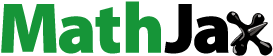
Abstract
Green synthesis approach for nanoparticle is environmental friendly, non-hazardous and the nanoparticles have shown enhanced biocompatibility for application in healthcare. Previous reviews have mentioned about green synthesis methods for nanoparticles and their biological activities. This review not only covers the general information about green synthesis of silver nanoparticles and characterization, but also focused on recent uses of various medicinal and nonmedical plants based AgNPs synthesis and their broad-spectrum antimicrobial and anticancer activities. In addition, this review emphasizes on elaborating underlying mechanism of anti-pathogenic microbial and anticancer activities of plant based AgNPs. Thus, present article provides a comprehensive analysis of plant-mediated synthesis of AgNPs and their potential applications in biomedical field including their mode of action and challenges in a single window.
Introduction
Cancer is the leading death causing diseases reported by United States of America. Deaths due to various types of cancer were mentioned in the World cancer report 2016. Major deaths causing cancer were reported due to lung, liver, stomach, colorectal and breast. It has reported that 1.5 million deaths due to lung cancer, 745,000 deaths in liver cancer, 723,000 deaths in stomach cancer, 694,000 and 521,000 deaths were occurred due to colorectal and breast cancer, respectively [Citation1]. Overall the population of Asia, Africa and the America contribute 70% of all the deaths due to any cancer and 60% for new cancer cases reported worldwide. Currently employed practices for cancer treatments are via surgery, radiation and chemotherapy. These are the conventional way of treatment and have some side effects as well. To overcome these problems and for betterment of the health of cancer patients, scientists all over the globe have been working in this area to explore the new possibilities for cancer treatment.
Similarly, multidrug resistance is constantly increasing with the increasing uses of antibiotics for the treatment of several pathogenic diseases. The use of broad-spectrum antibiotics has created resistance too many of the microbial human pathogens which appears as a major threat for mankind. More than 25% resistance reported in invasive staphylococcal isolates which are known to be methicillin resistance Staphylococcus aureus in some countries, which shows these pathogen required an efficient drug for their control. Candida albicans strains including Candida glabrata and Candida krusei are resistant to commonly used antimycotic drug fluconazole. Emergence of resistance viral strains are creating massive problem in antiretroviral therapy, particularly in case of HIV. Similar resistance problem were observed in case of plant infecting pathogens. Thus, there is an urgency demanding new alternatives for treatment and controlling these infectious pathogens [Citation2].
Due to the smaller size (1–100 nm) and recent advancement in the field of nanoparticles (NPs) gain a significant interest to overcome the limitations of conventional therapies. Their unique physicochemical properties and high surface area to volume ratio silver nanoparticles (AgNPs) have been proved to be a potential candidate for biological activities that includes cancer therapeutics, treatment of wounds, water disinfecting, nano-coating for bone implants, dental surgery and antimicrobial agents. Green synthesis approach for AgNPs is common interest for most of the researchers for its application. Unlike physical and chemical methods green synthesis method is eco-friendly and cost effective, which made it a promising and preferred method over others. Both plants and microbes can be used to reduce the AgNO3 solution to form AgNPs. But plant extracts are much more reliable and advantageous over the use of bacteria due to its toxic effect [Citation2]. It is deprived of any downstream processing and can eliminate some of the bio-safety issues unlike micro-organisms. Plant-based synthesis approach also provides rapid synthesis platform to achieve increased stability and high efficiency of nanoparticles. Green synthesis approach provides the flexibility to produce different sizes of nanoparticles compared to other methods [Citation3].
Considering the literature in this regard, we have tried to focus on plant-based synthesis of AgNPs, its characterization and application. This article describes systematically synthesis and characterization of AgNPs, application in bacterial resistance and anticancer activities in different cancer cells in a single window. Most importantly therapeutic challenges of nanoparticles in anticancer activity and antimicrobial activities were discussed before ending with conclusion and the future perspective of plant based synthesized AgNPs.
Metallic nanoparticles in therapeutics
Last few years there has been a remarkable advancement in nanotechnology research and its application towards therapeutics. In addition to that, medicinal plant research made possible for combating different types of human-threatening diseases with the help of nanotechnology which would ultimately benefit the patient physically and economically. Nanoparticles synthesized from medicinal plants are now the emerging area of research and has attracted most of the researchers and scientists. The main advantage to use nanoparticles is that it can bypass the impermeable membrane and deceive immune response too. There are many types of nanoparticles, especially metallic nanoparticles (MNPs), took a lot of attention from scientists over a century and now these nanoparticles are heavily utilized in biomedical fields. Today metallic nanoparticles can be synthesized easily and can be modified with various chemical functional groups coupled with antibodies, legends, drugs, chemical compounds, etc.
Drugs coated with nanoparticles can be targeted to specific tissues, so that it will achieve very high efficacy and consequently it can be used to encounter different forms of cancer [Citation4]. Many researchers have been using different nanoparticles for targeted drug delivery and gene delivery. The most used nanoparticle for anticancer, antimicrobial activity and drug delivery is gold nanoparticles (AuNPs). AuNPs were tagged with antibodies (Abs) targeting surface of cancer cells which then bind to cancerous cells resulting the death of the malignant cells. AuNPs possesses biocompatibility and hyper thermal activity, so it is the primary choice for researchers to use gold nanoparticles for killing of the cancerous cells [Citation5]. Silver nanoparticles are the well-known agent for antimicrobial activity and its composites are commercially available for biomedical applications. Recent times AgNP has also shown promising result in anticancer activity in various cancer cells, which attracts scientist to expand its application. Other nanoparticles including zinc oxide, iron oxide, copper oxide, and aluminium oxide are the most commonly used metallic nanoparticles for various applications and are focused in different areas of research. Silver and Gold ions are among the most important, flexible, reliable and prominent ones for green synthesis from medicinal plants and their components for therapeutic applications. Whenever the nanoparticles were used for in vivo application its biocompatibility for the normal tissue is an important concern for the researchers in this area. Despite all the significant potential of gold nanoparticles, its safety issue is currently highly controversial. The current researchers have raised this important issue which needs to be addressed properly. Factors like shape, size, surface functionalization, surface charge and its coating agent are expected to influence the biological systems by interacting with protein and peptides. These interactions are resulting in different outcomes in the biological system and hence, negatively influencing the potential of AuNPs for biomedical applications. So, keeping biocompatibility and the cost-effectiveness silver nanoparticles can be an ideal replacement of gold for cancer therapies for the researchers to work on.
Plant extracts were widely used in the formation of various metallic nanoparticles apart from silver and gold for various other important uses. Polladium nanoparticles (PdNPs) were used as catalyst in different reactions. PdNPs were synthesized using the plant extract of Hippophae rhamnoides and Catharanthus roseus leaf extracts shows catalytic activity in Suzuki miyaura coupling reaction in water and photo-catalytic degradation. Plant mediated some other metallic nanoparticle includes copper nanoparticles (CuNPs) formed by using Citrus medica Linn juice showed antimicrobial activity against Escherichia coli, Klebsiella pneumoniae, Propionibacterium acnes, Salmonella typhi and Pseudomonas aeruginosa. These above studies give clear idea about the applicability, advantages and broad aspect of plants in the field of nanotechnology [Citation5,Citation6]. In medicines, silver and silver nanoparticles were used as an ointment to overcome against burn and wound infection. Silver nanoparticles have been reported as having the highest electrical and thermal conductivity than any metal. They have extra ordinary properties like chemical stability, high conductivity and induce both catalytic and biological activities. These unique properties of silver force the researcher to consider over the other metallic nanoparticles for antimicrobial, antiviral, anticancer, antifungal and anti-inflammatory activities. It has been investigated and recognized that it has an ability to inhibit microbial growth present in medical and industrial processes.
