Abstract
Using portion of a nearby nerve trunk to reconstruct a severe nerve lesion by artificial biodegradable chitin conduit is the core practicable method based on peripheral nerve amplification regeneration. However, the quantitative influences on skeletal muscle function corresponding to the injury of the donated nerve fibres were not previously reported. Here, we aimed to explore the compensative capacity in tibialis anterior muscles of rats with the models of acute tibialis anterior nerve branch injuries. The tibialis anterior branch of deep peroneal nerve was transected in various levels each time. Both the decreased treads of maximal compound muscle action potential (CMAP) amplitude and complete tetanic tension of the tibialis anterior muscle in rats were similar with the increasing numbers of damaged nerve fibres, which showed two S-shaped curves. When the nerve injury level was less than approximately 10%, the skeletal muscle function remained normal through complete compensation of motor endplates. As the injury degree went from 10% to 85%, the muscle function was partially impaired due to the broken compensation of motor endplates. When the nerve injury level was over approximately 85%, the skeletal muscle function was totally lost. It suggests that within a certain level of nerve injury, the skeletal muscle function maintained basically unchanged via complete compensation of motor endplates. Such nerve fibres may be used as donor nerve to repair peripheral nerve injury.
Introduction
Peripheral nerve injury (PNI) is very common in clinical orthopaedics. The sensory, motor and nutritional disorders of the corresponding skeletal muscles are caused by the morphological destruction of axons and myelin sheaths [Citation1,Citation2]. The symptoms such as sensory abnormality or absence, myasthenia, amyotrophy, etc. occur subsequently, which remain as a clinical problem and demand urgent solutions. Due to the particularity of peripheral nerve, even if it can be repaired, the corresponding skeletal muscle function is difficult to fully recover, and the motor dysfunction is still a major cause that affects the daily life quality and endangers the physical and mental health of the patients especially in severe peripheral nerve lesion without proper donor nerve.
According to our previous study, portion of a nearby nerve trunk can be used as donor nerve to reconstruct the PNI. Although the injured nerve can get some recovery, the function loss of the father nerve cannot be avoided. Thus, how to improve the reconstruction effects while reducing the function loss on the donor nerve becomes to be a valuable problem. Here, we investigated the quantitative influences on skeletal muscle function corresponding to the injury of the donated nerve fibres. Acute experimental model with injuries of deep peroneal nerve in different levels were made and the compound muscle action potential (CMAP) and complete tetanic contraction were measured to evaluate the functional status of and compensative capacity of skeletal muscles. Results here may give suggestions for the reconstruction of the peripheral nerve and for reducing the risk of donor site function loss.
Materials and methods
Animal preparations
A total of 12 female adult Sprague Dawley rats of SPF level, aged 8 weeks and each weighing 250 ± 10 g, were obtained from the Laboratory Animal Center of Peking University (Beijing, China) and randomly divided into two groups with the electrophysiology group for the measurement of maximal CMAP amplitude and the muscle force group for the measurement of maximal complete tetanic tension. This study was performed in strict accordance with recommendations in the Institutional Animal Care Guidelines and approved ethically by the Administration Committee of Experimental Animals, Peking University People’s Hospital, Beijing, China (Permit Number: 2013-24). All efforts were made to minimize suffering.
CMAP amplitude measurement
The rat in electrophysiology group was deeply anesthetized with 10% chloral hydrate (30 mg/kg, i.p.) for surgical procedures and then put on the operating table in right-lateral position. After preoperative skin preparation and disinfection with 0.5% iodophor in the right lower limb, an L-shaped incision was made at the anterolateral site to expose the tibialis anterior branch of deep peroneal nerve and the tibialis anterior muscle. The active electrode was pierced into the dorsum of proximal tibialis anterior muscle in depth of 2 mm and the reference electrode was located distally in the same depth. The distance between the contact points of the above two electrodes was 15 mm on the muscle surface. The grounding electrode was located in the lateral site of musculi quadriceps femoris. The bipolar needle electrode with 2 mm of distance was chosen for stimulation. All the electrodes were isolated without any contact of each other or unrelated tissues. The stimulating signal produced by MedlecSynergy electrophysiological device (Oxford Instrument, Inc., Abingdon, UK) was set as square waves with 0.09 mA of current, 0.1 ms of duration and 1 Hz of frequency. The tibialis anterior nerve was hooked and gently pulled up without any contact of other tissues (. With a single stimulus, the waveform of CMAP was recorded. After each cut of the tibialis anterior nerve branches with micro-scissors, the same single stimulus was given repeatedly and the corresponding waveforms were recorded as well until it was undetectable. These nerve branches were taken for further process as the measurement was finished. We analysed every CMAP waveform and measured the maximal amplitudes to calculate their descent ratios after each cut.
