Abstract
Excessive bone resorption induced by abnormal osteoclast differentiation has been associated with bone microstructure damage and bone-associated disorders, including osteoporosis. Here, we investigated the physiological roles of the type 1 cysteinyl leukotriene receptor (cysLTR-1) and the pharmacological functions of the specific cysLTR-1 antagonist montelukast on M-CSF- and RANKL-induced osteoclast differentiation. We showed that cysLTR-1 but not cysLTR-2 is expressed in osteoclast precursor cells: mouse bone marrow-derived macrophages (BMMs). We also found that treatment with M-CSF and RANKL significantly increased expression of cysLTR-1. Overexpression of cysLTR-1 promoted osteoclast differentiation of BMMs by increasing NFATc1 and TRAP. In contrast, treatment with montelukast prevented M-CSF- and RANKL-induced osteoclast differentiation of BMMs. Mechanically, our findings demonstrate that montelukast treatment attenuated activation of the ERK1/2, p38, JNK and NF-κB signalling pathways. Additionally, we reported that montelukast treatment ameliorated the generation of ROS and calcium signalling. Importantly, the co-immunoprecipitation assay displayed that montelukast treatment prevented the interaction of RANK and TRAF6. Finally, in vivo experiments indicated that montelukast rescued the reduction of bone volume as well as trabecular number in an ovariectomy mouse model.
Introduction
Bone is an extensively dynamic structure, which is continuously remodelled to maintain homeostasis [Citation1]. Bone remodelling is mainly regulated by osteoblasts and osteoclasts. Osteoclasts play a pivotal role in bone resorption, while osteoblasts are responsible for bone formation [Citation2]. Excessive bone resorption caused by abnormal activation of osteoclasts results in bone diseases such as rheumatoid arthritis and osteoporosis [Citation3]. Osteoclasts are derived from myeloid lineage cells by cellular differentiation caused by physiological factors, such as macrophage-colony stimulating factor (M-CSF) and receptor activator of nuclear factor κB (NF-κB) ligand (RANKL) [Citation4]. M-CSF is important for maintaining the proliferation and survival of osteoclast precursor cells [Citation5]. RANKL initiates osteoclast differentiation by activating mitogen-activated protein (MAP) kinases and NF-κB by recruiting TNF receptor-associated factor 6 (TRAF6) to its specific receptor RANK [Citation6]. Additionally, phosphorylation of phospholipase Cγ (PLCγ) activates the Ca2+/NFATc1 signalling pathway, which is important in osteoclastogenesis [Citation7]. RANKL also induces the expression of tartrate-resistant acid phosphatase (TRAP), which is a marker gene of osteoclastogenesis [Citation8]. The regulatory mechanisms of osteoclast differentiation are complex. Successful identification of novel signalling pathways governing osteoclast differentiation is helpful for exploring therapeutic targets for osteoporosis.
Cysteinyl leukotrienes (cysLTs) are a family of lipid mediators derived from arachidonic acid. The physiological functions of cysLTs are mediated by their two receptors: the type 1 and type 2 cysteinyl leukotriene receptors (cysLTR-1, -2) [Citation9]. CysLTs such as LTD4 have been shown to be involved in the pathological development of various disorders, including asthma, atherosclerosis and inflammatory bowel disease [Citation10]. Interestingly, activation of cysLTR-1 and cysLTR-2 plays a pivotal role in pro-inflammatory reactions in diverse cells and tissues [Citation11,Citation12]. In the past decades, cysteinyl leukotrienes and their receptors have been extensively studied. Interestingly, montelukast, a potent and specific antagonist of cysLTR-1 was licensed by the U.S. food and drug administration (FDA) for the treatment of bronchial asthma [Citation13]. The therapeutic activity of montelukast is achieved through antagonism of cysLT1R. Increasing evidence has shown that montelukast possesses a wide range of anti-inflammatory abilities [Citation14]. For example, montelukast exerted an anti-inflammatory action in type 2 helper CD4+ T-lymphocytes [Citation15]. Additionally, administration of montelukast could reduce infarct volume and prevent behavioural impairments after stroke in mice and rats [Citation16]. However, little information regarding the effects of cysLTs and montelukast in bone homeostasis has been reported before. In the present study, we set out to evaluate the pharmacological roles of montelukast on osteoclast differentiation and attempted to explore the molecular mechanisms.
