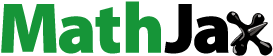
Abstract
The present study was undertaken to evaluate the synergistic effect of combining hyperthermia with irradiation and calcium carbonate nanoparticles (CC NPs) on proliferation of MCF-7 cells. The cells were randomly allocated to 19 groups: one negative control, three positive controls and 15 treatment groups. MCF-7 cells were treated with three concentrations of CC NPs (50, 100 and 150 μg/mL), gamma radiation (200 cGy), hyperthermia (41 °C for 1 h) and three concentrations of doxorubicin (200, 400 and 800 nm) and incubated at 37 °C for 24 h. Then the cell viability, the percentage of apoptosis and the levels of caspase-3, -8 and -9 proteins were measured. The results indicated that the combination group (150 µg/mL CC NPs + thermoradiotherapy) had a significant (p < .001) decrease in cell viability (48.65 ± 4.8%) and a significant (p < .001) increase in apoptosis percentage (45 ± 1.63%) of MCF-7 cells, as compared with the negative control and most of the other treatment groups. Moreover, a significant (p < .05) increase was observed in the activity of caspase-3 and caspase-9. Our findings revealed that CC NPs in combination with irradiation and hyperthermia could significantly reduce the cell viability and enhance the apoptosis of the MCF-7 breast cancer cells, the same as doxorubicin anti-cancer drug.
Introduction
Breast cancer remains one of the major clinical challenges throughout the world [Citation1]. Radiotherapy is used as a primary treatment or in combination with surgery, chemotherapy or hormone therapy [Citation2]. It has been estimated that virtually 50% of all cancer patients are candidates for radiation therapy during their course of disease [Citation3]. Radiation therapy is considered as a mandatory treatment for the patients undergoing conservative surgery and is appropriate for females with a high risk of recurrence of breast cancer after mastectomy [Citation4].
During the past decades, new strategies such as hyperthermia, immunotherapy, photodynamic therapy (PDT), laser treatment, gene therapy, nanoparticle therapy and angiogenesis inhibitors treatment have been proposed for cancer treatment. However, most of these strategies still require optimization, and in some cases, such as hyperthermia, PDT and nanoparticle therapy, new and improved equipment is needed [Citation5].
Over the last two decades, hyperthermia has been indicated to be effective in cancer treatments when combining with radiation therapy and/or chemotherapy [Citation6]. Of note, the radiosensitivity and thermosensitivity of the cells vary with the phase of the cell cycle. Hence, hyperthermia can be considered as a complement to radiotherapy [Citation7].
Recently, nanocarriers have received considerable attention in medicine. Due to their unique characteristics and features, they are used as the most specific method in the diagnosis and treatment of cancer. Another advantage of the nanoparticle delivery system is its ability to protect the drug component from premature degradation [Citation8].
In radiotherapy, combination of nanoparticles with ionizing radiation, as a radio-sensitizer, improves the contrast between cancerous and normal tissues by increasing local radiation dose. Delivery of high atomic number (Z) and/or high electron density nanoparticles to tumour cells can enhance the ability of radiations to kill tumour cells through increasing the probability of interactions between nanosized objects and ionizing radiations [Citation9]. The new strategy of combining radiation therapy with dose enhancement agents, such as nanoparticle radio-sensitizers, may be useful to minimize non-target radiation damage and to reduce the risk of secondary cancer development in healthy tissues [Citation10].
Calcium carbonate nanoparticles (CC NPs) are among novel inorganic materials with high potentials in biomedical applications. CC NPs can act as carriers for the control delivery system because they are naturally biodegradable, biocompatible and porous. Furthermore, CC NPs have turntable physicochemical properties and surface chemistry (shape and size) and can easily be produced on a large scale [Citation11]. Another merit of these nanoparticles is their high sensitivity to pH; their solubility increases exponentially with pH decrease. These characteristics of CC NPs give them a potential role in cancer treatment in future [Citation11].
Based on literature review, there is no published data on the role of CC NPs as potential radio-sensitizers; therefore, we decided to investigate the synergistic effect of combining hyperthermia with irradiation and CC NPs on the proliferation of human breast adenocarcinoma cell line (MCF-7 cells).
Materials and methods
CC NPs were purchased from the Iranian Nanomaterials Pioneers Company, Nanosany (Mashhad, Iran). Propidium iodide, acridine orange, phosphate buffered saline (PBS) and 3-[4,5-dimethylthiazol-2-yl]-2,5-diphenyltratrazolium bromide (MTT) bromide were procured from Sigma-Aldrich (St. Louis, MO). Fetal bovine serum and Dulbecco’s Modified Eagle’s Medium (DMEM) were obtained from GIBCO/Life Technologies Inc. (Carlsbad, CA). Caspase-3, -8 and -9 protease activities were determined using Biovision kits (BioVision Inc., Milpitas, CA).