Tetanic tension measurement
The anaesthesia, dissection and exposure methods were identical to the above ones. The PCLAB-UE biomedical signal acquisition and processing system (MicroStar Science and Technology Development Co. Ltd, Beijing, China) was applied for muscle strength measurement with its supporting PZ-1 tension sensor (50 g of measuring range). The items of “continuous contraction” and “0–50 g” were chosen as the measurement type and range. The image recording parameters were set as 25.00 mV/div and 2.000 s/div. First, a zero setting was performed with 50 g of balancing weight. With the immobilization of tibial plateau, the tibialis anterior tendon was dissociated with knitting sutures and connected parallel to the tension sensor. Then, the MedlecSynergy electrophysiological device was used as stimulation producer and the stimulating signal was set as square waves with 0.09 mA of current, 0.1 ms of duration and 100 Hz of frequency. The tibialis anterior nerve was given continuous stimuli to record the waveforms of complete tetanic contraction (. After each cut of the tibialis anterior nerve branches, the same continuous stimuli were given repeatedly and the corresponding waveforms were recorded until the muscle strength was undetectable. These nerve branches were taken for further process as well. Finally, we analysed every waveform of complete tetanic contraction and measured its maximal tension to calculate their descent ratios after each cut.
Nerve branches processing
All the specimens of nerve branches were fixed with 4% paraformaldehyde solution for 24 h postoperatively and washed under running water for 12 h. After staining with the 1% osmic acid (Ted Pella, Inc., Redding, CA) for 12 h, the specimens were washed again under running water for another 12 h. Ethanol solutions of 50%, 70%, 75%, 80%, 85%, 90% and 95% were prepared for manual dehydration. The embedding cassettes with specimens were immersed for 15 min in each of the above solutions from low to high concentrations, and then successively infused in the 100% ethanol for two times with 15 min per time. After gradient dehydration, the xylene was used for clearing process with the embedding cassettes immersed for two times with 15 min per time. Then the specimens were embedded in paraffin for section and the slices were obtained with a thickness of 2 μm for microscopic observation.
Microscopic observation and nerve fibres counting
The nerve slices were observed under Leica microscope (DM 4000B, Heidelberg, Germany). The nerve tracts were covered with spineurium, and the fat droplets and blood vessels were occasionally seen (). The medullary sheaths were like black rings with axons inside. The cross-section images were obtained and saved with LAS AF image acquisition and analysis system (Leica, Heidelberg, Germany), and the nerve fibres were counted using Image J software (National Institute of Health, Bethesda, MD).
Data analysis
The difference percentages between the total number of nerve fibres and the rest of them were calculated after each cut, and matched respectively with the percentage changes of maximal CMAP amplitudes or maximal complete tetanic tensions to obtain the corresponding discrete data graphs. The Origin software (OriginLab, Inc., Northampton, MA) was used for curve fitting, and therefore, we obtained their variation tendency with decreasing nerve fibres ().
Results
Relationship between PNI levels and maximal CMAP amplitude changes
General changes
The descent ratio of maximal CMAP amplitude in tibialis anterior muscle of rat was in non-linear positive correlation with the increasing ratio of damaged nerve fibres, and its changing trend was presented as an S-shaped curve (.
Figure 5. Diagram of CMAP waveform variations. (A) Maximal CMAP amplitude without nerve damage. (B) When 12.52% of the nerve fibres was damaged, the maximal CMAP amplitude declined by 14.55%. (C) When 25.74% of the nerve fibres was damaged, the maximal CMAP amplitude declined by 30.99%. (D) When 43.26% of the nerve fibres was damaged, the maximal CMAP amplitude declined by 63.14%. (E) When 72.61% of the nerve fibres was damaged, the maximal CMAP amplitude declined by 76.06%. (F) When 91.08% of the nerve fibres was damaged, the maximal CMAP amplitude declined by 92.49%.
