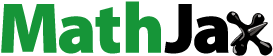
Abstract
Nanocapsules containing selol and doxorubicin (NCS-DOX) with an oily core of selol and a shell of poly(methyl vinyl ether-co-maleic anhydride) covalently conjugated to doxorubicin were developed in a previous work. In this study, these nanocapsules showed a similar antitumour effect in comparison to the free doxorubicin (DOX) treatment, but showed no evident DOX-related cardiotoxicity, as evidenced by serum creatine kinase-MB (CK-MB) activity. The histopathological analysis showed that the free DOX treatment induced more intense morphological damage to myocardial tissues in comparison to NCS-DOX treatment. Animals treated with free DOX presented important muscle fibre degradation and animals treated with NCS-DOX, heart tissue did not present signals of muscle fibre degeneration. These results indicate that the cardiotoxicity related to DOX is reduced when this drug is carried by the NCS-DOX. Noteworthy, biodistribution analyses showed that NCS-DOX accumulated more intensely in tumours than the free DOX. Thus, this study reinforces the importance of the development of nanocapsules as drug carriers for the treatment of cancer.
Introduction
In recent decades, nanocapsules have been investigated for treatment and diagnosis of different types of diseases. In particular, application of nanotechnology for cancer therapy has been widely addressed [Citation1,Citation2]. The use of nanostructures in cancer therapy has been classically supported by their accumulation in tumour tissues as a consequence of the enhanced permeability and retention (EPR) effect [Citation3]. The EPR effect was described in the 1980s, and occurs in certain solid tumours due to the leaky vasculature and reduced lymphatic vascularization often found in this kind of tissue. This morphophysiological pattern creates a kind of dead-end street, where nanocapsules can permeate tumour tissues, but cannot be cleared out by lymphatic vessels. Thus, different nanostructures have been described which improve the biodistribution of chemotherapeutic drugs [Citation4].
Besides the passive delivery of drugs to tumour tissues, nanostructures can also be used for simultaneous delivery of different chemotherapeutic agents to cancer cells [Citation5,Citation6]. This co-delivery, which is explored in this study, takes advantage of possible synergic or additive effects of two selected chemotherapeutical agents, selol and doxorubicin (DOX). Selol is an oily mixture of different selenitetriacylglycerides, which has been shown to be cytostatic and to sensitize cancer cells to the effects of chemotherapeutics such as vincristine and DOX [Citation7]. DOX is a widely used chemotherapeutic drug with cytotoxic effect against a plethora of cancer cell types [Citation8]. Our research group has investigated the use of different types of nanocarriers, including some nanocapsules. Muehlmann et al. [Citation9] developed a nanoparticle consisting of a copolymer (PVM/MA) sphere used to immobilize a photosensitizer for breast cancer photodynamic therapy [Citation10]. De Souza et al. [Citation11] developed nanocapsules with a core of selol, which is an oily mixture of selenitetriacylglycerides, and a shell of PVM/MA [Citation11,Citation12]. Next, Ganassin et al. [Citation5] combined DOX and selol in a similar PVM/MA-shelled nanocapsule (NCS-DOX), which was shown to co-deliver the loaded agents into mammary adenocarcinoma 4T1 cancer cells, as observed in in vitro experiments.
This study reports on the in vivo efficacy and safety of NCS-DOX. In short, NCS-DOX were efficiently delivered to tumour tissue, exhibiting an anticancer efficacy comparable to that of the free DOX treatment. It is noteworthy that the cardiac toxicity of NCS-DOX was significantly lower in comparison to that of free DOX.
Materials
Selol, 5% in selenium (w:w), was provided by the Medical University of Warsaw, Poland; PVM/MA (Gantrez AN 119, ISP Corporation, Brazil) was kindly donated by the ISP Corporation (São Paulo, Brazil). The mouse mammary carcinoma cell line (4T1) was purchased from American Type Culture Collection, Manassas, VA, and the mouse fibroblasts (NIH-3T3) were purchased from the Cell Bank of Rio de Janeiro, Rio de Janeiro, Brazil. Glucose was purchased from J. T. Backer (Brazil). DOX (European Pharmacopoeia standards) was purchased from Sigma, St. Louis, MO. Ketamine and xylazine were both purchased from Syntec, Cotia, Brazil. Eosin and hematoxylin were both purchased from Lafan Química Fina, Várzea Paulista, Brazil. Formaldehyde 37% (w:v) was purchased from Cromoline (Sao Paulo, Brazil). Phosphate buffered saline (PBS) was purchased from Laborclin (Parana, Brazil). Glacial acetic acid and glycerin were both purchased from Vetec (Brazil). Paraffin was purchased from Dinamica, Santa Cruz, Brazil.