Cell culture
MCF-7 cell line was obtained from National Cell Bank of Iran (Pasteur Institute of Iran, Tehran). Cancerous cells were seeded in a DMEM-containing flask. The medium used was adjusted to contain 10% fetal bovine serum. The cells were incubated at 37 °C in a 5% CO2 atmosphere. Following the incubation time for 24 h, the attached cells were trypsinized for 3–5 min and centrifuged at 1400 rpm for 5 min. The cells were then counted and transferred into a 24-well plate; each well contained 1 × 106 cells. Subsequently, the plate was incubated (in a 5% CO2 at 37 °C for 24 h) to allow the cells to attach to the bottom of the wells [Citation12].
Experimental design
The Ethical Committee of our university (approval number: IR.UMSU.REC. 62–1639/2015) approved all the experimental procedures performed in this study. The MCF-7 cells were suspended in DMEM, cultured in a plate and randomly allocated to 19 groups: one negative control (NC; untreated cells), 15 treatment groups, and three positive controls using doxorubicin at concentrations of 200, 400 and 800 nM, respectively. shows the characteristics of the treatment and control groups.
Table 1. Characteristics of the groups under study.
Treatment groups were divided into single-agent treatment and multi-agent treatment groups. The former included five groups consisting of radiation (R), hyperthermia (H) and CC NPs at three concentrations (50, 100 and 150 μg/mL) alone. The latter, which was obtained from the combination of single-agent treatment groups consisted of 10 groups, including a combination of radiation and hyperthermia with different concentrations of CC NPs.
Preparation of CC NPs for in vitro experiments
CC NPs were exposed to an UV illumination for 30 min to prevent bacterial and endotoxin contamination. A 6-W, UV-C lamp with the length of 30 cm was installed 5 cm above the samples surface. The maximum emission wavelength of light emitted from the lamp was 254 nm. The light intensity was measured by a radiometer at 200–400 nm (Hagner ECL-X), which was 1.8 W/m2 [Citation13]. The CC NPs were suspended in a cell culture medium and diluted to appropriate concentrations (50, 100 and 150 μg/mL). Before the exposure of the cells to nanoparticles, the CC NP dilutions were sonicated using a sonicator bath (UP200H/Hielscher, Germany) at 40 W and 24 kHz frequency at room temperature for 15 min, and after that, the cells were treated [Citation14].
Measurement of nanoparticle uptake
The MCF-7 cells were suspended in DMEM to reach the final concentration of 5 × 104/mL and were incubated separately with the serial concentrations of CC NPs (50, 100 and 150 μg/mL) in 5% CO2 at 37 °C for 24 h. Next, the treated cells were washed three times with PBS to remove non-uptake nanoparticles. Cells were then digested with 0.3 ml of 3% HCl in concentrated HNO3 at room temperature overnight. After the addition of 5 μL of 5-ppm indium (as the internal standard) and 5 ml of matrix solution (2% HCl and 2% HNO3), the calcium carbonate (CaCO3) content of each sample was measured by an atomic absorption spectrometer (wavelength 280 nm, Model 603, Perkins Elmer, Waltham, MA) and compared with the standard curve [Citation15].
Treatment with CC NPs and doxorubicin
In the single-agent treatment groups of CC NPs and doxorubicin, MCF-7 cells were seeded, and after 1 day, the cells were treated with different concentrations of CC NPs and also with doxorubicin as positive control (PC1 = 200, PC2 = 400 and PC3 = 800 nm) groups for 24 h. Then the supernatant containing CC NPs and doxorubicin was removed, and the cells were washed three times with PBS. After that, the cells were incubated in a 5% CO2 atmosphere at 37 °C for 1 day, prior to harvesting the cells to carry out different assays.
Exposure to ionizing radiation
For gamma radiation exposures, the cells were removed from the 37 °C incubator and exposed to a clinically relevant dose (200 cGy) of a cobalt-60 γ-ray source (Theratron Phoenix, Theratronics, Inc., Ottawa, Canada) at room temperature (22 °C) with an approximate dose rate of 34 cGy/min (source to skin distance [SSD] of 79.5 cm). Immediately after exposures, the cultures were returned to the 37 °C incubator and harvested after 24 h for performing different assays [Citation16].
Hyperthermic exposure
The culture medium of MCF-7 cells was seeded in each well of 24-well plates and pre-cultured at 37 °C overnight. Hyperthermic exposure was then performed by transferring the culture plates into a cell culture incubator (SB-14012, Germany), which maintained at 41 °C for 1 h for all experiments [Citation12]. In this system, temperature can be automatically monitored with the accuracy of 0.1 °C; however, the temperature was double-checked with a high-quality, calibrated thermometer.
In the thermoradiotherapy (R + H) group, the cells plate was exposed to γ-ray after the hyperthermia process. In both H and R + H groups, the cells were incubated in a 5% CO2 atmosphere at 37 °C for 24 h, before the cells to be harvested for doing different assays.
Multi-agent treatments
In R + H group, MCF-7 cells were cultured for 1 day, then incubated at 41 °C for 1 h and immediately irradiated by a relevant dose (200 cGy) of cobalt-60 γ-ray source.
In CC NPs + H groups, the same procedure used for the single-agent treatment groups of CC NPs was followed. After washing with PBS, the cells were incubated at 41 °C for 1 h.
In treatment groups CC NPs + R, we followed a similar procedure provided for single-agent treatment groups of CC NPs. After rinsing with PBS, the cells were irradiated by a cobalt-60 γ-ray source (200 cGy) [Citation16].
