Abstract
Keratins derived from human hair have been widely used for tissue engineering. However, some drawbacks relative to the traditional keratins extracts have been found: (a): difficultly controlling the amino acid composition; (b): batch to batch inconsistent quality; and (c): producing complex keratin and keratin-associated proteins (KAPs), which problems have made some studies concerning human hair keratins stagnant, especially in the mechanism studies related to hemostasis of keratins. Herein, a type-I human hair keratin of K37 and a type-II human hair keratin of K81 were heterologously expressed and firstly used for haemostatic application. SDS-PAGE analysis shows that the recombinant keratins had higher purity compared to the extracted keratins. The circular dichroism (CD) spectra of K37 and K81 suggested that the secondary structures were rich in α-helix. In addition, the recombinant keratin proteins could enhance fibrin colt formation at the site of injury and decrease the bleeding time and blood loss in liver puncture and femoral artery injury rat models. This study provides a new strategy for future works involving design and mechanism studies of keratin biomaterials.
Graphical Abstract
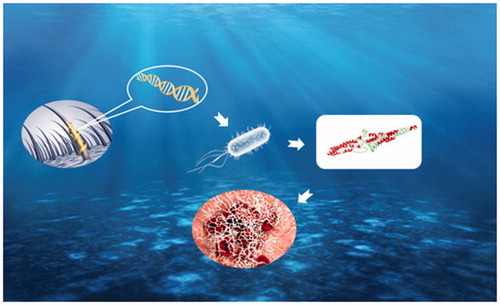
Introduction
Over the past decades, keratins have been extracted from human hair, feather, wool, horns, hooves and nails [Citation1–3], which were fabricated into the films, hydrogels, dressing and scaffolds for biomedical applications in terms of haemostasis, nerve regeneration, wound healing and bone regeneration [Citation4–7]. In particular, the haemostatic application of keratin proteins derived from human hair has been paid special attention [Citation6,Citation8]. The hair fibre consists of 50 − 60% keratins [Citation9], and the human hair keratin nanoparticles have been fabricated and used for haemostasis, the results showed that keratin nanoparticles can significantly reduce the blood loss and coagulation time in the liver puncture and tail amputation in rat models [Citation5].
However, some drawbacks of the human hair keratins extracts have been found: (a): difficultly controlling the amino acid composition and molecular weight (MW); (b): batch to batch inconsistent quality; (c): producing a complex composed of multiple keratin proteins. Human hair keratins are highly cross-linked proteins typically containing α-helix and are rich in cystine, threonine, serine and proline [Citation10]. The common extraction methods, including oxidative reaction, reductive reactions and enzyme hydrolysis, are used to break the cystine bonds of insoluble proteins [Citation6,Citation11,Citation12]. These extraction procedures hardly control the amino acid composition and molecular weight (MW) of extracted keratins due to the random break of cystine bonds [Citation10]. Besides, the properties of extracted proteins are critically dependent on the raw material sources [Citation13]. The quality of human hair keratins is also difficult to control because perming and colouring hair are more and more popular [Citation14]. In addition, cortex mainly contains the keratins and keratin-associated proteins (KAPs) [Citation15], and keratins typically exist as a heterodimeric paring of type I, low MW acidic proteins and type-II high MW, neutral or basic proteins. Genome analyses have demonstrated that human hair keratin comprises 11 type-I hair keratins (K31-K40) and 6 type-II hair keratins (K81-K86) [Citation16,Citation17]. Obviously, the extracted keratins from human hair are the complex of multiple keratins and KAPs.
Herein, we selected a type-I human hair keratin of K37 and a type-II human hair keratin of K81 for heterologous expression, and the haemostatic efficiency and mechanism of recombinant human hair keratin proteins were firstly assessed. Actually recombinant keratins have been previously produced to study the structure features and self-assembly process of intermediate filaments [Citation13,Citation18]. However, this study firstly described the heterologous expression of K37, and this is the first time recombinant keratin proteins were used in tissue engineering (haemostasis). We specifically investigated the physicochemical characterizations of recombinant keratin proteins, and the rat models were applied to assess haemostatic effect of recombinant keratins. Finally, the haemostatic mechanisms of recombinant K37 and K81 were also investigated.