Preparation of nanocapsules
Nanocapsules with an oily core of selol and a shell of PVM/MA conjugated to DOX, henceforward referred to as NCS-DOX, were prepared by a nanoprecipitation method recently described [Citation5]. The NCS-DOX present an average hydrodynamic diameter of 170 nm and a spherical shape, according to data previously published [Citation5]. Nanocapsules containing only selol (NCS), used as a control, were also prepared by using the same protocol.
Animals and tumour induction
Procedures with animals were performed according to international practices for animal use and care, and were approved by the Animal Care and Ethics Committee of the University of Brasilia (protocol number 139625/2015). Eight-week-old female BALB/c mice were obtained from IPEN-CNEN, São Paulo, Brazil. Mice were housed in a controlled environment, with circadian cycle of 12 h/12 h dark/light, at 24 °C, with purina chow and filtered water ad libitum.
For tumour induction, a previously described protocol was used [Citation10,Citation13]. Briefly, animals were first anesthetized with 100 µL solution of ketamine hydrochloride and xylazine (0.8 and 0.1 mg/kg, respectively), injected intraperitoneally. Then, 100 µL of a suspension of 4T1 cells in RPMI medium without FBS, at a concentration of 2 × 105 cells/mL, was injected subcutaneously in the lower right flank of each animal – except for non-tumour-bearing animals (control), which received only culture medium.
Clinical analyses
Fifteen days after the 4T1 cells were inoculated into the mice, treatments were intravenously (IV) administered through the tail vein. The administered treatments were: 1) NCS-DOX, 2) NCS, 3) DOX and 4) vehicle only (administered to both tumour- and non-tumour-bearing controls). The vehicle of all formulations consisted of an aqueous solution of glucose (5%, w:v). Each group comprised six mice, which received four doses (3.2 mg DOX/kg/dose) of treatments every 3 d. Mice were then observed for 21 d after the treatment for their body weight and tumour size.
The volume of tumours was calculated according to the following equation:
where TV is the tumour volume in mm3, L is the tumour length in mm and W is the tumour width (mm). Notice in the equation above that (L+ W) is the estimated tumour diameter.
Quantification of the serum creatine kinase MB activity
Blood was collected in tubes containing separating gel for serum separation. The activity of creatine kinase-MB (CK-MB) in serum samples was analysed with an automatic analyser (Chem Well T, LabTest, Brazil).
Cardiac tissue histology
For morphological analysis, heart samples were fixed with paraformaldehyde (4%, w:v) for 4 h, and prepared for conventional histological preparation [Citation10]. Histological slides were sectioned (5 µm) and stained with classical hematoxylin and eosin (H&E).
Biodistribution study
Twenty-one days after the 4T1 cells were inoculated, mice received a single dose of NCS-DOX or DOX, equivalent to 2.2 mg DOX/kg, or the vehicle only (aqueous solution of glucose 5% w:v). At 2, 4 or 8 h after administration, heart, lung, spleen, liver, kidneys and tumours were collected. The DOX-specific fluorescence in organs/tumour was analysed ex vivo with the imaging system IVIS® Lumina XR (PerkinElmer, Waltham, MA). The excitation and emission wavelengths for detection of DOX were 480 and 570 nm, respectively. The biodistribution data were expressed as photons/second/gram of tissue.
Statistical analysis
Data were analysed with GraphPad Prism® version 6.0 software (La Jolla, CA). Significant differences between data sets were assessed by one- or two-way ANOVA, with Tukey’s or Sidak’s post-tests (α = 0.05). Quantitative results were expressed as mean ± standard error of the mean.
Results
Biodistribution
shows a qualitative representation of DOX fluorescence in different organs, and different time points and treatments. It is possible to observe that the liver and tumours presented the highest DOX fluorescence signals. presents the quantitative evaluation of DOX fluorescence signal whereas represents the area under the curve of the data plotted in . show that the NCS-DOX treatment significantly increase (p < .05) the DOX delivery to the tumour mass in comparison to the free DOX treatment. Although there were no significant differences at 2 and 4 h post-administration, at 8 h mice treated with NCS-DOX show a significantly higher fluorescence signal in comparison to 2 and 4 h while the mice treated with free DOX present no signal, i.e. under the detection limit.