In CC NPs + H + R combination treatment group, a similar procedure to that in CC NPs + H group was applied. Immediately after hyperthermia, the cells were irradiated by a relevant dose (200 cGy) of cobalt-60 γ-ray source.
In all these multi-agent combination treatment groups, the cells were finally incubated in a 5% CO2 atmosphere at 37 °C for 1 day, prior to harvesting the cells for assessment.
Treatment analysis
MTT assay
The cytotoxicity in the treated groups against the MCF-7 cell lines was examined by the MTT assay method [Citation17,Citation18]. The MCF-7 cells (1 × 106 cells/well) were seeded on a 96-well plate in a minimum essential medium containing fetal bovine serum. The cells were incubated during the night for attachment to the plate surface. The cells in the wells were exposed to various agents () and incubated in 5% CO2 at 37 °C for 24 h. The cells were subsequently treated with MTT (Sigma Chemical Co., St. Louis, MO) and incubated at 37 °C for 4 h until a purple-coloured formazan product was developed. After 4 h, the entire medium containing MTT solution (5 mg/mL) was aspirated from the wells. The remaining formazan crystals were dissolved in dimethyl sulfoxide, and the absorbance or optical density (OD) was measured at 570 nm using a 96-well microplate reader (Bio-Rad, Hercules, CA). Cell viability was calculated as follows [Citation19].
Evaluation of apoptosis of MCF-7 cells
The apoptosis of MCF-7 cells was evaluated by fluorescence microscopy. In brief, for preparation of the staining solution, 100 µl of propidium iodide and acridine orange (1 mg/mL each) was added to 10 mL PBS. MCF-7 cells suspensions were blended at a ratio of 1:1 with the staining solution in microtiter wells [Citation20]. The apoptotic cell percentage was determined in an improved Neubauer rhodium haemocytometer (Germany) under a fluorescent microscope (Olympus CKX41, UK) [Citation20].
Measurement of caspase-3, -8 and -9 activities
Proteolytic activities of caspase-3, -8 and -9 were measured by colorimetric assay kits (Sigma-Aldrich, St. Louis, MO) according to the instructions specified by the manufacturer. Briefly, the cells were seeded on a dish at a seeding density of 1 × 106 after becoming confluent, serum-containing medium was aspirated and co-cultured with different concentrations of CC/doxorubicin in a serum-free medium at 37 °C for 24 h. The cell pellets were suspended in a cell lysis buffer (50 mM of Tris-HCl [pH 7.4], 0.5% NP-40, 250 mM of NaCl, 5 mM of EDTA and 50 mM of NaF) and incubated on ice for 10 min. The lysate was vortexed every 15 min. After centrifugation at 11,000 rpm for 15 min, supernatants were collected. For measurement of the activities of caspase-3, -8 and -9, 20 µL of cell lysate was added immediately to the buffer composed of a p-nitroaniline (pNA)-conjugated substrate for caspase-3 (Ac-DEVD-pNA), -8 (Ac-IETD-pNA) and -9 (LEHD-pNlabeled). The samples were then incubated at 37 °C for 1 h. A microplate reader (SpectraFluor, TECAN, Sunrise, Austria) was used to detect the fluorescence. The released pNA concentration was calculated from the absorbance values at 405 nm, and caspases activities were determined as fold increase over the non-treated cell control [Citation21].
Statistical analysis
Data were expressed as mean ± standard deviation (SD) and were analysed using one-way ANOVA to make comparisons between the groups. Whenever the ANOVA analysis indicated significant differences, Tukey’s multiple comparison post-hoc test was performed to compare the mean values between the different groups. Differences between the groups were considered statistically significant if p values were < .05.
Results
Physicochemical characterization of CC NPs
shows the typical transmission electron microscopy (TEM) image of CC NPs, which exhibits the majority of particles are in a spherical shape with smooth surfaces. The average TEM diameter of CC NPs was 20 nm.
Measurement of CC NPs uptake
The uptake of CC NPs into the MCF-7 cells was examined using the quantitative spectrophotometric method. We observed a linear correlation (r2 > 0.96) between the absorbance (OD) and concentration of CC NPs in the culture medium (). Based on the standard curve, CC NPs uptake into the MCF-7 cells in concentration of 150 μg/mL was 59.038 μg/mL.
MTT results
The mean ± SD of ODs from the MTT test in the studied groups after 24-h incubation is listed in . The results of the MTT assay showed that the lowest ODs were associated with the PC3 and NP3 + R + H groups without any significant difference between them. This finding is correlated with the highest cytotoxicity in the mentioned groups.
Table 2. Mean ± standard deviations (SD) of optical densities and apoptosis in the groups under study.
The results of pairwise comparisons of cell viability between the studied groups are provided in . The results of the MTT assay with the aim of evaluating MCF-7 cell survival rates showed that the cell viability in CC NPs treatment groups of NP1, NP2 and NP3 decreased significantly with increasing the concentration of CC NPs from 50 to 150 μg/mL (). As seen in the figure, the highest cytotoxicity (lowest cell viability) groups were 150 μg/mL NPs + R + H and PC3, as compared to the other groups (). Although there was a reduction in the cell viability in the single-agent treatment (NP1 and H) groups relative to the NC group, no significant difference was observed in NP1 and H groups in comparison to NC group. The cell viability results also showed a significant difference between R group and both NC and H groups, as well as between the two-agent combined treatment groups (H + R, NP1 + H and NP1 + R) and NC, H and NP1, respectively. In addition, no significant difference was observed between R and NP1 groups, as well as between the above-mentioned two-agent combined treatment groups and R group ().
