosynthesis of silver and gold nanoparticles by using Panax ginseng root extract, and their biological applications. Artif Cells Nanomed Biotechnol. 2016;44:1150–1157.
- Markus J, Mathiyalagan R, Kim YJ, et al. Intracellular synthesis of gold nanoparticles with antioxidant activity by probiotic Lactobacillus kimchicus DCY51 T isolated from Korean kimchi. Enzyme Microb Technol. 2016;95:85–93.
- Gebäck T, Schulz M, Koumoutsakos P, et al. TScratch: a novel and simple software tool for automated analysis of monolayer wound healing assays. Biotechniques. 2009;46:265–274.
- Abbai R, Mathiyalagan R, Markus J, et al. Green synthesis of multifunctional silver and gold nanoparticles from the oriental herbal adaptogen: Siberian ginseng. Int J Nanomedicine. 2016;11:3131.
- Soshnikova V, Kim YJ, Singh P, et al. Cardamom fruits as a green resource for facile synthesis of gold and silver nanoparticles and their biological applications. Artif Cells Nanomed Biotechnol. 2017;46:108–117.
- Huo Y, Singh P, Kim YJ, et al. Biological synthesis of gold and silver chloride nanoparticles by Glycyrrhiza uralensis and in vitro applications. Artif Cells Nanomed Biotechnol. 2017;46:303–312.
- Hromiš NM, Lazić VL, Markov SL, et al. Optimization of chitosan biofilm properties by addition of caraway essential oil and beeswax. J Food Eng. 2015;158:86–93.
- Oladoja N, Alliu Y, Ofomaja A, et al. Synchronous attenuation of metal ions and colour in aqua stream using tannin–alum synergy. Desalination. 2011;271:34–40.
- Özacar M, Soykan C, Şengi̇ İA. Studies on synthesis, characterization, and metal adsorption of mimosa and valonia tannin resins. J Appl Polym Sci. 2006;102:786–797.
- Castro-Aceituno V, Abbai R, Moon SS, et al. Pleuropterus multiflorus (Hasuo) mediated straightforward eco-friendly synthesis of silver, gold nanoparticles and evaluation of their anti-cancer activity on A549 lung cancer cell line. Biomed Pharmacother. 2017;93:995–1003.
- Hainfeld JF, Smilowitz HM, O’Connor MJ, et al. Gold nanoparticle imaging and radiotherapy of brain tumors in mice. Nanomedicine (Lond). 2013;8:1601–1609.
- Sosa V, Moliné T, Somoza R, et al. Oxidative stress and cancer: an overview. Ageing Res Rev. 2013;12:376–390.
- Vandamme M, Robert E, Lerondel S, et al. ROS implication in a new antitumor strategy based on non‐thermal plasma. Int J Cancer. 2012;130:2185–2194.
- Han JW, Gurunathan S, Jeong JK, et al. Oxidative stress-mediated cytotoxicity of biologically synthesized silver nanoparticles in human lung epithelial adenocarcinoma cell line. Nanoscale Res Lett. 2014;9:459.
- Saini P, Saha SK, Roy P, et al. Evidence of reactive oxygen species (ROS) mediated apoptosis in Setaria cervi induced by green silver nanoparticles from Acacia auriculiformis at a very low dose. Exp Parasitol. 2016;160:39–48.
- Steeg PS. Targeting metastasis. Nat Rev Cancer. 2016;16:201–218.
- Liu N, Li Y, Su S, et al. Inhibition of cell migration by ouabain in the A549 human lung cancer cell line. Oncol Lett. 2013;6:475–479.
- Siegel R, Ma J, Zou Z, et al. Cancer statistics, 2014. CA Cancer J Clin. 2014;64:9–29.
- Bartlett JB, Dredge K, Dalgleish AG. The evolution of thalidomide and its IMiD derivatives as anticancer agents. Nat Rev Cancer. 2004;4:314.
- Love RR, Leventhal H, Easterling DV, et al. Side effects and emotional distress during cancer chemotherapy. Cancer. 1989;63:604–612.
- Griffin A, Butow P, Coates A, et al. On the receiving end V: patient perceptions of the side effects of cancer chemotherapy in 1993. Ann Oncol. 1996;7:189–195.
- Haeri A, Osouli M, Bayat F, et al. Nanomedicine approaches for sirolimus delivery: a review of pharmaceutical properties and preclinical studies. Artif Cells Nanomed Biotechnol. 2017. DOI: 10.1080/21691401.2017.1408123
- Allhoff F. The coming era of nanomedicine. Ethics and emerging technologies. Berlin: Springer; 2014. p. 155–66.
- Usmani A, Mishra A, Ahmad M. Nanomedicines: a theranostic approach for hepatocellular carcinoma. Artif Cells Nanomed Biotechnol. 2017;46:680–690.
- Iravani S. Green synthesis of metal nanoparticles using plants. Green Chem. 2011;13:2638–2650.
- Singh P, Kim YJ, Yang DC. A strategic approach for rapid synthesis of gold and silver nanoparticles by Panax ginseng leaves. Artif Cells Nanomed Biotechnol. 2016;44:1949–1957.
- Wang C, Mathiyalagan R, Kim YJ, et al. Rapid green synthesis of silver and gold nanoparticles using Dendropanax morbifera leaf extract and their anticancer activities. Int J Nanomedicine. 2016;11:3691.
- Ahn S, Singh P, Jang M, et al. Gold nanoflowers synthesized using Acanthopanacis cortex extract inhibit inflammatory mediators in LPS-induced RAW264. 7 macrophages via NF-κB and AP-1 pathways. Colloids Surf B Biointerfaces. 2017;160:423–428.
- Oh KH, Soshnikova V, Markus J, et al. Biosynthesized gold and silver nanoparticles by aqueous fruit extract of Chaenomeles sinensis and screening of their biomedical activities. Artif Cells Nanomed Biotechnol. 2017;46:599–606.
- Singh P, Ahn S, Kang JP, et al. In vitro anti-inflammatory activity of spherical silver nanoparticles and monodisperse hexagonal gold nanoparticles by fruit extract of Prunus serrulata: a green synthetic approach. Artif Cells Nanomed Biotechnol. 2017. DOI: 10.1080/21691401.2017.1408117
- Kim CG, Castro-Aceituno V, Abbai R, et al. Caspase-3/MAPK pathways as main regulators of the apoptotic effect of the phyto-mediated synthesized silver nanoparticle from dried stem of Eleutherococcus senticosus in human cancer cells. Biomed Pharmacother. 2018;99:128–133.
- Aceituno VC, Ahn S, Simu SY, et al. Silver nanoparticles from Dendropanax morbifera Léveille inhibit cell migration, induce apoptosis, and increase generation of reactive oxygen species in A549 lung cancer cells. In Vitro Celldevbiol-Animal. 2016;52:1012–1019.