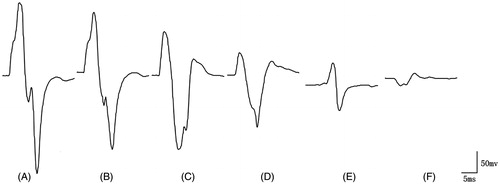
Segmental changes
When the damage level of nerve fibres was approximately below 10%, the curve changed gently in a macroscopic perspective. When the damage level of nerve fibres was 10–45%, the curve went up rapidly, and the ratio of the decreased percentage of maximal CMAP amplitude to the decreased percentage of nerve fibres was larger than 1. When the injury level was 45–80%, the increasing trend of the curve slowed down. Once reached 80% or above, the slope of the curve became steeper again, and the maximal amplitude became 0 as the injury level was up to 100% (.
Relationship between PNI levels and maximal complete tetanic contraction
General changes
The decent ratio of maximal complete tetanic tension in tibialis anterior muscle of rat was in non-linear positive correlation with the declining ratio of damaged nerve fibres, and its changing trend was presented as an S-shaped curve (.
Segmental changes
When the damage level of nerve fibres was approximately below 10%, the maximal complete tetanic tension had no obvious decline (). When the damage level was between 10% and 25%, the slope of the curve was steep, and the ratio of the decreased percentage of maximal complete tetanic tension to the decreased percentage of nerve fibres was larger than 1. The curve changed gently as the injury level was 25–55%, and the decline of maximal complete tetanic tension reached a platform. Once the injury level was more than 55%, the slope of the curve became steeper again, and also, the ratio rose to 1. As the injury level reached approximately 80% or above, the maximal complete tetanic tension was close to 0 (.
Figure 6. Variation trend of maximal complete tetanic tension. Each of the SD rat provides 3–4 fitting points.
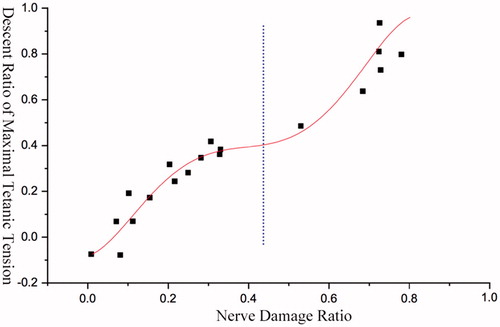
Figure 7. Diagram of complete tetanic contraction waveform variations. (A) Maximal complete tetanic tension without nerve damage. (B) When 7.25% of the nerve fibres was damaged, the maximal complete tetanic tension declined by 6.60% of maximal complete tetanic tension decline with nerve injury. (C) When 25.11% of the nerve fibres was damaged, the maximal complete tetanic tension declined by 27.92%. (D) When 48.02% of the nerve fibres was damaged, the maximal complete tetanic tension declined by 54.25%. (E) When 68.56% of the nerve fibres was damaged, the maximal complete tetanic tension declined by 63.51%. (F) When 72.94% of the nerve fibres was damaged, the maximal complete tetanic tension declined by 92.47%.
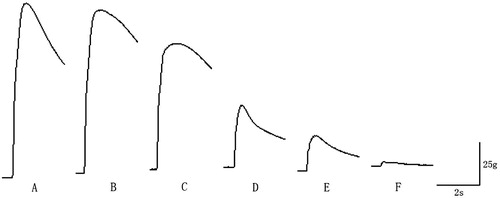
Discussion
In this study, we investigated the quantitative influences on skeletal muscle function corresponding to the injury of the donated nerve fibres. We found that after acute PNI, the compensative capacity of skeletal muscle was in non-linear positive correlation with the damage level of nerve fibres. The skeletal muscle function remained unchanged via complete compensation of motor endplates within certain range of nerve injury; once beyond this range, the skeletal muscle function was impaired or even lost due to partial or complete decompensation.
Motor endplate is widely distributed in skeletal muscle tissues, and it was firstly described by Ramon y Cajal [Citation3]. Being composed of nerve terminals and muscle fibres, the motor endplate is a special type of chemical synaptic structure that transforms electrical signals of nerve fibres to mechanical movements of muscle fibres [Citation4]. The normal structure and function of motor endplates depend on the integrity of nerve fibres and determine the function of skeletal muscles. More importantly, they are considered as the key factors affecting the post-traumatic recovery of neurological function [Citation5,Citation6]. The skeletal muscle function can be evaluated by CMAPs and tetanic contractions. However, quantitative studies about the effects of PNI on skeletal muscle function were seldomly reported before. The experimental anatomy shows that musculi tibialis anterior is a single head muscle innervated by the tibialis anterior branch of deep peroneal nerve, which excluded the possibility of the interference from multicipital muscles or multiple innervations. Using this model, we explored the compensative capacity variations of skeletal muscle function.