Figure 3. Effects of hyperthermia (H), radiation (R), calcium carbonate nanoparticles (NPs) and doxorubicin, as positive control (PC), on the cell viability of MCF-7 cells in the studied groups. The values were normalized to those of negative control group. The Figure abbreviations are provided in . The following groups had significant differences (p < .05) as follows: NC and H with the groups labelled (A). NP1 with the groups labelled (B). R, R + H, NP1 + H and NP1 + R with the groups labelled (C). NP2 with the groups labelled (D). NP1 + R + H, NP2 + R, NP2 + H, NP3 and NP2 + R + H with the groups labelled (E). NP3 + H, PC1 and NP3 + R with the groups labelled (F).
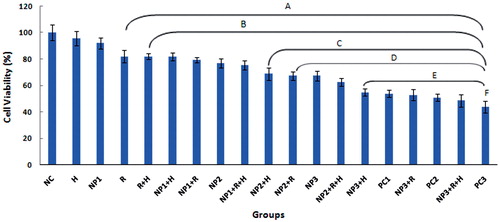
Quantification of apoptosis
The effects of hyperthermia, gamma radiation and CC NPs on the survival of MCF-7 cells were evaluated by propidium iodide/acridine orange staining. In this method, MCF-7 cells were categorized based on their colour and chromatin morphology. As shown in , the green cells (excluding propidium iodide) are viable with diffused chromatin, whereas the cells with condensed chromatin are apoptotic. Further, the red cells (including propidium iodide) with non-condensed chromatin are necrotic.
Figure 4. The assessment of MCF-7 apoptosis by propidium iodide/acridine orange staining. The green cells with diffused chromatin are viable (A), the red cells with no condensed chromatin are necrotic (B) and the cells with condensed chromatin are apoptotic (C).
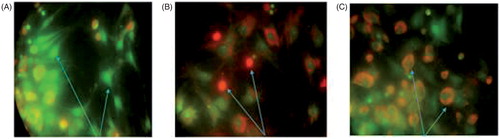
In the CC NPs-treated groups, cell apoptosis elevated with an increase in the concentration of NPs (from 50 to 150 μg/mL), indicating the concentration-dependent of apoptotic effects of CC NPs. However, apoptosis increase in the NP3 (150 μg/mL) group was significantly higher than the NP1 and NP2 groups ().
The rate of apoptosis of MCF-7 cells in R group was approximately similar to that of the CC NPs group of 50 μg/mL (NP1), but a significant difference was observed between these groups and H group. In addition, the apoptosis rate in the R + H group was significantly higher than the H group (). The apoptosis results also showed a significant difference between the groups treated with the medium and high concentrations of CC NPs in combination with radiotherapy (NP2 + R and NP3 + R) in comparison to NP2, NP3 and R groups alone. There was no significant difference between the combined treatment group (NP1 + R and NP1 + H) and single-agent treatment groups of NP1 and R, but a significant difference was observed in H group alone.
The highest apoptosis rate was found in the multi-agent treatment groups, NP3 + R + H, and PC3. Although there was no significant difference between these two groups, but a significant difference was observed between these two groups in comparison to other groups ().
Measurement of caspase-3, -8 and -9 activities
The activities of caspase-3, -8 and -9 were assessed to find out the possible biochemical mechanisms that led to apoptosis in the treated group. Assessment of caspase-3, -8 and -9 activities demonstrated that with increasing the concentration of CC NPs (from 50 to 150 μg/mL) in all the treatment groups, the activity of caspase-3 and -9 elevated significantly compared to the NC group (), suggesting that caspase-3 and -9 activities are dependent on CC NPs concentration. In H group, caspase-3 and -9 activities did not show any significant changes compared to the NC group (p > .05), but in the R group, the result was reversed (p < .05). The results also showed that the activity of caspase-8 protease had no significant change ().
Table 3. Mean ± standard deviations (SD) of caspase-3, -8, and -9 activities in the groups under study.
Discussion
We herein assessed the synergistic effect of combining hyperthermia with irradiation and CC NPs on the proliferation of MCF-7 cells. The results of the MTT assay showed that the cell viability decreased significantly with increasing the CC NPs concentration from 50 to 150 μg/mL. MTT results also demonstrated that the viability of MCF-7 cells was affected by the cytotoxic effects of CC NPs, indicating the concentration-dependent cytotoxic effects of CC NPs (). In line with our finding, Abdolmohammadi et al. [Citation22] have also demonstrated that the cell viability of the MCF-7 cell lines reduces with increasing ZnO nanofluid concentrations.