Figure 1. Ex vivo fluorescence of Doxorubicin in organs collected from mice treated with free doxorubicin (DOX) or with nanocapsules containing selol and doxorubicin (NCS-DOX) at 2, 4 and 8 h post-administration. Each panel above shows the organs of one representative animal out of three studied for each time and treatment.
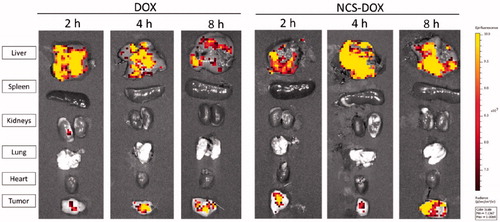
Figure 2. Graphs presents the quantitative evaluation the DOX-specific fluorescence photon counts of DOX (free doxorubicin) and nanocapsules containing selol and doxorubicin (NCS-DOX), as photons per second per gram of tissue (p/s/g). Data are expressed as mean ± standard error of the mean for three animals.
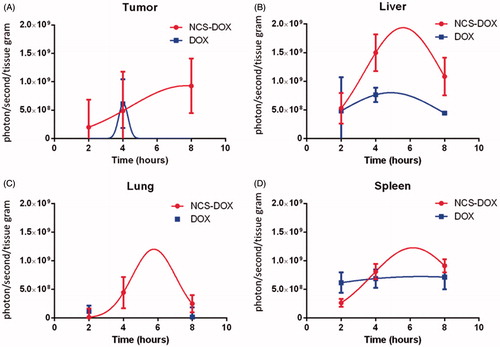
Figure 3. Graphs represents the area under the curve of the data plotted in . Data are expressed as mean ± standard error of the mean for three animals.
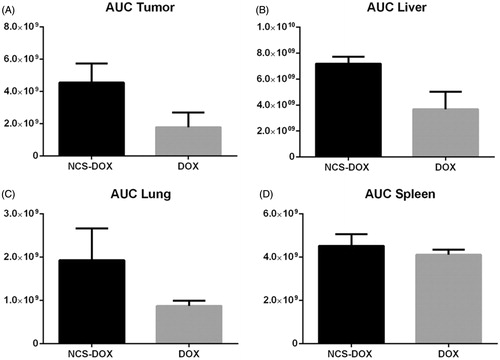
DOX accumulation in liver, lungs and spleen was not statistically different (p > .05), at any tested time, while comparing with NCS-DOX and free DOX treatments. For the kidneys, DOX was quantified just for the free DOX treatment at 2 h post-treatment. For all the other treatments and endpoints, quantification was under the detection limit. In heart, the DOX signal was not detected for either NCS-DOX or free DOX treatments.
Efficacy study
All experimental treatments evaluated in this study (NCS-DOX, NCS, and free DOX) impaired the 4T1 tumour growth with comparable efficacy (). Tumour growth curve shows that the three experimental treatments reduced tumour growth in comparison to untreated tumour-bearing mice. On the last day of tumour measurements, tumours from the treated mice (NCS-DOX, DOX and NCS) were about seven times smaller on average than tumours in control group (). This indicates that NCS-DOX, DOX and NCS show similar in vivo antitumour potential in the present experimental model.
Figure 4. Graphs represents evolution of 4T1 tumours volume in mice treated with free doxorubicin (DOX), nanocapsules containing selol and doxorubicin (NCS-DOX), nanocapsules of selol (NCS) and control group (vehicle only, which consisted of an aqueous solution of glucose 5% w:v). Treatments were performed at days 0, 3, 6 and 9 (arrows). Data are expressed as mean ± standard error of the mean. n = 6 mice per group. a=p < .05 versus all other treatments.
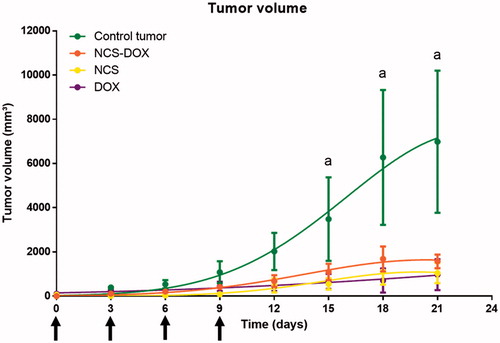
Toxicity evaluation
Mice overall body weight
Mice treated with either NCS or NCS-DOX did not present significant weight alteration during the experiments. However, in comparison to the mice without tumours, free DOX treatment significantly reduced (p < .05) the overall body weight after two weeks of observation ().