Materials and methods
Cloning of K81 and K37
E. coli TOP10 was used for construction of recombinant plasmid. E. coli BL21 was used as a host strain for expressing recombinant K37 and K81. Gene sequence information of K37 and K81 was obtained from GenBank 8688 and 3877, respectively. The two gene sequences were synthesized, cloned into the vector pET28a and verified by Sangon Biotech (Shanghai, China).
Expression and purification of K81 and K37
E. coli BL21 (DE3) was grown in Luria-Bertani (LB) medium (1 L) with 50 μg/mL ampicillin at 37 °C until an OD600 of 0.8 was reached. Subsequently, isopropyl-β-Dthiogalactoside (IPTG) was added at a final concentration of 0.2 mM to induce enzyme expression at 16 °C for 12 h. The samples were centrifuged at 8000 rpm for 10 min at 4 °C, and cell pellets were resuspended in Buffer A (50 mM Tris pH 8.0, 150 mM NaCl, 5 mM EDTA, 20 mM β-mercaptoethanol) and lysed by constant cell disruption systems (FB-110, Litu mechanical equipment engineering Co., Ltd. China) at 10 KPSI.
The cell extract was centrifuged at 20,000 rpm for 15 min at 4 °C. The precipitates were resuspended in Buffer B (50 mM Tris pH 8.0, 150 mM NaCl, 5 mM EDTA, 20 mM β-mercaptoethanol, 1 M Urea, 0.5% TritonX-100) and sonicated for 3 min. This process was repeated 2 times, and then, the precipitates were washed 2 times with purified water. Finally, the inclusion bodies were dissolved in the Buffer C (2% SDS, pH 11.0, 3% β-mercaptoethanol, 7 M urea) at 50 °C for 15 h. Samples were then centrifuged at 20,000 rpm for 15 min at 4 °C. The supernatant was collected and adjusted to pH 7.4. After centrifugation at 20,000 rpm, the supernatant was dialysed against 10 mM Tris–HCl (pH 7.4) and freeze-dried.
Characterizations
Sds-page
K37 or K81 solution mixed with 4X SDS loading buffer containing 0.6 M β-mercaptoethanol. The mixture was heated at 100 °C for 5 min and used to prepare sample for SDS-PAGE. The denatured solution was loaded onto precast 5–10% gradient Tris–HCl gels. Electrophoresis was performed at 75 V for 1 h, followed by 120 V for 2 h. The gels were then stained by 0.02% (w/v) Coomassie Brilliant Blue G-250 for 2 h and distained with acetic acid twice.
UV spectroscopy
The absorption of the K37 and K81 solutions was measured by using UV–VIS spectrophotometer (Lambda 900, PerkinElmer, USA).
FTIR spectroscopy
The chemical structures of K37 and K81 were analysed by a FT-IR (5DX/550II, Nicolet). The samples were prepared by grinding the dried specimens with KBr and pressing them to form disks.
X-ray diffraction
The XRD experiments were carried out using an X-ray diffractometer (6000X, Shimadzu, Japan). K37 and K81 were analysed in the 2θ ranging from 5 to 45°with a step width of 0.04° and a count time of 2 s.
CD spectroscopy
CD data were collected at 25 °C on a circular dichroism spectrophotometer (CHIRASCAN, Applied Photophysics, UK) by using a 1-mm pathlength quartz cuvette. The spectra were obtained at 1 nm intervals and 1 nm bandwidth from 190 to 300 nm with 1-s signal averaging time. Each outcome was the mean of at least three scans.
DSC analysis
DSC experiments were conducted using a Differential Scanning Calorimeter (DSC 204 F1 Phoenix®, Netzsch, Germany). K37 and K81 (approx. 2 mg) were weighed into 40 μL perforated aluminium pans and heated from 25 to 350 °C at a heating rate of 10 °C/min under a nitrogen atmosphere.