Advantages of plant-based synthesis of metallic nanoparticles
There are many advantages of using plant material as reducing agent for the synthesis of silver nanoparticles. These advantages includes: its easy availability, safety towards handling, cost-effectiveness, very low maintenance cost and eco-friendly [Citation7]. It has various metabolites which enhances the reduction reaction resulting in rapid synthesis of nanoparticles and can act as a capping agent which produces very stable nanoparticles for further uses [Citation5]. These phyto-compounds gives the flexibility required for better control over size and shape of the nanoparticles and lastly, it is a one step process which further reduces the complications of the formulation process. After this, other metallic nanoparticles were formulated using various medicinal plants and their extracts. Metallic nanoparticles, such as silver, copper, cobalt, nickel and zinc, have been synthesized using three different plant extracts, namely Medicago sativa (Alfa alfa), Brassica juncea (Mustard), and Heliantus annus (Sunflower). Their metal accumulating ability was assessed, because it is known for certain plants that these can accumulate higher concentrations of metals, called hyper accumulators. From the above metallic nanoparticle synthesis, Brassica juncea (Mustard) is clearly showed higher metal accumulating ability than the others. Nearly 321, 212 plant species exist in earth reported by the Botanic Gardens Conservation International (BGCI) due to which the scientist have been able to access through a significant class of plants for the nanoparticle synthesis purpose. Among them diverse group of plant extracts have the dual properties like reducing as well as stabilizing agents that includes phytochemicals and other plant derivatives (e.g. starch, dextran, alginate, cellulose, chitin, etc.). Due to the oxidation of –OH groups and carbonyl group of the phytochemicals, it can act as a reducing as well as capping agent for the stabilization of metallic nanoparticles. The extracts of these plats might have some medicinal phytochemicals which helps the nanoparticles to be effective on cancerous cells as well as different microorganisms. According to the literature, the reducing phytochemicals includes the plant secondary metabolites, phyto-proteins, phenolic compounds, flavonoids; alkaloids, polysaccharides, sugar compounds; aromatic amines, acidic compounds and much more compounds present in the plant extract [Citation5]. shows the list of plants and its different parts which have been used for green synthesis of AgNPs, size, shape and characterization. Generally, leaf extracts are the primary source for the reduction of Ag+ ions which are commonly used by the researchers. Meanwhile many researchers reported various parts of plants used which are bark, callus, flower, fruit, stem, seed, peel, leaves, petals and rhizome.
Table 1. Showing green synthesis of silver nanoparticles using plant extracts and characterization technique.
Green synthesis approach for AgNPs
Like all the other nanoparticles there are numerous methods to synthesize AgNPs through chemical and physical as well as biological approaches. In one report, these different approaches has been categorised as Bottom Up and Top Down approach [Citation6]. Chemical route to formulate AgNPs requires a chemical reagent to reduce Ag+ ion to Ag0 (e.g. Sodium borohydrate, Sodium citrate etc.). Nonetheless, low biocompatibility issues make these chemical methods inadmissible. Green chemistry approach using plant extract is contemplated as a substitute for chemical synthesis for metal nanoparticles because; the reduction reaction occurs by phytochemical compounds. In modern era, green synthesis is called biogenic synthesis and the era is considered as ‘Phytonanotechnology’. Different parts of plants are being used to synthesize silver nanoparticles optimizing the conditions according to the downstream utilization. The desired size and shape can be obtained by optimizing the solvent type and reduction time. Green synthesis approach of silver nanoparticles is a simple and hasty that includes association of metal ion with a plant material. General protocol for synthesis starts by incubating the AgNO3 salts and reducing agent (plant extract), which causes reduction of Ag+ ion to Ag0 followed by aggregation and capping leads to the formation colloidal silver nanoparticles [Citation6]. Some studies use an external source of energy for incubation to promote the reaction. Reduction reaction takes place due to the presence of biocatalyst in the form of specific parts plant extract. A schematic representation of the synthesis process is depicted in (.
Figure 1. Schematic showing A. various approaches for synthesis of AgNPs. B. One pot green synthesis method for synthesis of AgNPs.
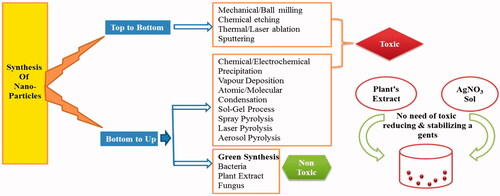
Reaction is as follows:
Parameters that need to be considered while synthesizing AgNPs are pH, temperature, duration of reaction and the proportion of mixing biological extract to AgNO3. These parameters affect the overall outcome of the nanoparticle. Report suggests that adjustment of pH causes zeta potential of nanoparticles changes since the cationic nature of Ag+ ion tends to change due to change in ionic strength of solution. Likewise reaction rate increases by increasing the reaction temperature, which also affecting the thermo stability of reducing compound, thus affecting the yields. Along with the above two parameter time is directly proportional to the rate of reaction in most cases. Lastly, the size and shape of the nanoparticles depends upon the proportion of mixture in which the plant extract and AgNO3 were mixed [Citation7,Citation8].
Phytochemicals involved in AgNPs synthesis
Up to now lot of work has been done in green chemistry method for plant-assisted formulation of metallic nanoparticle and the investigation for the roles of phytochemicals for different plants. Terpenoids, flavonoids, ketones, aldehydes, amides and carboxylic acid were the most important phytochemicals involved in the reduction of metal were identified by spectroscopic studies [Citation7,Citation8]. Some phytochemicals such as flavones, quinones were found and identified as water soluble which are responsible for rapid reduction process. For the synthesis of silver nanoparticles, the phytochemicals present in Xerophytes (Bryophyllum species), Mesophytes (Cyprus species) and Hydrophytes (Hydrilla species) were studied for their role. Bryophyllum species was found to be containing an anthraquinone (emodin) which undergoes tautomerization and causes formation of silver nanoparticles. On the other hand, Cyprus species was found to be containing cyperoquinone, dietchequinone and remirin. Quinones are the tuning factor for size variability. It was suggested that slight heating of the sample resulting activation of quinones and leading to reduction in particle size. From the above study, it was reported that using xerophytes, mesophytes and hydrophytes the size of nanoparticle were in the range of 2–5 nm [Citation9]. On the other hand, plant extracts containing water-soluble phytochemicals requires much lesser time for the same purpose. Therefore, it is clear that plant extracts are more efficient, effective and safer than compared to bacteria and fungi, hence better candidate for the synthesis of silver nanoparticles. It has been introduced fully green nanotechnology as a gateway to beneficiation of natural cellulose fibres. So the above discussion concludes that by using techniques like plant tissue culture and downstream processing metallic as well as oxide nanoparticles can be synthesized on an industrial scale by properly addressing and identifying the metabolic status of respective plant species.