Figure 5. Graphs represent weight alteration in mice treated with free doxorubicin (DOX), nanocapsules containing selol and doxorubicin (NCS-DOX), nanocapsules of selol (NCS), control group with and without tumour (vehicle only, which consisted of an aqueous solution of glucose 5% w:v).during the experiments. Data are expressed as mean ± standard error of the mean. n = 6 mice per group. a = p < .05 versus all other treatments.
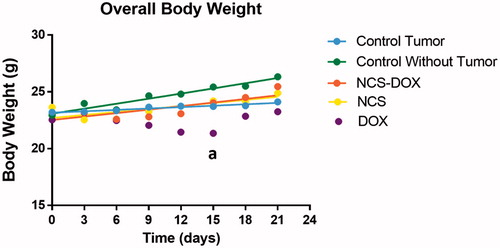
Cardiotoxicity
Free DOX treatment induced a statistically significant (p < .05) cardiotoxicity to experimental mice, as evidenced by the higher serum CK-MB levels in comparison to control groups (). The serum CK-MB activity of mice treated with NCS-DOX was not significantly different from that observed in non-treated tumour-bearing mice.
Figure 6. Graphs represent creatine kinase MB (CK-MB) activity in the serum of mice treated with doxorubicin alone (DOX), nanocapsules containing selol and doxorubicin (NCS-DOX), nanocapsules of selol (NCS), and control mice, with and without tumour (vehicle only, which consisted of an aqueous solution of glucose 5% w:v). Data are expressed as mean ± standard error of the mean. a = p < .05 when compared to the other groups, except NCS.
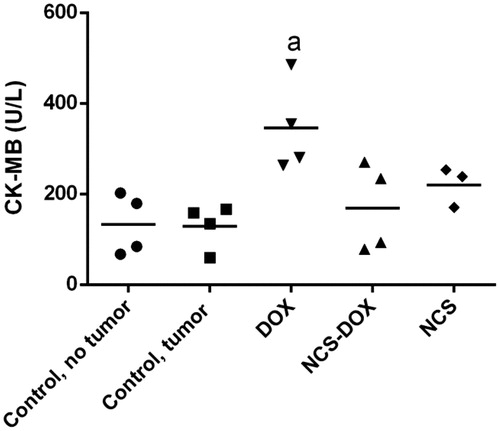
Besides the CK-MB levels in serum, the heart histology was also analysed to observe possible morphological alterations in cardiac tissue. It is possible to observe the normal aspect of healthy heart histology in , from control and NCS-treated mice, respectively, presenting straight, parallel muscle fibres and perpendicular intercalated discs. In addition, healthy myocardial cells have euchromatic nucleus with an evident nucleolus. On the other hand, signs of tissue degeneration are clearly observed in different degrees in mice treated with DOX () and NCS-DOX (). Animals treated with free DOX present important muscle fibre degradation, represented by the wavy shape of muscle fibres (big arrow). Moreover, the presence of heterochromatic nucleus is an anomalous condition. For animals treated with NCS-DOX, heart tissue did not present muscle fibre degeneration, but some amount of inflammatory infiltration is observed, which is an initial indicator of tissue degeneration. These results indicate that the cardiotoxicity related to DOX is reduced when this molecule is associated with the NCS-DOX.
Figure 7. Optical microscopy images of heart tissue of: (A) control tumour-bearing mice (received vehicle only, which consisted of an aqueous solution of glucose 5% w:v), (B) mice treated with nanocapsules of selol (NCS), (C) mice treated with doxorubicin alone (DOX), and (D) mice treated with nanocapsules containing selol and doxorubicin (NCS-DOX). Arrows in A indicate parallel muscle fibers and the perpendicular intercalated discs. The arrow in C indicates wavy muscle fibers. The circle in D shows inflammatory infiltrate. Classical staining with hematoxylin and eosin was used.