The results of MTT assay and the viability of MCF-7 cell lines in the multi-agent treatment groups showed that radiotherapy was more effective when combining with hyperthermia and nanoparticles than their use separately (). This observation could be related to the Chatterjee’s et al. [Citation6] finding that the loading tumours with systematically administered nanoparticles have an interesting effect on transduction of extrinsic energy sources, such as ionizing radiation and heat.
MTT results from the current study also showed that the treated group with doxorubicin 800 nM (PC3) had the most reduction in the cell viability compared to the other treatment groups, and there was not any significant difference between the PC3 and NP3 + R + H groups (). We observed that the viability of MCF-7 cell lines was highly influenced by the combination of temperature, radiation and CC NPs. In other words, the multi-agent combination treatment groups were more effective than treatment with any of them individually.
The results of acridine orange/propidium iodide staining with the aim of evaluating cell apoptosis revealed that all treated groups had MCF-7 apoptosis (), indicating that the single- and multi-agent treatments have ability to induce radiosensitivity in MCF-7 cells. Previous studies have also suggested that there is a direct link between apoptosis and radiosensitivity, i.e. in the presence of apoptosis, cells become radiosensitive, but in its absence, cells become radioresistant [Citation7,Citation23]. We found that the rate of apoptosis in the groups treated with different concentrations of CC NPs in combination with hyperthermia (NP1 + H, NP2 + H and NP3 + H) increased in comparison to other groups that received different concentrations of CC NPs (NP1, NP2 and NP3) alone. In addition, apoptosis increase in the combined group NP3 + H was statistically significant compared to NP3 group (p < .05).
Our results showed a close percentage of apoptosis in both the positive control (PC1, PC2 and PC3) groups and CC NPs (NP1, NP2 and NP3) groups, which displays a similar concentration-dependent mechanism for these both groups. This finding is in accordance with Liang et al.’s [Citation24] study in which apoptosis was observed to be increased with the elevation of doxorubicin dose. It should also be noted that there was a significant difference between the PC groups (). Comparing the percentage of apoptosis in the treated MCF-7 cells in groups NP1 + R + H, NP2 + R + H and NP3 + R + H with PC1, PC2 and PC3 demonstrated no significant difference between these two groups, respectively The highest percentage of apoptosis was identified in NP3 + R + H group, which was slightly higher than the PC3 group (). This synergistic effect could be due to high tumour temperature (hyperthermia) and CC NPS, as potent radio-sensitizers, during radiotherapy process. The most resistant phase of the cell cycle in radiotherapy (late S) is most sensitive to hyperthermia. Therefore, hyperthermia can be considered as a complement to radiotherapy [Citation7]. There is a temporal relationship between synergistic effect and thermoradiotherapy procedure. It has been reported that the use of hyperthermia before radiotherapy makes the outcome more effective than the reverse [Citation25]. In the current study, the cell plate was exposed to γ-ray immediately after the hyperthermia process. It has been indicated that the levels of reactive oxygen species (ROS) elevate after exposure to both lethal (≥42 °C) and non-lethal (40 °C) temperatures. This elevation mainly happens because of the increased generation of ROS such as superoxide and hydrogen peroxide (H2O2), most likely as the result of dysfunction of the mitochondrial respiratory chain [Citation6,Citation26]. In our study, more synergistic effects were observed when γ-ray was combined with hyperthermia and CC NPs for the better treatment of breast cancer.
During the irradiation process, free radicals are created via ionizing reactions that are effective in killing cancer cells by DNA damaging [Citation16]. Numerous studies have revealed that the increase of ROS is a mechanism by which nanoparticles and hyperthermia sensitize human breast cancer cell lines to the effects of irradiation [Citation6,Citation16,Citation26,Citation27]. It has also been found that ROS is produced from extracellular and intracellular sources [Citation28]. In the present study, the main intracellular source of ROS production is mitochondria, and the extracellular sources of ROS generation include ROS-inducing agents, such as CC NPs, heat and exposure to radiation. The mechanism related to nanoparticle-induced ROS production varies among different nanoparticles, and the cellular mechanism associated with ROS generation remains unexplained [Citation28]. It seems that increased accumulation of CC NPs in mitochondria may disrupt the mitochondrial electron-transfer chain as well as the high levels of ROS-mediated cytotoxicity.
ROS is a key player in mediation of oxidative stress, activation of the c-Jun-N-terminal kinase (JNK), up-regulation of Bax/Bcl-2 ratio, and damage to mitochondrial membrane potential. It is released from mitochondria by destruction of cytochrome c, and then the caspase-3/-9 cascade is activated [Citation29]. Taken together, we suppose that CC NPs alternatively induce antiproliferation effects in MCF-7 cells and that CC NPs-induced apoptosis is mediated through a ROS/JNK-dependent mitochondrial/caspase pathway.
Previous studies have demonstrated that the radiosensitizing ability of nanomaterials could be influenced by size, shape, concentration, surface coating, surface charge and radiation energy [Citation30,Citation31]. Zhang et al. [Citation30] have evaluated the radiosensitivity of different sizes of gold nanoparticles (GNPs) and concluded that GNPs of 12.1 and 27.3 nm can show higher radiosensitivity than GNPs with other sizes [Citation30]. It was interesting that we obtained a similar result when using one size of CC NPs (20 nm). The results of aforementioned studies confirm that nanoparticles can enhance the dose deposition phenomenon in nanoparticle-loaded tumours [Citation32,Citation33]. Jain et al. [Citation34] have reported that gold nanoparticles have radiosensitization effect at kilovoltage and megavoltage photon energies. However, there are controversial results on the effect of photon energy and nanoparticle size in the literature [Citation29,Citation35].