Characterization of AgNPs
Characterizations of green-synthesized nanoparticles are very important to confirm their shape, size and stability even before assessing its toxicity. Various biophysical techniques have been used to characterize green synthesized AgNPs () that includes UV-Visible Spectroscopy (UV-Vis), Dynamic Light Scattering (DLS), Surface Plasmon Resonance (SPR), Microscopy techniques (TEM, SEM), Atomic Force Microscopy (AFM), X-Ray Diffraction (XRD), Fourier transform infrared spectroscopy (FTIR), Energy-Dispersive Analysis (EDAX)etc. Plant-mediated synthesis of AgNPs with their sizes and characterisation techniques were mentioned in the table below ().
Figure 2. Characterization and Application of AgNPs. (A) UV-Visible spectral analysis. (B) TEM micrograph. (C) Particle size analysis by DLS. (D) FTIR spectra. (E) Showing Fluorescent microscopic images showing staining of rhodamine 123 (i) untreated A549 cells, (ii) AgNPs treated A549 cells, (iii) untreated Hep2 cells (iv) AgNPs treated Hep2 cells. (F) Showing quantitative analysis of MMP levels in A549 and Hep2 cells. (G) Showing a zone of inhibition in the presence of AgNPs and antibiotics in E. coli cells. (H) Growth kinetics of E. coli in different concentrations of AgNPs [Citation43].
![Figure 2. Characterization and Application of AgNPs. (A) UV-Visible spectral analysis. (B) TEM micrograph. (C) Particle size analysis by DLS. (D) FTIR spectra. (E) Showing Fluorescent microscopic images showing staining of rhodamine 123 (i) untreated A549 cells, (ii) AgNPs treated A549 cells, (iii) untreated Hep2 cells (iv) AgNPs treated Hep2 cells. (F) Showing quantitative analysis of MMP levels in A549 and Hep2 cells. (G) Showing a zone of inhibition in the presence of AgNPs and antibiotics in E. coli cells. (H) Growth kinetics of E. coli in different concentrations of AgNPs [Citation43].](/cms/asset/f7a6b297-7f45-4588-88f4-24ac1c87f2cd/ianb_a_1489262_f0002_c.jpg)
UV-vis
UV visible spectrometry analysis is commonly used as the primary quantitative analysis for confirming the nanoparticle formation and also used to monitor the stability. UV visible spectroscopy is very simple technique, sensitive and fast. Most importantly it is selective for different nanoparticles Synthesis was monitored by the colour change of AgNO3 from colourless to brownish yellow and its absorption spectra appeared at 410–430 nm. It quantifies the amount of UV or visible radiation observed by the sample in the solution. In nanoparticles, the free moving electron produces surface Plasmon resonance (SPR) spectra due to combined oscillation of electrons of AgNPs with the incident light. Nanoparticles morphology, chemical composition, size and dielectric medium are the determinant for its absorption spectra.
XRD
XRD is widely used to study the crystalline structural lineaments of nanoparticles, various biomolecules, polymers, etc. by giving degree of crystallinity. It will quantify the resolution of different chemical compounds, gives approximate idea of different chemical groups and particle sizes. The working principle behind this analysis is that when a monochromatic beam of X-ray is directed towards the crystal, it produces different diffraction patterns. These diffracted X-rays produces interferences pattern which then analysed by using Bragg’s equation to know the characteristics of crystalline or polycrystalline material. XRD technique can be used in various other bio-molecules such as polymers, superconductors, etc. The quantifications from this technique are made in Angstrom (Å) (1 Å = 0.1 nm). XRD is the primary determinant for characteristic crystal size and its unit dimensions. In case of silver nanoparticles, XRD spectra determine the crystalline nature by overall oxidation state of particles as a function of time.
TEM
TEM is the most common and a very powerful technique to characterize the silver nanoparticles. It is generally used to get the quantitative values of SNPs such as size and morphology. The principle behind TEM is that it uses electron beam which interact with the sample and the image is shown in photographic plate. Resolving power of TEM is very high, so it can detect and quantify individual nanoparticles. Spatial resolution power is the advantage of using TEM over SEM. Development of various high-resolution microscopy techniques is driving the researchers in this field of nano-science and technology to gain information using beam of high energy electrons.
SEM
The principle behind scanning electron microscope (SEM) technique is that the projected electron beams are interacting with the atoms at various depths within the sample. The reflected secondary electrons are captured by detectors translate into images. Presently, among all the microscopy techniques, SEM is most efficient method to resolve different particle size and shapes of nanoparticles. It can also determine the information about surface morphology of nanoparticles in nanoscale. SEM is coupled with energy-disperse X-ray spectroscopy (EDX). One can able to make a histogram of number of particles from the image by manual counting or by using some specific software. It can determine the chemical composition of the particle. As a limitation, SEM cannot determine the internal structure of nanoparticles. The advantage of using SEM is that it can resolve the particle below 10 nm size.
DLS
Dynamic light scattering (DLS) is a technique used for determining the size distribution and also the poly-disperse nature of the nanoparticles in the suspension. This technique is the most common technique that is used for size determination. The working principle behind this technique is that it measures the hydrodynamic radius of the particle in Brownian motion. The particle in the solution when exposed to laser light, it scatters the light in different intensities. By using Stokes–Einstein relationship equation these intensities of fluctuations were analysed, and hence, corresponding particle sizes were obtained. The method is dependent upon the interaction of light to the particle in suspension. DLS is reliable in for the particle size ranges from 20–200 nm. The size obtained from DLS is comparatively larger from results obtained from TEM and SEM, because it measures the size in the solution where the particle is in Brownian motion.
FTIR
Fourier transform infrared (FTIR) spectroscopy is used to evaluate the surface chemistry such as chemical bonds and functional atoms. It also can characterize the physical properties of synthesized nanoparticles so as to find out the biomolecules involved in nanoparticle formation. Researchers used it to know the chemical compound present in the leaf or other plant extract involved in nanoparticle synthesis. Principle behind this technique is that when infrared radiation passed through the sample it absorbs some radiation and non-absorbed radiations are passed away from sample. The spectrum determines the identity of the sample by its absorbance and transmittance value. One of the paper reports that terpenoids or flavonoids whichever are present in the leaf extracts of Prosopis juliflora, may be important factor for the decay of AgNPs synthesis. In another report, AgNPs synthesized using Capsicum annuum extracts, FTIR analysis suggested that the formation of nanoparticles are arbitrate by amine groups or the secondary structure of proteins. Furthermore, FTIR is a non-invasive, suitable, important and very simple technique to explore the role of plant extract in the reduction of silver.
Above characterization techniques are familiar to ensure the determination of AgNPs resulted through various investigations. Off these, TEM appears to be promising in advance science to ascertain the size range of silver nanoparticles, though other techniques supports to understand the salient feature of the nanoparticles.