The CMAP of skeletal muscle is integrated by multiple single action potentials of nerve fibres under stimulation [Citation7]. As a typical and mature method in the diagnosis of PNI [Citation8], the maximal CMAP amplitudes quantify the complete structure of “axon – motor endplate – muscle fibre” and reflects the overall function of the motor units [Citation9,Citation10]. If the peripheral nerve is injured, the structural integrity will be lost due to axonotmesis with the decline of maximal CMAP amplitude [Citation11,Citation12]. Another method used in the assessment of PNI is to measure the contractility of the skeletal muscle. According to the stimulus frequency, skeletal muscle contractions include single contraction and tetanic contraction. Overall, the complex movements of limbs, the maintenance of postures and the preservation of body temperature mainly depend on the tetanus contraction. The maximal tension produced by complete tetanic contraction is much larger than that of single contraction tension, and it reflects the number of functional motor endplates as well [Citation13].
We also found that the declining trends of maximal CMAP amplitude and maximal complete tetanic tension were accordant and both of them were presented as similar S-shaped curves. Based on the calculation, the ratio of nerve fibres between the two major branches of tibialis anterior nerve was approximately 1:0.82 to 1:1.22. At the early stage of nerve injury, due to the few impacted motor units and the complete compensatory of motor endplates, the changing trends of the curves were relatively smooth, and both of the peakslopes were less than 1. With the increasing level of nerve injury, both the maximal CMAP amplitude and the maximal complete tetanic tension decreased to the platform of intermediate stage, and the motor endplates were in the partial compensatory stage. This was possibly because one of the major nerve branch was almost impaired while the other was still at the very early stage of damage. Thus, with the platform as a boundary, the curves were divided into two parts and there were certain similarities between each of them in shapes and changing trends. The latter half of the curves was more likely to reflect the trend of skeletal muscle function after the injury of another smaller nerve branch. Once the PNI level was beyond approximately 85%, the maximal CMAP amplitude decreased significantly and the maximal complete tetanic tension was seldomly measured. This was mainly because that the function of motor endplates was close to total decompensation and the contraction of few muscle fibres was unable to produce the cocontraction of the whole skeletal muscle.
The treatment of peripheral nerve defect is still a great challenge for orthopaedic surgeons. It is a complicated process of nerve injury repair due to the special anatomy and function. Gu [Citation14] believes that when the length of nerve defect is over four times of its diameter, it should be repaired with nerve graft because of the excessive tension after anastomosis. More importantly, tension free autologous nerve transplantation is still considered the most significant way of repair in the present [Citation9,Citation11,Citation12]. The compensative capability variations of motor endplates in tibialis anterior muscle of rat were reflected objectively by the methods mentioned above. When the acute nerve injury level was approximately 10%, the maximal CMAP amplitude decreased less than 10% and the maximal complete tetanic tension had no remarkable change. Thus, the function of tibialis anterior muscle maintained stable through total compensation of the remaining motor endplates. This means that the function of the tibialis anterior muscle will not be significantly affected even if 10% of the nerve fibres are used as a nerve graft donor. Plomp et al. [Citation15] believe that 10% of the CMAP decrements is generally considered as evidence of motor endplates defect, which is in accordance with our results. Although not yet used in clinical practice, these results provide new theoretical guidance for the selection of donors in the treatment of peripheral nerve defects by autologous nerve transplantation [Citation16–20].
As the amplitude size and shape of CMAP depend on the location of recording electrodes [Citation21–24], the nerve fibre types [Citation18] and the features and number of motor endplate in skeletal muscle [Citation5,Citation25], the measurement of CMAP is not the most direct method in the evaluation of muscle function. However, muscle strength, which is not affected by the electrode location and other variables, is more intuitive and reliable. Hence, this research also provides a new idea to confirm the nerve transection ratio of selective peripheral neurotomy for the treatment of simple limb spasticity [Citation18,Citation16].
To sum up, within a certain range of acute PNI, the skeletal muscle function maintains a relatively stable trend through motor endplates compensation. Such nerve fibres may be used as donor nerve to repair PNI. As our study is an acute animal experiment, it is necessary to explore the long-term change law of skeletal muscle compensative capacity after PNIs of different levels.
Disclosure statement
No potential conflict of interest was reported by the authors.
Additional information
Funding
References
- Millesi H. Reappraisal of nerve repair. Surg Clin N Am. 1981;61:321–340.