Our results revealed that the administration of CC NPs in tumour cells may be effective in mortality of tumour cells by increasing radiosensitization. This observation can be attributed to the improved effectiveness of ionizing radiation via increasing the interaction between the kilovoltage (keV) and megavoltage (MeV) photon beam (X- or γ-rays) energies and CaCO3 with the high effective atomic number (Zeff > 10) as compared to soft tissues (Zeff < 4). In this sense, the probability interaction of the photon beams in CaCO3 is higher than in soft tissues due to photoelectric absorption in the low-energy range and pair production process in the high-energy (megavoltage) range [Citation36,Citation37]. In addition, the higher electron density (Nel) of CaCO3 (>3 × 1023 electron/g) than that of soft tissues (>1.5 × 1023 electron/g) [Citation36,Citation37] leads to the predominance of Campton interaction phenomena in the Compton range of energies (keV and low MeV). Hence, the administration of CC NPs in tumour cells may be effective in radiation dose enhancement. In other words, tumour cells containing CC NPs may receive higher radiation dose than normal cells, meaning that CC NPs can raise the radio-sensitivity of tumour cells. Chithrani et al. [Citation33] have reported that more absorption of nanoparticles in the cells can increase the probability of radiation interactions inside the cells.
CC NPs have displayed to have a promising potential in the development of drug carriers, but little research has been conducted regarding their safe dosage for maximizing the therapeutic activity without harming the biosystems. The results of study by Zhang et al. [Citation38] have indicated that CC NPs may cause oxidative damage to HeLa cells. Furthermore, CC NPs are likely to have more appropriate degree of biocompatibility than other nanomaterials [Citation38]. Overall, CC NPs with higher effective electron density than soft tissues (enhancement of radiosensitization due to increase in the absorbed irradiation dose) and particular characteristics such as biocompatibility, slow biodegradability, low cost, safety, availability, etc. could be regarded as potential radio-sensitizer agents in radiotherapy in future.
Apoptosis results in our study showed that among the treatment groups, the NP3 + R + H and H were the highest and lowest significant apoptosis groups, respectively (). In moderate hyperthermia (temperature between 40 and 42 °C), similar to what was used in this study (41 °C), the temperature is non-lethal [Citation6], but it can be lethal if combined with other agents such as CC NPs and radiation. Our results also revealed that the apoptosis rate of combined group NP3 + R + H is the highest and is comparable to that of doxorubicin 800 nM (PC3) group.
Evaluation of caspase-8 activity demonstrated that there is no significant difference between the expression of caspase-8 in all treatment (single- and multi-agent treatments) groups and NC group (p > .05). In addition, the assessment of caspase-8 activity in PC1, PC2 and PC3 groups revealed that the activity of caspase-8 in the MCF-7 cell was not significantly different from that of the control group (p > .05). It means that the expression of caspase-8 is not dependent on doxorubicin concentration. In the combined groups of R + H with NP1, NP2 and NP3, the expressions of caspase-3 and -9 significantly increased in comparison to NC, as well as CC NPs + R, CC NPs + H and R + H groups. In addition, the activity of caspase-9 raised significantly with increasing doxorubicin concentration, but caspase-8 activity showed no significant change, as reported by Sharifi et al. [Citation39]. They have observed that the elevation of caspase-9 level is dependent on the doxorubicin dose, while caspase-8 level did not follow dose dependency pattern [Citation39].
The activity of caspase-3 (a marker for apoptosis) in PC3 group was significantly higher than the other groups. Meanwhile, the activity of caspase-9 (a marker for the mitochondrial pathway of apoptosis and ROS production) elevated significantly with increasing doxorubicin concentration. There was also no significant difference between PC3 group and the combined groups of R + H with different concentrations of CC NPs. On the other hand, the highest expressions of caspase-3 and -9 were found in PC3 and NP3 + R + H groups, but no significant difference was observed in the expression of caspase-9 between the two groups (). This finding suggests that the CC NPs-induced cell death through mitochondrial pathway is similar to doxorubicin.
Mammalian cells have at least two main pathways causing apoptosis: extrinsic death-receptor-dependent apoptosis and intrinsic mitochondrial-dependent apoptosis. In both of these apoptotic pathways, cell death induction is associated with caspases activation [Citation40]. In anticancer chemotherapy, the activation of caspases can be started when the extrinsic pathway is stimulated, or the intrinsic pathway is activated in the mitochondria. To induce apoptosis in tumour cells, doxorubicin, as a single agent, is applied in the first-line therapy of breast cancer. Doxorubicin increases the level of caspase-9, but not caspase-8, in a time- and dose-dependent manner [Citation35]. In two recent studies by Baharara et al. [Citation41,Citation42], it has been reported that the activities of caspase-3 and -9 increased in cells treated with silver nanoparticles and GNPs [Citation41,Citation42]. The results from the present study showed that despite an increase in caspase-8 protease activity, no significant change was observed. However, the activities of caspase-3 and caspase-9 raised significantly in all the treated groups. Therefore, our results confirm that CC NPs in combination with hyperthermia and radiotherapy induce mitochondrial-dependent apoptosis by the up-regulation of caspase-3 and -9 expressions. Our findings have consistency with a number of previous studies [Citation41–44] that have demonstrated that the up-regulation of caspase-3 and -9 in MCF-7 cells can be attributed to the NP-induced cell death through the mitochondrial pathway.