Therapeutic applications of plant-based AgNPs
Anticancer
AgNPs synthesized from the leaf extract of Mentha pulegium were found cytotoxic to Hela and MCF-7 cancer cells [Citation10]. AgNPs were treated on MCF-7 cell line shows drastic changes in morphological characteristics. The membrane integrity was disrupted and cell growth was arrested. Along with that cytoplasmic shrinkage and cell clustering was observed unlike the case in AgNPs treated normal cells. The cells were treated with 20 μg/mL concentration of AgNPs and kept for 48 h for further observation and found apoptosis of the cells [Citation10]. AgNPs synthesized with Coriandrum sativum and Prosopis cineraria extract as a reducing agent showed anticancer activity against MCF-7 cancer cells [Citation11,Citation14]. Roots of Angelica pubescens a Chinese herbal plant-mediated novel AgNPs having a crystallite size of 12.48 and showing antioxidant activity against 2, 2-diphenyl-1-picrylhydrzyl (DPPH) radicals; antimicrobial activity against human pathogens includes Escherichia coli, Staphylococcus aureus, Pseudomonas aeruginosa and Salmonella enterica. The cytotoxicity of AgNPs was investigated in RAW 264.7 cells and LPS-stimulated RAW 264.7 cell line [Citation12]. AgNPs synthesized by one-step pot method using marine sponge extract (Haliclona exigua) and AgNPs showed antibacterial as well as anticancer activity against oral bio-film bacteria and oral cancer cell lines (KB cells), respectively. Zone of inhibition was 9.1 mm for oral bio-film bacteria and IC50 value was found to be 0.6 μg/ml for oral cancer cell lines [Citation13]. Green AgNPs were synthesized by Andrographis echioides leaf extracts and characterized. Its antibacterial and toxicity were shown by zone of inhibition and MTT assays, respectively. For anticancer activity, they have used MCF-7 and human keratinocyte cell line (HaCaT) [Citation17]. Caffeic acid, which is a natural extract, used successfully to prepare the AgNPs where caffeic acid acts as both a reducing agent and a stabilizer. Further, its anti-cancer activity was assessed against the human hepatoma HepG2 cells. Petals of Cucurbita maxima, leaves of Moringa oleifera and rhizome of Acorus calamus [Citation19] were used for nanoparticle synthesis and the AgNPs showed antibacterial activity against medical isolates. Their toxicity was tested using skin cancer cells (A431). It was reported that the phytochemicals of above plants such as phenols, flavonoids and terpenoids acts as reducing as well as stabilizing agent [Citation7]. Similarly, AgNPs prepared by various other plant extracts from Plumeria alba [Citation21], Abutilon indicum [Citation53], Erythrina indica [Citation25], Indigofera tinctoria [Citation36], Mukia maderaspatna [Citation39] Glycyrrhi zauralensis [Citation41] shows anticancer activity against B16F10, HNGC2, A549, COLO 205, PC-3, A357, MDA-MB-231 cell lines. Some in vivo studies were done to know the antiangiogenic property in rat aortic model where AgNP synthesized from fruit extract of Piper longum was being used [Citation28]. AgNPs formed by Coleus amboinicus extracts shown cytotoxic effect to human oesophageal adenocarcinoma (EAC) cell lines. AgNPs showed 50% at 30 μg/mL and 70% cytotoxicity at 30 and 50 μg/mL, respectively, which suggesting some information about the concentration dependent cytotoxicity [Citation48]. The extensively elaborates on the anticancer properties of plant-based AgNPs synthesized from different plants recent research studies.
It is reported in some in vivo studies that effect of metal nanoparticle to the cell lines strongly depends upon their capping agent. AgNPs differing in capping agents have been reported to be cytotoxic in some cases. Therefore, keeping the challenges in mind plant-based AgNPs can be a really good tool for the researchers to overcome these diseases due to its physic-chemical properties and biological advantages.
Antimicrobial
AgNPs antibacterial properties are known for decades, but their characterisation and quantification on scientific basis started recently. Sundi & Salopek Sondi were reported the first antimicrobial activity of AgNP in 2004 against E. coli. Devi and Joshi in 2012 reported the activity of AgNPs in Staphylococcus aureus, Streptococcus pyogenes, Salmonella enterica and Enterococcus faccalis. These AgNPs shows synergistic effect with erythromycin, methicillin, chloramphenicol and coprfloxicin against klebsilla pneumineae. Size-dependent activities of AgNPs were reported by Shameli et al., 2012, described in Gram-positive and Gramnegative bacteria. Factor affecting the activity of AgNPs against bacteria are concentration, size, shape, concentration of AgNPs combined with other antibiotics [Citation8]. AgNPs synthesized by one-step pot method using marine sponge extract (Haliclona exigua) and this AgNPs showed antibacterial activity against oral biofilm bacteria [Citation13]. Green AgNPs were synthesized by Andrographis echioides leaf extracts and characterized. Its antibacterial and toxicity were shown by zone of inhibition [Citation17]. Biogenic colloidal AgNPs synthesized with Mentha pulegium extract as a reducing agent showed antibacterial activity against Escherichia coli, Staphylococcus aureus, Steptococcus pyogenes, Candida Albicans [Citation10]. AgNPs synthesized from Syzygium jambos showed antibacterial and antifungal properties [Citation37]. Similarly Syzygium cumini synthesized AgNPs showed activity against multidrug-resistant (MDR) pathogens, Salmonella enterica and Stayphylocuccous aureous [Citation45]. Cucurbita maxima, Moringa oleifera and Acorus calamus plant extract-mediated AgNPs showed activity against broad spectrum microbes, for example Pseudomonas aeruginosa, Bacillus subtilis, Escherichia coli and Vibrio cholera [Citation19].
As compared to antibacterial activity, antifungal activity is less studied. This is clear by the number of publication shown in Pubmed Central database. Chemically synthesized AgNP was studied against 44 strains of 6 fungal species, namely Candida tropicalis, Candida glabrata, Candida krusei, Candida parapsilosis and Trichophyton mentagrophytes as reported in literature [Citation8]. Plant-based AgNPs were effective against some plant pathogenic fungi. Jio et al. 2009 studied the effect of AgNPs on two plant pathogenic fungi, namely Bipolaris sorokiniana and Magnaporthe grisea. They have also studied the antifungal activity of AgNPs with combination with other antifungal compounds. AgNPs synthesized from leaf extract of Svensonia hyderobadensis showed high antifungal activity against Aspergillus flavus, Aspergillus niger, Curvularia sp., Fusarium sp. and Rhizopus sp. indicates that AgNPs synthesized from medicinal plant extract have significant action against microbes [Citation8].
AgNPs also act as antiviral agent against HIV-1, Polio virus, respiratory syncytial virus, herpes simplex virus and monkey pox virus. Report suggested the mode of action for AgNPs against HIV-1 virus. Its mechanism is based on the initial stages of HIV-1 cycle [Citation8]. AgNPs binds to the glycoprotein-120 (gp-120) inhibiting the CD-4-dependent binding, thus inhibiting the fusion with cell membrane and infectivity [Citation8]. AgNPs can be a broad-spectrum antiviral agent, because they are not prone to develop resistance against AgNPs. The extensively elaborates the recent reported antimicrobial activity of different plant-induced AgNPs against broad spectrum microbes.