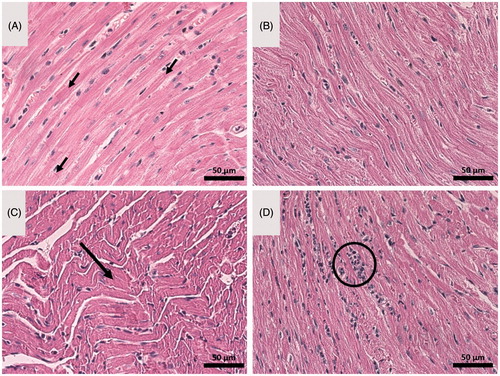
Discussion
Our findings herein reported show that the NCS-DOX formulation had a similar antitumour effect in comparison to the free DOX treatment, though significantly reducing DOX-related cardiotoxicity. These results are similar to those described for Doxil™, the first FDA-approved liposomal nanotech product, which has been extensively investigated in clinical trials in recent years [Citation14]. These are very important results, since the cardiotoxicity is a dose-limiting issue in DOX treatments [Citation14,Citation15].
Despite these important clinical results reported for Doxil™, some concerns related to production and manufacturing contamination of these classical liposomal formulations have been published in the literature [Citation16]. As a multistep process that involves liposome size selection based on filtration, such a protocol could increase the risks of contamination or reduce the reproducibility of different batches. Ideally, nanoparticles should be produced by one-step self-assembly protocols to avoid the aforementioned drawback. The classical nanoprecipitation method for polymeric nanocapsules preparation was used in this study, and it represents an effective alternative manufacturing process for new nanocarriers. As a main advantage, the nanocapsules are formed in a single-step process, a fact that may avoid product contamination, and increase production reproducibility.
The biodistribution study showed that NCS-DOX was able to increase DOX delivery to tumours in comparison to the free DOX treatment. This important result can be explained by the classical EPR effect, which allows for certain nanocapsules to passively permeate leaky tumour vessels; once the nanocapsules reach the tumour interstitium, they are retained for long periods due to poor lymph drainage [Citation17]. This could lead to longer exposure of the cancer cells to nanostructures, increasing the effectiveness of the treatment.
As a small hydrophilic compound, the free DOX is readily cleared by the renal circulation [Citation18], which explains the short half-life of this drug, which is usually less than 1 h. However, when the molecule is associated with a nanocarrier, such as NCS-DOX, renal clearance is reduced due to the size of the nanocarriers, and the DOX half-life is increased as a consequence. This evidence was also observed in our in vivo results, where the DOX signal was not observed in the kidneys in the NCS-DOX treatments, but it was detected in the free DOX groups (data not presented).
For liver, spleen and lungs, no statistically significant difference was observed between the experimental groups, but in all these organs NCS-DOX had a higher DOX signal compared to the free DOX treatment. As they are highly vascularized organs, this event is commonly reported in the literature [Citation13]. For heart analysis, no significant DOX signal was detected. This result can be related to the limit of detection of the techniques used in our experiment; however, in the histopathological analysis is clear that the free DOX treatment induced more intense morphological damage to myocardial tissues in comparison to NCS-DOX treatment. This is an important evidence that DOX was not delivered in significant amounts to heart tissue in mice treated with NCS-DOX.
In addition to the reduction of cardiotoxicity, evidenced by the CK-MB levels and the histological evaluation, absence of weight loss was observed in mice treated with NCS-DOX, but this adverse effect was also observed in mice treated with free DOX after the second week of observation. These are very important results, since free DOX treatment induced both weight loss and cardiotoxicity, which are the most important side-effects described for this chemotherapeutic drug. Indeed, at the moment, the benefits of nanocarrier use are more evident for the prevention or reduction of the toxic effects of the traditional chemotherapeutic drugs.
The main objective of this study was to investigate the antitumour improvement of a nanocapsule containing both selol and DOX as chemotherapeutic agents. The antitumour effect of a similar nanocapsule containing selol was previously observed by our research group [Citation12]. In addition, the intracellular co-delivery of these molecules and the enhanced in vitro cytotoxicity achieved with selol and DOX combined were also demonstrated by our research group in 4T1 cancer cells [Citation5].
As evidenced by our results, NCS-DOX presented similar antitumour activity in comparison to both free DOX and the NCS treatment. In contrast to our previous in vitro experiments [Citation5], enhanced efficacy due to the co-delivery of anticancer agents was not achieved in our in vivo cancer model. However, because superior safety was observed with the treatment with NCS-DOX compared to free DOX, this nanostructures may be used in the future to reduce the toxicity of DOX and, possibly, of other chemotherapeutic drugs.