Our results indicated that the synergistic effect of combining hyperthermia, irradiation and CC NPs (NP3 + R + H) could be comparable with doxorubicin (P3), as an anti-cancer drug. In summary, the present work reveals that using CC NPs in combination with hyperthermia and radiation can have promising applications in the treatment of MCF-7 cancer cells.
Acknowledgements
Authors would like to acknowledge Solid Tumor Research Center of Urmia University of Medical Sciences (Urmia, Iran) for providing financial support to this project. The authors also specially thank the physicians and staff of the Omid Research and Treatment Center (Urmia, Iran) who helped in doing the present study.
Disclosure statement
The authors report they have no conflict of interest. The authors individually accept the responsibility for the content of this article.
References
- Burstein HJ, Temin S, Anderson H, et al. Adjuvant endocrine therapy for women with hormone receptor-positive breast cancer: American society of clinical oncology clinical practice guideline focused update. J Clin Oncol. 2014;32:2255–2269.
- Tutt A, Yarnold J. Radiobiology of breast cancer. Clin Oncol (R Coll Radiol). 2006;18:166–178.
- Mahmoudi R, Jabbari N, Aghdasi M, et al. Energy dependence of measured CT numbers on substituted materials used for CT number calibration of radiotherapy treatment planning systems. PLoS One. 2016;11:e0158828.
- Jagsi R. Progress and controversies: radiation therapy for invasive breast cancer. CA Cancer J Clin. 2014;64:135–152.
- Arruebo M, Vilaboa N, Sáez-Gutierrez B, et al. Assessment of the evolution of cancer treatment therapies. Cancers (Basel). 2011;3:3279–3330.
- Chatterjee DK, Diagaradjane P, Krishnan S. Nanoparticle-mediated hyperthermia in cancer therapy. Ther Deliv. 2011;2:1001–1014.
- Hall EJ, Giaccia AJ. Radiobiology for the radiologist. Philadelphia (US): Lippincott Williams & Wilkins; 2011.
- Jabir NR, Tabrez S, Ashraf GM, et al. Nanotechnology-based approaches in anticancer research. Int J Nanomedicine. 2012;7:4391–4408.
- Pottier A, Borghi E, Levy L. The future of nanosized radiation enhancers. Br J Radiol. 2015;88:20150171.
- Botchway SW, Coulter JA, Currell FJ. Imaging intracellular and systemic in vivo gold nanoparticles to enhance radiotherapy. Br J Radiol. 2015;88:20150170.
- Maleki Dizaj S, Barzegar-Jalali M, Zarrintan MH, et al. Calcium carbonate nanoparticles as cancer drug delivery system. Expert Opin Drug Deliv. 2015;12:1649–1660.
- Elengoe A, Hamdan S. Heat sensitivity between human normal liver (WRL-68) and breast cancer (MCF-7) cell lines. J Biotechnol Lett. 2013;4:45–50.
- Yang Y, Xie XY, Mei XG. Preparation and in vitro evaluation of thienorphine-loaded PLGA nanoparticles. Drug Deliv. 2016;23:787–793.
- Akhtar MJ, Ahamed M, Kumar S, et al. Zinc oxide nanoparticles selectively induce apoptosis in human cancer cells through reactive oxygen species. Int J Nanomedicine. 2012;7:845–857.
- Chung H, Choia b, Liangliang H, et al. Mechanism for the endocytosis of spherical nucleic acid nanoparticle conjugates. Appl Biol Sci. 2013;110:7625–7630.
- Chen F, Zhang XH, Hu XD, et al. The effects of combined selenium nanoparticles and radiation therapy on breast cancer cells in vitro. Artif Cells Nanomed Biotechnol. 2017;7:1–12.
- Florento L, Matias R, Tuaño E, et al. Comparison of cytotoxic activity of anticancer drugs against various human tumor cell lines using in vitro cell-based approach. Int J Biomed Sci. 2012;8:76–80.
- Beik J, Jafariyan M, Montazerabadi A, et al. The benefits of folic acid-modified gold nanoparticles in CT-based molecular imaging: radiation dose reduction and image contrast enhancement. Artif Cells Nanomed Biotechnol. 2017;2:1–9.
- Sadat Shandiz SA, Shafiee Ardestani M, Shahbazzadeh D, et al. Novel imatinib-loaded silver nanoparticles for enhanced apoptosis of human breast cancer MCF-7 cells. Artif Cells Nanomed Biotechnol. 2017;45:1–10.
- Subramani T, Yeap SK, Ho WY, et al. Vitamin C suppresses cell death in MCF-7 human breast cancer cells induced by tamoxifen. J Cell Mol Med. 2014;18:305–313.