Table 2. Antimicrobial and anticancer activities of green synthesized silver nanoparticles.
Mechanism of anticancer activity
Plant-based AgNPs generally induces reactive oxygen species (ROS) causing the cellular deaths. ROS affecting the signal transduction process pathways which also causes the cellular apoptosis [Citation4]. The generation of peroxide (H2O2) radicals alters the trans-membrane potential of mitochondria causing uncoupling of respiration process. Up-regulation of p53 protein by AgNPs also one of the mechanism proposed by researchers. One of the studies reveals that through western blotting the p53 up-regulation in biogenic AgNPs treated PB16 cell lines [Citation18]. P53 up-regulation occurs by the activation of apoptosis pathway activation. Caspase-3 activation and oxidative stress are the reasons for cell deaths when treated the cells with AgNPs [Citation40]. Researcher reported one recent article showing green synthesis of AgNPs (GSNPs) from Mentha arvensis, when treated induces cytotoxicity to breast cancer cells by mediating caspase 9-dependent apoptosis in MCF-7 cell lines [Citation40]. Silver nanoparticles synthesized from calli cells of Mentha arvensis shows toxicity against Hep 2 cells via caspase 9-dependent apoptosis. The cell cycle analysis shows a significant rise in sub-G1 cell proliferation which illustrates the cytotoxicity of AgNPs [Citation40]. Concentration of silver ion released in cells determines the selective cancer cell deaths. The silver ion release is varied from normal cell lines to cancer cell lines in different pH. At acidic pH, the silver ion release is high. When the AgNPs were incubated both in pH 7.4 and pH 5 buffers, it was seen that silver ion release was more at acidic pH, confirming the selective killing of cancer cells at lower pH. It is also confirmed that the formation of nanoparticle aggregation can control the cervical cancer cell and non-aggregates can increase the cellular viscosity and disturb the important cellular processes that eventually leads to cellular apoptosis [Citation32]. Anti-angiogenic properties of AgNPs are also illustrated by some researchers. In one of the study, green synthesized AgNP shows antiangiogenic activity by inhibiting vascular endothelial growth factor (VEGF) induced cell proliferation. After entering the cell, via Src-dependent pathway AgNPs inhibits VEGF and IL-1β induced vascular permeability [Citation23]. In another report, plant-induced AgNP using extract of Saliva officinalis shows antiangiogenic property of AgNPs can be a new target for cancer therapeutics [Citation4,Citation27]. Recently, one report identified another mechanism suggesting that nanoparticles can induce cellular degradation via autophagy induction and increased autophagy promotes cell death. AgNPs induced autophagy due to the assembly of autophagolysosome in cancer cells, and finally, it caused cell deaths due to its elevated level. Hydrastis canadensis and Thuja occidentalis induce differential cytotoxicity through G2/M arrest in A375 cells [Citation38]. In one of the recent finding suggested that breast cancer can be diagnosed by analysing the concentration of sialic acid in human saliva using surface-enhanced Raman spectroscopy (SERS) of silver nanoparticles. The figure () showing schematic model for the mechanisms involved for anticancer activity of AgNPs.
Mechanism of antimicrobial activity
Silver compounds are regarded as among the most potent and rapidly acting antimicrobial agents. AgNPs have shown effectiveness against broad spectrum microorganisms including multidrug resistant and methicillin resistant bacteria Staphylococcus aureous known as MRSA [Citation2,Citation43]. Some researchers reported through proteomics and biochemical data analysis that nanomolar concentration of AgNPs can cause dissipation of proton motive force (PMF) which leads to rapid inactivation of the bacteria when treated to Escherichia coli. The current medications cannot control the emerging multidrug-resistant pathogens due to their antigenic shifts or drifts. This resistance has become a threat to public health. Silver has been used for long many years as antiseptics even without knowing its mode of action. But now researchers are able to explore some of the mechanisms through which the silver is effective in controlling the activity of pathogenic microorganisms. Both silver nanoparticles and silver ions interfere with the protein structure by acting on its disulphide bonds; further it blocks the functioning of microbes. Silver ions tend to release free radicals inside the host cell which cause the toxicity to bacterial cells and also silver ions interact with cellular DNA and enzymes via electron release mechanism. When AgNPs were exposed to bacterial cell DNA condensation took place due to binding of Ag+ with DNA. AgNPs promotes ROS (Reacting oxygen species) inside the cell. Reports suggest that the production of ROS either inside or outside of the cell can cause damage to the cell membrane [Citation19]. Some reports suggests that silver ions also can able to interact with nucleic acids specifically with nucleoside of the inside the microbial cell. Thiol (–SH) group is the binding target for AgNPs forming an S-Ag and subsequently leading to enzyme deactivation required for energy generation and electron transport [Citation20]. Cell lysis is the common mechanism for antimicrobial activity of silver NPs, because silver ions are positive and cell membrane possesses negative charge promoting the electrostatic attraction to takes place between them. The AgNPs then got deposited in the cell membrane and finally enter to the cell by disrupting the membrane. When bacterial cell is exposed to silver nanoparticles, it results in GSH (glutathione) depletion, due to which it cannot protect the cell from harmful redox reactions by ROS molecule. The AgNPs modulates phosphotyrosine profile of peptides present in microorganisms leading to failure of signal transduction system and quorum sensing; finally growth of microorganisms is inhibited [Citation22,Citation23]. The resistance of silver nanoparticles in Gram-positive bacterial pathogens are high due to their thick peptidoglycan layer unlike Gram-negative bacteria. So the concentration is very important determinant the effectiveness of silver nanoparticles to combat microorganisms [Citation3]. showing the schematic model for mechanisms involved in anti-microbial activity of AgNPs.
Figure 4. Schematic model representation of mechanisms and mode of action of AgNPs in microbial cells.
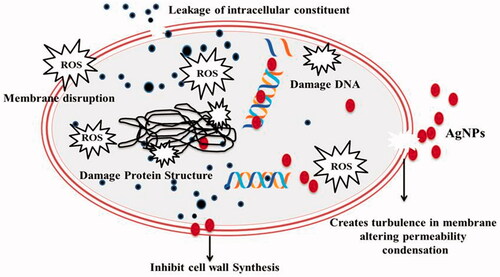
In addition to that, modified AgNPs were also screened for its antimicrobial activities. The modification includes detergents like SDS and Tween 80 and surfactants such as polymer and PVP which significantly increases the AgNPs activity. Mechanistic study reveals that SDS provides more stability than others due to its ionic property. It helps in destroying the bacterial cell wall of gram positive strains [Citation2]. PVP and other polymers are helps in stabilization of nanoparticle because it plays an important role to avoid the particles from aggregation. Reports suggest that AgNPs shows higher activity than AuNPs due to their higher oxidant property and tends to easily lose electrons. To compare this phenomenon DPPH (2, 2-diphenyl-1-picrylhydrazyl) was used to check the activity of AgNPs and AuNPs. DPPH is used for evaluations of antioxidant potential of these nanoparticles. AgNPs showed significantly higher antioxidant activity than AuNPs. The NPs with larger size and spherical shape accept electron easily from hydroxyl radical. The AuNPs and AgNPs radical inhibition is 47% and 52% as reported [Citation2]. Above discussion summarizes the mechanism of action of AgNPs and how the surfactant molecules enhance its mode of action obtained from different studies.