Haemostatic experiments
The well-established rat liver injury and femoral artery injury models were used to test the haemostatic efficacy of K37 and K81 [Citation19]. All procedures were performed under a protocol approved by the Third Military University Animal Care and Use Committee (Chongqing, China). Surgeries were completed on 1–2-month-old Sprague-Dawley rats. Anaesthesia was induced with 10% chloral hydrate by intraperitoneal injection (0.5 ml/100 g). Liver injury model was established by dissection and scratch of about 2 mm in depth with surgical blade to cause bleeding. Furthermore, the femoral artery injury model was established as follows: the left femoral artery was isolated and clamped proximally and distally. Fifty microlitre of 2% lidocaine was applied on the artery to minimize vasoconstriction and reduce variation in the initial free bleeding rate. A 3 mm longitudinal incision was made in the femoral artery segment between the clamps to simulate injury. The experimental groups were treated with K37 and K81 (10 mg) to cover the bleeding location, respectively. The time until cease to haemorrhage was recorded immediately and the mass of blood loss was also measured. The control groups were done with the same procedure but only stopping bleeding with gauze instead of the application of K37 and K81 nanoparticles.
Histological analysis
The femoral artery was excised from femoral artery-injured rats, and Martius scarlet blue (MSB) staining was performed for fibrin in adjacent serial sections.
aPTT and PT assays
Different concentrations of K37, K81 or free heparin were added to 50 μL control normal human plasma (Thermo Scientific) and incubated with 50 μL aPTT reagent (Thermo Scientific) at 37 °C for 3 min. Subsequently, 50 μL 25 mM CaCl2 preincubated at 37 °C was added to the samples, and clotting was monitored at the absorbance of 605 nm with a microplate reader (ELx808, BioTek Instruments, Inc.,). In the PT clotting measurement, normal human plasma (50 μL) was mixed with K37 or K81 and incubated at 37 °C for 3 min, then 50 μL PT reagent pre-incubated at 37 °C for 10 min was added and clotting time was recorded.
Recalcified plasma clotting assay
Recalcified plasma clotting test was performed using a spectrophotometeric method for turbidity measurements. Plasma was recalcificated with 13 mM CaCl2 at 37 °C and added to wells of a 96-well microtitre plate containing 0.5% K37 or K81. PBS and 0.5 U thrombin (Sigma Aldrich, St. Louis, MO) were used as negative and positive controls, respectively. After manual shaking for 5 s, turbidity of the solution was measured by the absorbance at 340 nm every 30 s for 60 min at 37 °C. Lag times were determined using linear regression formulas to calculate the point of intersection between the initial phase of optical density and rapid increase in optical density.
Scanning electron microscopy
Rat blood was collected from retro orbital choroid plexus and mixed K37 or K81 (0.1%, w/v) at 37 °C for 60 min. The clot was removed and fixed with 2.5% gluteraldehyde in 0.1 M sodium cacodylate buffer containing 2 mM CaCl2 for 2 h at room temperature. Then, the clots were washed with sodium cacodylate buffer and counterstained with osmium tetroxide for 1 h. After a final wash with deionized water, samples were put through ethanol dehydration and critical point dried. The samples were mounted and gold sputter coated prior to SEM (Nova 400, FEI).
Statistical analyses
All measurements were performed at least triplicate, and data were presented as mean ± standard deviation (SD). For selected evaluation tests, one-way analysis of variance (ANOVA) was applied by SPSS software. The statistical significance level (p) was set at < .05.
Results and discussion
Molecular weight of the K37 and K81
The haemostatic effects of human hair keratins have been confirmed in the previous studies. However, the haemostatic mechanisms of human hair keratins are not clear because that keratin extracts are rich in keratin proteins and KAPs. Therefore, heterologous expression of human hair keratins is a rational strategy to obtain the pure human hair keratin and then to be used for haemostatic mechanism analysis. The human hair keratin cDNA clones coding for K37 and K81 were generated by PCR and subcloned into the expression vectors pET28a. The expression of these two model proteins were investigated using SDS-PAGE. The molecular weight of K37 and K81 are 49 747 Da and 54 928 Da, respectively. shows the patterns of expression of k37 (approx. 50 kDa) and K81 (approx. 55 kDa). Moreover, SDS-PAGE analysis indicated that the recombinant proteins were more purified than the keratin extracts, since the only bands could be observed in the gel [Citation11,Citation20]. Inclusion body proteins instead of soluble proteins were obtained in this study, which could be explained by the fact that human hair keratins are not water-soluble in nature. However, inclusion body proteins are also useful for haemostatic application, and the solubilization of inclusion body proteins was performed before characterization and haemostatic experiments.