- Gurunathan S, Park JH, Han JW, et al. Comparative assessment of the apoptotic potential of silver nanoparticles synthesized by Bacillus tequilensis and Calocybe indica in MDA-MB-231 human breast cancer cells: targeting p53 for anticancer therapy. Int J Nanomedicine. 2015;10:4203–4223.
- Abdolmohammadi MH, Fallahian F, Fakhroueian Z, et al. Application of new ZnO nanoformulation and Ag/Fe/ZnO nanocomposites as water-based nanofluids to consider in vitro cytotoxic effects against MCF-7 breast cancer cells. Artif Cells Nanomed Biotechnol. 2017;45:1769–1777.
- Rupnow BA, Murtha AD, Alarcon RM, et al. Direct evidence that apoptosis enhances tumor responses to fractionated radiotherapy. Cancer Res. 1998;58:1779–1784.
- Liang Y, Yan C, Schor NF. Apoptosis in the absence of caspase 3. Oncogene. 2001;20:6570–6578.
- Robinson JE, Wizenberg MJ. Thermal sensitivity and the effect of elevated temperatures on the radiation sensitivity of Chinese hamster cells. Acta Radiol Ther Phys Biol. 1974;13:241–248.
- Bettaieb A, Wrzal PK, Averill-Bates DA. Hyperthermia: cancer treatment and beyond. In: Rangel L, editor. Cancer treatment: conventional and innovative approaches. Croatia: InTech. 2013:257–283.
- Fu Q, Huang T, Wang X, et al. Association of elevated reactive oxygen species and hyperthermia induced radiosensitivity in cancer stem-like cells. Oncotarget. 2017; 8:101560–101571.
- Abdal Dayem A, Hossain MK, Lee SB, et al. The role of reactive oxygen species (ROS) in the biological activities of metallic nanoparticles. Int J Mol Sci. 2017;18:E120.
- Liu G, Li Q, Ni W, et al. Cytotoxicity of various types of gold-mesoporous silica nanoparticles in human breast cancer cells. Int J Nanomedicine. 2015;10:6075–6087.
- Zhang XD, Wu D, Shen X, et al. Size-dependent radiosensitization of PEG-coated gold nanoparticles for cancer radiation therapy. Biomaterials. 2012;33:6408–6419.
- Su XY, Liu PD, Wu H. Enhancement of radiosensitization by metal-based nanoparticles in cancer radiation therapy. Cancer Biol Med. 2014;11:86–91.
- Lee J, Chatterjee DK, Lee MH, et al. Gold nanoparticles in breast cancer treatment: promise and potential pitfalls. Cancer Lett. 2014;347:46–53.
- Chithrani DB, Jelveh S, Jalali F, et al. Gold nanoparticles as radiation sensitizers in cancer therapy. Radiat Res. 2010;173:719–728.
- Jain S, Hirst DG, Osullivan JM. Gold nanoparticles as novel agents for cancer therapy. Br J Radiol. 2012;85:101–113.
- Mesbahi A. A review on gold nanoparticles radiosensitization effect in radiation therapy of cancer. Rep Pract Oncol Radiother. 2010;5:176–180.
- Gowda S, Krishnaveni S, Yashoda T, et al. Photon mass attenuation coefficients, effective atomic numbers and electron densities of some thermoluminescent dosimetric compounds. Pramana - J Phys. 2004;63:529–541.
- Kurudirek M. Effective atomic numbers and electron densities of some human tissues and dosimetric materials for mean energies of various radiation sources relevant to radiotherapy and medical applications. Radiat Phys Chem. 2014;102:139–146.
- Zhang Y, Ma P, Wang Y, et al. Biocompatibility of porous spherical calcium carbonate microparticles on HeLa cells. World J Nano Sci Eng. 2012;2:25–31.
- Sharifi S, Barar J, Hejazi MS, et al. Doxorubicin changes Bax/Bcl-xL ratio, caspase-8 and 9 in breast cancer cells. Adv Pharm Bull. 2015;5:351–359.
- Wong RS. Apoptosis in cancer: from pathogenesis to treatment. J Exp Clin Cancer Res. 2011;30:87.
- Baharara J, Ramezani T, Divsalar A, et al. Induction of apoptosis by green synthesized gold nanoparticles through activation of caspase-3 and 9 in human cervical cancer cells. Avicenna J Med Biotechnol. 2016;8:75–83.
- Baharara J, Namvar F, Ramezani T, et al. Silver nanoparticles biosynthesized using Achillea biebersteinii flower extract: apoptosis induction in MCF-7 cells via caspase activation and regulation of Bax and Bcl-2 gene expression. Molecules. 2015;20:2693–2706.
- Brentnall M, Rodriguez-Menocal L, De Guevara RL, et al. Caspase-9, caspase-3 and caspase-7 have distinct roles during intrinsic apoptosis. BMC Cell Biol. 2013;14:32.
- Ahamed M, Akhtar MJ, Alhadlaq HA, et al. Copper ferrite nanoparticle-induced cytotoxicity and oxidative stress in human breast cancer MCF-7 cells. Colloids Surf B Biointerfaces. 2016;142:46–54.