Therapeutic challenges of AgNPs
Major challenge for green AgNPs before forwarding to human trials are to confirm its cytotoxicity level in all kinds of cells, should be compatible, degradable and less or no side effects. Most of the on-going research in AgNPs and its cytotoxicity are in vitro assays and shows non-toxic effect in various cells which is significantly differs from the in vivo experiments [Citation20]. But not much work has been reported regarding toxicity of AgNPs in animal models. One recent work reported that Ficus religiosa-mediated AgNPs are tested in rats and no significant changes was observed, so its biocompatible nature might be useful in nanomedicine [Citation43]. Reports also suggested that the toxicity of the AgNP depends upon it size, concentration, shape and the reducing chemicals which were used. Cytotoxicity mostly depends upon the size and surface area of nanoparticles; smaller nanoparticles have larger surface area. In biological system, application of AgNPs can have interaction with various proteins which could lead to unexpected effects and interfering the normal biological activities [Citation53]. So to overcome this concern, scientist have to discover about the interaction of AgNPs with proteins in-vivo for better understanding and hence confidence to use it in the cancer therapy. Apart from the above discussed challenges some limitations should be addressed for better effectiveness of nanoparticles; these are (i) reducing physiological barriers (ii) enhancing permeability and (iii) its retention in the cell [Citation52].
Challenges for antimicrobial activity
Currently, there is a debate in the scientific community that whether the biological activities shown by the silver nanoparticles are contributed via released Ag+ ions or due to some specific nanoparticle properties. Many studies have shown the particle-specific toxicity of silver nanoparticle using systems (e.g., micro-organisms, human cell lines, zebra fish etc.) where they have shown AgNPs are more toxic to the cells than silver ions at equivalent concentrations [Citation3,Citation8]. However, more definitive study explaining environmental and toxicological effect of Ag+ ion and AgNPs would answer this question [Citation53]. Concentration-dependent doses to both Gram-positive and Gram-negative micro-organisms needs to be used as Gram-positive microorganism possess much thicker cell wall than Gram-negative microbes. A quantitative study suggests that the antimicrobial activity of small AgNPs (d < 10 nm) shows high inhibition due to its higher release of Ag+ ions. On the other hand, they took very high concentration of larger AgNPs and centrifuged and used only the pellet fraction containing particle not the supernatant containing Ag+ ions. It shows comparable result with small AgNPs. These observations led to conclusion that exact nanoparticle properties responsible for antimicrobial effect are still remained unanswered. Now development of ultra-stable AgNPs is gaining more attention to its uniqueness, so the use of ultra-stable AgNPs may clarify these issues [Citation52]. Although recent research in nanotechnology explored several mechanisms for antimicrobial activity of AgNPs and making a strong case for medical application as antibiotic replacements, but due to the variation in the literature, most mechanism is not fully understood and generalized in microbes. The most challenging aspect of AgNPs as antimicrobial agent is that, AgNPs cannot discriminate between beneficial and harmful microbes in a sample. It will act on both the microbes. So, further studies need for it to be targeted for specific microorganisms. Due to the variation literature reported around the world, it is very hard to incorporate the existing knowledge to a result oriented alternative antibiotic application [Citation53].
Practical uses of AgNPs in therapeutics
Robbert burrel (London, UK) developed first nanosilver product to treat various wounds including severe burns, chronic ulcers, epidermal necrosis and pemphigus. Another composite of AgNP and chitosan is used for better wound-healing ability with less infection. For cardiovascular implant nano-composite used as surface coating agent for heart valves and stents that showed antithrombogenic and antibacterial properties. AgNPs are useful for the catheter design due its anti-microbial properties. Silverline (Hamburg, Germany) and ON-Q-Silver SoakerTM (USA) are the two commercially available catheters containing AgNP to prevent infections. Medical catheters are prone to bacterial infection which can lead to serious medical complications so AgNP is useful component to avoid the infection. Apart from above-mentioned applications, AgNPs are used in various other therapeutic applications in medicinal field like dental materials, bio-diagnosis, drug carrier and also development of antibacterial membrane filters for water purification.
Future therapeutic prospective
Last 10–12 years green synthesis approach through plants has achieve enormous attention in the field of nanoscience, because they are the natural reservoir of bioactive phytochemicals which have unique property of reducing silver and can produce AgNPs of various sizes and applications. Due to their broad spectrum activity against various microbes as well as cancer cells which makes AgNPs not only for treatment purposes but also for control of the diseases [Citation53,Citation54]. After reviewing recent studies about the application of silver nanoparticles, it leads to address several issues and limitations. (i) Synthesis methods of AgNPs with optimal properties which make it efficient for both antimicrobial and anticancer agent and minimizing potential toxicity on human or animal cell lines. (ii) Detection of specific microbes against which AgNPs can be a potential drug applied either alone or in combination with conventional drug. (iii) Detection of possible target among the various cancer cells which can be effectively targeted. (iv) Producing universal clinically accepted form of AgNPs for antimicrobial as well as anticancer applications. (v) Involvement of other entities like biological fluid, oxygen pressure and other chemical and biological factors on the activity of AgNPs should be deeply evaluated. Though plant based AgNPs have shown promising result in vitro, further research is needed in animal models to get a more clear and comprehensive picture of its biocompatibility, toxicity and side effects if any [Citation52,Citation54]. Combination therapy would be an alternative and efficient approach for targeted therapy for different cancer cells and for antimicrobial activity. Shakti et al. reported hybrid nanoparticle for cytotoxicity and inhibition of angiogenesis in oral cancer stem cells by bioactive quinacrine and Ag. These hybrid nanoparticles caused apoptosis in OSCC − CSCs by inhibiting the angiogenesis and BER in cells. Inhibition of angiogenesis was shown by fertilized egg [Citation55]. If these studies achieve positive results, it would be a big boost in the researchers in the field of nanoscience and technology to develop more efficient, safer and compatible anticancer and antimicrobial AgNPs. As mentioned earlier, some of the new life-threatening disease causing organisms such as avian influenza, MERS, Ebola virus, Zika virus, etc., are being exposed around the globe. These diseases are very new and understanding the mechanism of infection is very difficult, so to overcome their infection in the affected area and their treatment, nanotechnology would be an effective tool. Therefore, keeping the challenges in mind plant-based AgNPs would become a really good tool for the researchers to overcome these diseases due to its physicochemical properties and biological advantages. The other advantage of Ag particle is the extensive photothermal activity. It can capture the heat from near infra radiation (NIR) and activated. When it will release the heat inside the infected cells, cells will be died due to heat. So combination of AgNP and NIR exposure will produce double effect inside the cells which will ultimately help to destroy the infected cells.