Characterizations of K37 and K81
The aim of recombinant keratins characterization was to investigate their structure and compare the physicochemical features with keratin extracts. Therefore, we have performed the IR, CD, XRD and DSC analysis to study the difference between recombinant keratins and extracts in present study. The UV-Vis absorption spectra of K37 and K81 are shown in . The maximum absorption of K37 and K81 was around 280 nm, which was in agreement with the extracted keratins from human hair [Citation21]. Furthermore, the refolding process was obtained by using CD spectra to estimate the secondary structure of proteins (). The CD spectra of K37 and K81 suggested that the secondary structures were rich in α-helix with a negative band at 220 nm and 215 nm, respectively. This observation was convinced that α-keratin, as a traditional protein α-helix, is the primary component in mammal hairs. depicts the FT-IR spectra of K37 and K81. Similar with the extracted keratins (keratin and keratose), the IR spectra of the recombinant keratins showed some characteristic bands. Primary amide was located at 1650 cm−1, indicating a significant proportion of α-helix structure. The secondary and tertiary amide bonds were observed at 1541 cm−1 and 1260 cm−1. Meanwhile, C-H stretching and O-H stretching vibrations were found at 3292 cm−1, and the -CH2 stretching vibrations were shown at 2926 cm−1. Additionally, the XRD patterns of K37 and K81 are displayed in . Two peaks at 10° and 21° were found in the diffractograms of K37 and K81, suggesting the presence of α-helix structures in the recombinant keratin proteins [Citation22], and the two peaks were similar with that of the extracted keratins [Citation5]. The thermogravimetric curves for K37 and K81 are shown in . The initial weight loss was observed at 50–100 °C due to the loss of moisture, while the second endothermic events occurred in the range of 200–250 °C which was attributed to denaturation of α-helix crystallites in the recombinant proteins [Citation23]. Overall, the recombinant keratin protein shows a similar structure properties with keratin extracts.
Haemostatic effects of K37 and K81
The haemostatic effect of extracted keratins from human hair have been demonstrated in our previous study [Citation5]. To investigate whether the recombinant keratins have this property, the in vivo haemostasis efficacy studies were performed on the liver puncture and femoral artery injury rat models. K37 and K81 (10 mg) were used for femoral artery and liver puncture model to cover the wound areas (). In the liver puncture model, bleeding time was reduced significantly in the presence of recombinant K37 (approx. 38 s) and K81 (approx. 40 s) compared to only vehicle (approx. 170 s, p < .01), and the total blood loss was also significantly less in the presence of K37 and K81 (p < .01). Moreover, the recombinant keratin proteins also obviously decreased bleeding time compared with the control group (approx. 50 s vs. 270 s) in femoral artery injury model. Importantly, K37 and K81 displayed the stronger haemostasis than the extracted keratins (approx. 80 s) for the treatment of rats liver injury [Citation5]. Therefore, the recombinant human hair keratins support a platform to develop the novel haemostatic products based on keratin biomaterials.
Figure 2. Mass of blood loss and time to haemostasis in liver puncture (A, B) and femoral artery injury rat models (C, D). (E) Serial sections of femoral veins were stained for fibrin through MSB staining (fibrin, red; collagen, blue; erythrocytes, yellow). **p < .01, *p < .05.