- Lundborg G. A 25-year perspective of peripheral nerve surgery: evolving neuroscientific concepts and clinical significance. J Hand Surg. 2000;25:391–414.
- Ramon y Cajal S. Degeneration and regeneration in the nervous system. London: Oxford University Press; 1928.
- Miledi R, Slater CR. On the degeneration of rat neuromuscular junctions after nerve section. J Physiol (Lond). 1970;207:507.
- Li Q, Zhang P, Yin X, et al. Early nerve protection with anterior interosseous nerve in modified end-to-side neurorrhaphy repairs high ulnar nerve injury: a hypothesis of a novel surgical technique. Artif Cells Nanomed Biotechnol. 2015;43:103.
- Zhang P, Yin X, Kou Y, et al. The electrophysiology analysis of biological conduit sleeve bridging rhesus monkey median nerve injury with small gap. Artif Cells Nanomed Biotechnol. 2009;37:101.
- Caliandro P, Stålberg E, La TG, et al. Sensitivity of conventional motor nerve conduction examination in detecting patchy demyelination: a simulated model. Clin Neurophysiol. 2007;118:1577–1585.
- Rutz S, Dietz V, Curt A. Diagnostic and prognostic value of compound motor action potential of lower limbs in acute paraplegic patients. Spinal Cord. 2000;38:203–210.
- Sleutjes BTHM, Montfoort I, Maathuis EM, et al. CMAP scan discontinuities: automated detection and relation to motor unit loss. Clin Neurophysiol. 2014;125:388–395.
- Yin F, Kou YH, Wang YH, et al. Morphological study on the collaterals developed by one axon during peripheral nerve regeneration. Artif Cells Nanomed Biotechnol. 2014;42:217.
- Friedman WA. The electrophysiology of peripheral nerve injuries. Neurosurg Clin N Am. 1991;2:43–56.
- Kane NM, Oware A. Nerve conduction and electromyography studies. J Neurol. 2012;259:1502–1508.
- Celichowski J, Raikova R, Aladjov H, et al. Dynamic changes of twitchlike responses to successive stimuli studied by decomposition of motor unit tetanic contractions in rat medial gastrocnemius. J Neurophysiol. 2014;112:3116–3124.
- Gu Y. The present status and progress in the treatment of peripheral nerve defects. Chin J Trauma. 2002;18:517–519.
- Plomp JJ, Morsch M, Phillips WD, et al. Electrophysiological analysis of neuromuscular synaptic function in myasthenia gravis patients and animal models. Exp Neurol. 2015;270:41–54.
- Han F, Guo J, Yu, et al. Highly selective peripheral neurotomy of muscular branch for treatment of spastic cerebral palsy. Chin J Pediatr Surg. 2003;24:38–40.
- Li J, Liu H, Du L, et al. Comparison of therapeutic effects of selective neurotomy of tibial nerve muscle branches under microscope for spastic foot in adults and children with cerebral palsy. Chin J Surg. 2015;663–666.
- Moradzadeh A, Borschel GH, Luciano JP, et al. The impact of motor and sensory nerve architecture on nerve regeneration. Exp Neurol. 2008;212:370–376.
- Yin XF, Kou YH, Wang YH, et al. Portion of a nerve trunk can be used as a donor nerve to reconstruct the injured nerve and donor site simultaneously. Artif Cells Nanomed Biotechnol. 2011;39:304–309.
- Yin XF, Kou YH, Wang YH, et al. Can "dor to dor + rec neurorrhaphy" by biodegradable chitin conduit be a new method for peripheral nerve injury? Artif Cells Nanomed Biotechnol. 2011;39:110–115.
- Kincaid JC, Brashear A, Markand ON. The influence of the reference electrode on CMAP configuration. Muscle Nerve. 1993;16:392–396.
- Brashear A, Kincaid JC. The influence of the reference electrode on CMAP configuration: leg nerve observations and an alternative reference site. Muscle Nerve. 1996;19:63–67.
- Kurokawa K, Mimori Y, Tanaka E, et al. Age-related change in peripheral nerve conduction: compound muscle action potential duration and dispersion. Gerontology. 1999;45:168.
- Phongsamart G, Wertsch JJ, Ferdjallah M, et al. Effect of reference electrode position on the compound muscle action potential (CMAP) onset latency. Muscle Nerve. 2002;25:816–821.
- Jiang BG, Feng YX, Xun ZP, et al. Hypothesis of peripheral nerve regeneration induced by terminal effectors. Artif Cells Nanomed Biotechnol. 2013;42:92–94.