Conclusion
The article accomplishes the investigation of the prospective and application of AgNPs for its anticancer and antimicrobial approach by utilizing the different parts of plants of numerous sources. The nanoparticles synthesized from different plant extracts have yielded significant results in biomedical application combating the broad-spectrum pathogenic microbes and also different types of cancerous cells. Source of plants used for AgNPs synthesis found to have special role for resulting positive outputs of AgNPs in both microbial and cancerous cells. In consequence, cancer cell lines and its decreased cytotoxicity against normal cells make it a promising candidate for future cancer treatment. Furthermore, there is the possibility for the application of plant-based AgNPs on human welfare which could be considered after evaluating its adoption in both positive and negative aspect. Though the success of biogenic AgNPs on various in vitro and in vivo studies generates enthusiasm for its application, still lots of scope for research in this area specifically controlling the size and shape of AgNPs by optimizing the synthesis condition using suitable technique to reduce its toxicity and target oriented delivery for achieving productive transformation of AgNPs.
Disclosure statement
No potential conflict of interest was reported by the authors.
Additional information
Funding
References
- Rao PV, Nallappan D, Madhavi K, et al. Phytochemicals and biogenic metallic nanoparticles as anticancer agents. Oxid Med Cell Longev. 2016;2016:1–15.
- Khan ZU, Khan A, Chen Y, et al. Biomedical applications of green synthesized Nobel metal nanoparticles. J Photochem Photobiol B. 2017;173:150–164.
- Okafor F, Janen A, Kukhtareva T, et al. Green synthesis of silver nanoparticles, their characterization, application and antibacterial activity. Ijerph. 2013;10:5221–5238.
- Ovais M, Khalil AT, Raza A, et al. Green synthesis of silver nanoparticles via plant extracts: beginning a new era in cancer theranostics. Nanomedicine (Lond). 2016;11:3157–3177.
- Mittal AK, Chisti Y, Banerjee UC. Synthesis of metallic nanoparticles using plant extracts. Biotechnol. Adv. 2013;31:346–356.
- Nath D, Banerjee P. Green nanotechnology – a new hope for medical biology. Environ Toxicol Pharmacol. 2013;36:997–1014.
- Fahmy TY, Mobarak F. Green nanotechnology: a short cut to beneficiation of natural fibers. Int J Biol Macromol. 2011;48:134–136.
- Rai M, Kon K, Ingle A, et al. Broad-spectrum bioactivities of silver nanoparticles: the emerging trends and future prospects. Appl Microbiol Biotechnol. 2014;98:1951–1961.
- Mashwani ZUR, Khan MA, Khan T, et al. Applications of plant terpenoids in the synthesis of colloidal silver nanoparticles. Adv Colloid Interface Sci. 2016;234:132–141.
- Kelkawi AH, Kajani AA, Bordbar AK. Green synthesis of silver nanoparticles using Mentha pulegium and investigation of their antibacterial, antifungal and anticancer activity. IET Nanobiotechnol. 2017;11:370–376.
- Jinu U, Gomathi M, Saiqa I, et al. Green engineered biomolecule-capped silver and copper nanohybrids using Prosopis cineraria leaf extract: Enhanced antibacterial activity against microbial pathogens of public health relevance and cytotoxicity on human breast cancer cells (MCF-7). Microb Pathog. 2017;105:86–95.
- Markus J, Wang D, Kim YJ, et al. Biosynthesis, characterization, and bioactivities evaluation of silver and gold nanoparticles mediated by the roots of Chinese herbal Angelica pubescens Maxim. Nanoscale Res Lett. 2017;12:46.
- Inbakandan D, Kumar C, Bavanilatha M, et al. Ultrasonic-assisted green synthesis of flower like silver nanocolloids using marine sponge extract and its effect on oral biofilm bacteria and oral cancer cell lines. Microb Pathog. 2016;99:135–141.
- Sathishkumar P, Preethi J, Vijayan R, et al. Anti-acne, anti-dandruff and anti-breast cancer efficacy of green synthesised silver nanoparticles using Coriandrum sativum leaf extract. J. Photochem. Photobiol. B Biol. 2016;163:69–76.
- Du J, Singh H, Yi TH. Antibacterial, anti-biofilm and anticancer potentials of green synthesized silver nanoparticles using benzoin gum (Styrax benzoin) extract. Bioprocess Biosyst Eng. 2016;39:1923–1931.
- Abbai R, Mathiyalagan R, Markus J, et al. Green synthesis of multifunctional silver and gold nanoparticles from the oriental herbal adaptogen: Siberian ginseng. Int J Nanomed. 2016;11:3131–3143.
- Elangovan K, Elumalai D, Anupriya S, et al. Phyto mediated biogenic synthesis of silver nanoparticles using leaf extract of Andrographis echioides and its bio-efficacy on anticancer and antibacterial activities. J Photochem Photobiol B Biol. 2015;151:118–124.
- Arunachalam KD, Arun LB, Annamalai SK, et al. Potential anticancer properties of bioactive compounds of Gymnema sylvestre and its bio-functionalized silver nanoparticles. Int J Nanomed. 2015;10:31–41.
- Nayak D, Pradhan S, Ashe S, et al. Biologically synthesised silver nanoparticles from three diverse family of plant extracts and their anticancer activity against epidermoid A431 carcinoma. J Colloid Interface Sci. 2015;457:329–338.
- Patra S, Mukherjee S, Barui AK, et al. Green synthesis, characterization of gold and silver nanoparticles and their potential application for cancer therapeutics. Mater Sci Eng C. 2015;53:298–309.
- Mata R, Nakkala JR, Sadras SR. Catalytic and biological activities of green silver nanoparticles synthesized from Plumeria alba (frangipani) flower extract. Mater Sci Eng C. 2015;51:216–225.
- Ramar M, Manikandan B, Marimuthu PN, et al. Synthesis of silver nanoparticles using Solanum trilobatum fruits extract and its antibacterial, cytotoxic activity against human breast cancer cell line MCF 7. Spectrochim Acta, Part-A Mol Biomol Spectrosc. 2015;140:223–228.
- Manikandan R, Manikandan B, Raman T, et al. Biosynthesis of silver nanoparticles using ethanolic petals extract of Rosa indica and characterization of its antibacterial, anticancer and anti-inflammatory activities. Spectrochim. Acta, Part A Mol Biomol Spectrosc. 2015;138:120–129.
- Venkatesan B, Subramanian V, Tumala A, et al. Rapid synthesis of biocompatible silver nanoparticles using aqueous extract of Rosa damascena petals and evaluation of their anticancer activity. Asian Pac J Trop Med. 2014;7:S294–S300.
- Sre PR, Reka M, Poovazhagi R, et al. Antibacterial and cytotoxic effect of biologically synthesized silver nanoparticles using aqueous root extract of Erythrinaindica lam. Spectrochim Acta, Part A Mol Biomol Spectrosc. 2015;135:1137–1144.
- Brahmachari G, Sarkar S, Ghosh R, et al. Sunlight-induced rapid and efficient biogenic synthesis of silver nanoparticles using aqueous leaf extract of Ocimum sanctum Linn. with enhanced antibacterial activity with enhanced antibacterial activity. Org Med Chem Lett. 2014;4:18–28.