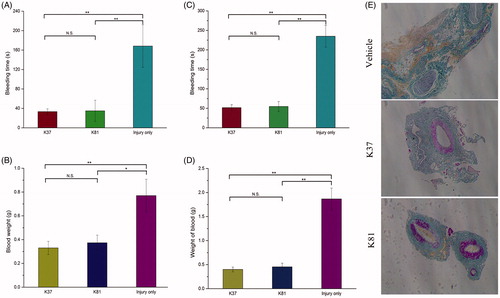
In addition, the haemostatic mechanism of recombinant keratins were also investigated. Fibrin formation on the wound tissue was analysed by Martius Scarlet Blue (MSB) staining (). Fibrin clots were found (in red), and the higher levels of fibrin staining were observed in vessels after treatment with K37 and K81 compared to the control group. These results showed that the recombinant keratins could enhance fibrin colt formation at the site of injury and decrease the bleeding time and blood loss.
There are two main mechanisms for blood coagulation, that is, intrinsic and extrinsic pathways. aPTT and PT are used to measure the performance of the intrinsic and extrinsic coagulation pathway, respectively. aPTT represents the time taken for a fibrin clot to form in the plasma, while PT representing the time taken for a fibrin clot to form after tissue thromboplastin is added [Citation24]. As shown in , the introduction of K37 and K81 decreased aPTT in a concentration-dependent manner, although no significant decrease was observed. The results suggested that the recombinant keratin proteins had strong effect on the intrinsic route of blood coagulation. In addition, K37 and K81 also caused the decrease of PT in the concentration range 0.1–10 g/L, which indicated that K37 and K81 participated in the extrinsic coagulation pathway.
Figure 3. Activated partial thromboplastin time and prothrombin time of normal human control plasma spiked with recombinant human hair K37 (A, B) and K81 (C, D). Scanning electron microscopy of whole blood clots in the presence of recombinant human hair K37 (E) and K81 (F) (0.1%).
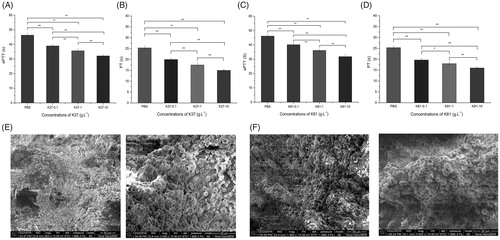
The blood clot formation recombinant K37 and K81 was observed by SEM in the present (). Red blood cells formed aggregates, and fibrin fibres were found in clot. Recalcified plasma clot formation was studied by measuring the change in optical density over time (). The initial lag phase referred to the formation of half-staggered double-stranded fibrin proto fibrils and followed rapid increase in optical density representing the lateral assembly of fibrin fibres. Therefore, K37 and K81 could significantly shorten the lag time compared to the control plasma, and 0.2 U thrombin showed the minimum of lag time. The different slopes indicated that recombinant keratin proteins had the potential to enhance fibrin polymerization in plasma. The results showed that recombinant K37 and K81 participated in both the intrinsic and extrinsic routes of blood coagulation, which can enhance the blood clotting via fibrin clotting. However, more human hair keratins need to be heterologously expressed, which haemostatic properties and mechanisms should also be studied.
Conclusions
In summary, to overcome the limitation of the extracted human hair keratins, we succeeded in heterologously expressing and purifying two recombinant human hair keratins (K37 and K81), and their haemostatic efficiency and mechanism were firstly investigated in the present study. The recombinant keratins had higher purity than the extracted keratins, displaying a typical α-helix structure. Furthermore, the recombinant keratin proteins had strong effect on enhancing fibrin colt formation at the site of injury and halting the bleeding. The data provided in this study not only demonstrated that the recombinant human hair keratins had the similar haemostatic property with the extracted keratins, but also provided a new approach for the research of keratin biomaterials. The recombinant keratin proteins, with a feature of high purity, distinct amino acid sequence and molecular weight and stable properties, have great potential to be used for future studies involving design and mechanism studies of keratin biomaterials in tissue engineering.
Disclosure statement
The authors report that the article content has no conflict of interest.
Additional information
Funding
References
- Cheng Z, Chen X, Zhai D, et al. Development of keratin nanoparticles for controlled gastric mucoadhesion and drug release. J Nanobiotechnology. 2018;16:24.