Conclusions
This study demonstrates that intratumoural accumulation of DOX delivered in vivo by NCS-DOX was prolonged in comparison to free DOX, a result that may be associated with the EPR effect. Although the antitumour efficacy of NCS-DOX was not increased in comparison to selol or free DOX alone, it presented significantly reduced cardiotoxicity in comparison to free DOX. Thus, NCS-DOX has to be further studied regarding its potential use in humans to reduce the toxicity of DOX-based anticancer treatments.
Disclosure statement
No potential conflict of interest was reported by the authors.
Additional information
Funding
References
- Torchilin V. Tumor delivery of macromolecular drugs based on the EPR effect. Adv Drug Deliv Rev. 2011;63:131–135.
- Nichols JW, Bae YH. Odyssey of a cancer nanoparticle: from injection site to site of action. Nano Today. 2012;7:606–618.
- Wilhelm S, Tavares AJ, Dai Q, et al. Analysis of nanoparticle delivery to tumours. Nat Rev Mater. 2016;1:16014.
- Xu Y, Meng H. Paclitaxel-loaded stealth liposomes: development, characterization, pharmacokinetics, and biodistribution. Artif Cell Nanomed Biotechnol. 2016;44:350–355.
- Ganassin R, Merker C, Rodrigues MC, et al. Nanocapsules for the co-delivery of selol and doxorubicin to breast adenocarcinoma 4T1 cells in vitro. Artif Cell Nanomed Biotechnol. 2017;1–11. DOI: 10.1080/21691401.2017.1408020
- Afsharzadeh M, Hashemi M, Mokhtarzadeh A, et al. Recent advances in co-delivery systems based on polymeric nanoparticle for cancer treatment. Artif Cell Nanomed Biotechnol. 2017;1–16. DOI: 10.1080/21691401.2017.1376675
- Suchocki P, Misiewicz I, Skupinska K, et al. The activity of Selol in multidrug-resistant and sensitive human leukemia cells. Oncol Rep. 2007;18(4): 893–899.
- Praga C, Beretta G, Vigo PL, et al. Adriamycin cardiotoxicity: a survey of 1273 patients. Cancer Treat Rep. 1979;63:827–834.
- Muehlmann L, Ma B, Longo J, et al. Aluminum & phthalocyanine chloride associated to poly(methyl vinyl ether-co-maleic anhydride) nanoparticles as a new third-generation photosensitizer for anticancer photodynamic therapy. Int J Nanomed. 2014;9:1199.
- Longo JPF, Muehlmann LA, Miranda-Vilela AL, et al. Prevention of distant lung metastasis after photodynamic therapy application in a breast cancer tumor model. J Biomed Nanotechnol. 2016;12:689–699.
- de Souza LR, Muehlmann LA, Dos Santos MSC, et al. PVM/MA-shelled selol nanocapsules promote cell cycle arrest in A549 lung adenocarcinoma cells. J Nanobiotechnol. 2014;12:32.
- de Souza LR, Muehlmann LA, Matos LC, et al. Antitumor activity and systemic effects of PVM/MA-shelled selol nanocapsules in lung adenocarcinoma-bearing mice. Nanotechnology. 2015;26:505101.
- dos Santos Câmara AL, Nagel G, Tschiche HR, et al. Acid-sensitive lipidated doxorubicin prodrug entrapped in nanoemulsion impairs lung tumor metastasis in a breast cancer model. Nanomedicine. 2017;12:1751–1765.
- O’Brien MER, Wigler N, Inbar M, et al. Reduced cardiotoxicity and comparable efficacy in a phase III trial of pegylated liposomal doxorubicin HCl (CAELYX/Doxil) versus conventional doxorubicin for first-line treatment of metastatic breast cancer. Ann Oncol. 2004;15:440–449.
- Mitry MA, Edwards JG. Doxorubicin induced heart failure: phenotype and molecular mechanisms. IJC Heart Vasc. 2016;10:17–24.
- Wicki A, Witzigmann D, Balasubramanian V, et al. Nanomedicine in cancer therapy: Challenges, opportunities, and clinical applications. J Control Release. 2015;200:138–157.
- Yuan, F, Dellian, M, Fukumura, D, et al. Vascular permeability in a human tumor xenograft: molecular size dependence and cutoff size. Cancer Res. 1995;55(17):3752–3756.
- Krishna, R, McIntosh, N, Riggs, KW, et al. Doxorubicin encapsulated in sterically stabilized liposomes exhibits renal and biliary clearance properties that are independent of valspodar (PSC 833) under conditions that significantly inhibit nonencapsulated drug excretion. Clin Cancer Res. 1999;5(10):2939–2947.