- Baharara J, Namvar F, Ramezani T, et al. Green synthesis of silver nanoparticles using Achillea biebersteinii flower extract and its anti-angiogenic properties in the rat aortic ring model. Molecules. 2014;19:4624–4634.
- Reddy NJ, Vali DN, Rani M, et al. Evaluation of antioxidant, antibacterial and cytotoxic effects of green synthesized silver nanoparticles by Piper longum fruit. Mater Sci Eng C. 2014;34:115–122.
- Baghbani-Arani F, Movagharnia R, Sharifian A, et al. Photo-catalytic, anti-bacterial, and anti-cancer properties of phyto-mediated synthesis of silver nanoparticles from Artemisia tournefortiana Rchb extract. J Photochem Photobiol B Biol. 2017;173:640–649.
- Sulaiman GM, Mohammed WH, Marzoog TR, et al. Green synthesis, antimicrobial and cytotoxic effects of silver nanoparticles using Eucalyptus chapmaniana leaves extract. Asian Pac J Trop Biomed. 2013;3:58–63.
- Salehi S, Shandiz SA, Ghanbar F, et al. Phytosynthesis of silver nanoparticles using Artemisia marschalliana Sprengel aerial part extract and assessment of their antioxidant, anticancer, and antibacterial properties. Int J Nanomedicine. 2016;11:1835–1846.
- Soshnikova V, Kim YJ, Singh P, et al. Cardamom fruits as a green resource for facile synthesis of gold and silver nanoparticles and their biological applications. Artif Cells Nanomed Biotechnol. 2017;0:1–10.
- Bhuvaneswari R, Xavier RJ, Arumugam M. Facile synthesis of multifunctional silver nanoparticles using mangrove plant Excoecaria agallocha L. for its antibacterial, antioxidant and cytotoxic effects. J Parasit Dis. 2017;41:180–187.
- He Y, Li X, Wang J, et al. characterization and evaluation cytotoxic activity of silver nanoparticles synthesized by Chinese herbal Cornus officinalis via environment friendly approach. Environ Toxicol Pharmacol. 2017;56:56–60.
- Saratale RG, Benelli G, Kumar G, et al. Bio-fabrication of silver nanoparticles using the leaf extract of an ancient herbal medicine, dandelion (Taraxacum officinale), evaluation of their antioxidant, anticancer potential, and antimicrobial activity against phytopathogens. Environ Sci Pollut Res. 2017;2017:1–15.
- Vijayan R, Joseph S, Mathew B. Indigofera tinctoria leaf extract mediated green synthesis of silver and gold nanoparticles and assessment of their anticancer, antimicrobial, antioxidant and catalytic properties. Artif Cells Nanomed Biotechnol. 2017;0:1–11.
- Dutta PP, Bordoloi M, Gogoi K, et al. Roy. Antimalarial silver and gold nanoparticles: Green synthesis, characterization and in vitro study. Biomed Pharmacother. 2017;91:567–580.
- Das S, Das J, Samadder A, et al. Biosynthesized silver nanoparticles by ethanolic extracts of Phytolacca decandra, Gelsemium sempervirens, Hydrastis canadensis and Thuja occidentalis induce differential cytotoxicity through G2/M arrest in A375 cells. Colloids Surf B Biointerfaces. 2013;101:325–336.
- Devi GK, Sathishkumar K. Synthesis of gold and silver nanoparticles using Mukia maderaspatna plant extract and its anticancer activity. IET Nanobiotechnol. 2017;11:143–151.
- Banerjee PP, Bandyopadhyay A, Harsha SN, et al. Mentha arvensis (Linn.)-mediated green silver nanoparticles trigger caspase 9-dependent cell death in MCF7 and MDA-MB-231 cells. Bctt. 2017;9:265–278.
- Huo Y, Singh P, Kim YJ, et al. Biological synthesis of gold and silver chloride nanoparticles by Glycyrrhi zauralensis and in vitro applications. Artif Cells Nanomed Biotechnol. 2017;0:1–13.
- Dehghanizade S, Arasteh J, Mirzaie A. Green synthesis of silver nanoparticles using Anthemis atropatana extract. Characterization and in Vitro Biological Activities. Artif Cells Nanomed Biotechnol. 2017;0:1–9.
- Nakkala JR, Mata R, Sadras SR. Green synthesized nano silver: Synthesis, physicochemical profiling, antibacterial, anticancer activities and biological in vivo toxicity. J Colloid Interface Sci. 2017;499:33–45.
- Rasheed T, Bilal M, Iqbal HM, et al. Green biosynthesis of silver nanoparticles using leaves extract of Artemisia vulgaris and their potential biomedical applications. Colloids Surf B Biointerfaces. 2017;158:408–415.
- Balakumaran MD, Ramachandran R, Kalaichelvan PT. Exploitation of endophytic fungus, Guignardia mangiferae for extracellular synthesis of silver nanoparticles and their in vitro biological activities. Microbiol Res. 2015;178:9–17.
- Bhattacharyya SS, Das J, Das S, et al. Rapid green synthesis of silver nanoparticles from silver nitrate by a homeopathic mother tincture Phytolacca Decandra. J Chin Integr Med. 2012;10:546–554.
- Otunola GA, Afolayan AJ, Ajayi EO, et al. Characterization, antibacterial and antioxidant properties of silver nanoparticles synthesized from aqueous extracts of Allium sativum, Zingiber officinale and Capsicum frutescens. Phcog Mag. 2017;13:201–208.
- Vadivel S, Suja S. Green synthesis of silver nanoparticles using Coleus amboinicus lour, antioxidant activity and in vitro cytotoxicity against Ehrlich’s Ascite carcinoma. J Pharm Res. 2012;55:1268–1272.
- Das J, Das MP, Velusamy P. Sesbania grandiflora leaf extract mediated green synthesis of antibacterial silver nanoparticles against selected human pathogens. Spectrochimica Acta Part A Molecular and Biomolecular Spectrosc. 2013;104:265–270.
- Sankar R, Karthik A, Prabu A, et al. Origanum vulgare mediated biosynthesis of silver nanoparticles for its antibacterial and anticancer activity. Colloids Surf B Biointerfaces. 2013;108:80–84.
- Dipankar C, Murugan S. The green synthesis, characterization and evaluation of the biological activities of silver nanoparticles synthesized from Iresine herbstii leaf aqueous extracts. Colloids Surf. B Biointerfaces. 2012;98:112–119.
- Mathur P, Jha S, Ramteke S, et al. Pharmaceutical aspects of silver nanoparticles. Artif Cells Nanomed Biotechnol. 2017;12:1–2.
- Park J, Lim DH, Lim HJ, et al. Size dependent macrophage responses and toxicological effects of Ag nanoparticles. Chem Commun (Camb). 2011;47:4382–4384.
- Ge L, Li Q, Wang M, et al. Nanosilver particles in medical applications: synthesis, performance, and toxicity. Int J Nanomed. 2014;9:2399.
- Satapathy SR, Siddharth S, Das D, et al. Enhancement of cytotoxicity and inhibition of angiogenesis in oral cancer stem cells by a hybrid nanoparticle of bioactive quinacrine and silver: implication of base excision repair cascade. Mol Pharmaceutics. 2015;12:4011–4025.