- Rahmani Del Bakhshayesh A, Annabi N, Khalilov R, et al. Recent advances on biomedical applications of scaffolds in wound healing and dermal tissue engineering. Artif Cells Nanomed Biotechnol. 2018;46:691–705.
- Pilehvar-Soltanahmadi Y, Akbarzadeh A, Moazzez-Lalaklo N, et al. An update on clinical applications of electrospun nanofibers for skin bioengineering. Artif Cells Nanomed Biotechnol. 2016;44:1350–1364.
- Wang S, Wang Z, Foo SEM, et al. Culturing fibroblasts in 3D human hair keratin hydrogels. Acs Appl Mater Interfaces. 2015;7:5187–5198.
- Luo T, Hao S, Chen X, et al. Development and assessment of kerateine nanoparticles for use as a hemostatic agent. Mater Sci Eng C Mater Biol Appl. 2016;63:352–358.
- Burnett LR, Rahmany MB, Richter JR, et al. Hemostatic properties and the role of cell receptor recognition in human hair keratin protein hydrogels. Biomaterials. 2013;34:2632–2640.
- Sierpinski P, Garrett J, Ma J, et al. The use of keratin biomaterials derived from human hair for the promotion of rapid regeneration of peripheral nerves. Biomaterials. 2008;29:118–128.
- Rahmany MB, Hantgan RR, Mark VD. A mechanistic investigation of the effect of keratin-based hemostatic agents on coagulation. Biomaterials. 2013;34:2492–2500.
- Rouse JG, Van Dyke ME. A review of keratin-based biomaterials for biomedical applications. Materials. 2010;3:999–1014.
- Buchanan JH. A cystine-rich protein fraction from oxidized alpha-keratin. Biochem J. 1977;167:489.
- Wang J, Hao S, Luo T, et al. Keratose/poly (vinyl alcohol) blended nanofibers: Fabrication and biocompatibility assessment. Mat Sci Eng C-Mater. 2017;72:212–219.
- Wang J, Hao S, Luo T, et al. Feather keratin hydrogel for wound repair: Preparation, healing effect and biocompatibility evaluation. Colloids Surf B. 2017;149:341–350.
- Parker RN, Roth KL, Kim C, et al. Homo‐and heteropolymer self‐assembly of recombinant trichocytic keratins. Biopolymers. 2017;107:e23037.
- Wang J, Hao S, Luo T, et al. Development of feather keratin nanoparticles and investigation of their hemostatic efficacy. Materials Science and Engineering: C. 2016;68:768–773.
- Lee YJ, Rice RH, Lee YM. Proteome analysis of human hair shaft from protein identification to posttranslational modification. Mol Cell Proteomics. 2006;5:789–800.
- Moll R, Divo M, Langbein L. The human keratins: biology and pathology. Histochem Cell Biol. 2008;129:705.
- Porter RM. The new keratin nomenclature. J Invest Dermatol. 2006;126:2366–2368.
- Coulombe PA, Fuchs E. Elucidating the early stages of keratin filament assembly. J Cell Biol. 1990;111:153–169.
- Zhang M, Zhao Y, Cheng J, et al. Novel carbon dots derived from Schizonepetae Herba Carbonisata and investigation of their haemostatic efficacy. Artif Cells Nanomed Biotechnol. 2017;19:1–10.
- Richter JR, De Guzman RC, Greengauz-Roberts OK, et al. Structure–property relationships of meta-kerateine biomaterials derived from human hair. Acta Biomater. 2012;8:274–281.
- Jain D, Stark AY, Niewiarowski PH, et al. NMR spectroscopy reveals the presence and association of lipids and keratin in adhesive gecko setae. Sci Rep. 2015;5.
- Idris A, Vijayaraghavan R, Rana UA, et al. Dissolution and regeneration of wool keratin in ionic liquids. Green Chemistry. 2014;16:2857–2864.
- Cao J. Melting study of the α-form crystallites in human hair keratin by DSC. Thermochimica Acta. 1999;335:5–9.
- Zhong D, Jiao Y, Zhang Y, et al. Effects of the gene carrier polyethyleneimines on structure and function of blood components. Biomaterials. 2013;34:294–305.