Materials and methods
Mouse bone marrow-derived macrophage (BMM) isolation and culture
The BMMs were isolated from murine bone marrow from five-week-old C57BL/six mice following the protocol reported in previous studies [Citation17]. Briefly, erythrocytes were washed away. Cells were maintained in α-MEM (24 h). Non-adherent bone marrow cells were collected and incubated with M-CSF (30 ng/ml) for 3 days. Non-adherent cells were then washed away and bone marrow-derived macrophages (BMMs, adherent cells) were used for subsequent studies. BMMs were maintained in α-MEM.
Ex vivo osteoclastogenesis
To induce osteoclast differentiation, isolated BMMs were incubated with 50 ng/ml RANKL and 20 ng/ml M-CSF for 1 day, 3 days and 6 days. Cells were then fixed with 4% polyformaldehyde and stained with TRAP as published previously [Citation18].
Real-time PCR analysis
The RNA was extracted from BMMs with TRIZOL (Life Technologies, Carlsbad, CA). cDNA was made via reverse transcription PCR reaction with 2 μg of total RNA. One microgram of synthesized cDNA was used for quantitative real-time PCR on an ABI StepOne Plus instrument (Applied Biosystems, Foster City, CA) with a TaqMan assay. The relative levels of target genes were represented by normalizing to GAPDH using the 2−ΔΔCt method.
Co-immunoprecipitation and Western blot analysis
After the indicated treatment, BMMs were harvested with cell lysis buffer (Thermo Scientific, Waltham, MA). The concentration of lysates was measured using the BCA method (Thermo Scientific, Waltham, MA). For immunoprecipitation, protein extract (0.8 ml) was incubated with TRAF6 antibody (0.5 μg) and GammaBind G Plus-Sepharose (25 μl) (4 °C, 2 h). Beads were then washed away. Samples were denatured by boiling at 95 °C for 5 min. Equal amounts (20 μg) of each sample were subjected to 4–12% Bis-Tris gel and electrotransferred onto PVDF membranes. Samples were blocked with 5% BSA. Membranes were then probed with primary antibodies (4 °C, overnight) and HRP-conjugated secondary antibodies (1 h, RT). Blots were developed with enhanced chemiluminescence (Thermo Scientific, Waltham, MA).
Assessment of reactive oxygen species (ROS)
The levels of intracellular ROS in BMMs were determined using the DCFH-DA method (Invitrogen, Carlsbad, CA). Upon completion of the indicated incubation, BMMs were washed three times and probed with 5 μM DCFH-DA for 20 min in darkness at 37 °C. Fluorescent signals were then recorded with a Zeiss fluorescence microscopy (Germany). Intracellular levels of ROS were calculated using Image-Pro Plus software (Media Cybernetics, Inc., Rockville, MD).
Ovariectomized (OVX) mouse model
Ovariectomized mouse models were made as described previously [Citation19]. All experimental procedures were approved by the ethics committee at Linyi People’s Hospital. Briefly, 24 C57BL/six mice (9 weeks old, female) were used to make OVX models. Three groups of mice were prepared: sham group, OVX group and OVX +30 mg/kg montelukast group. Femurs and lumbar vertebrae from mice were used for histomorphometric analysis. Three-dimensional microcomputed tomography and bone morphometric analyses were carried out as described previously [Citation20].
Statistical analysis
Data are represented as means ± standard error. Statistical analysis was performed using ANOVA. p < .05 was considered as statistically significant.
Results
Firstly, we examined the expression patterns of cysLTR-1 and cysLTR-2 in BMMs with a positive control (human A431 cells). Our results indicate that cysLTR-1 could be detected in BMM cells at both the gene () and protein levels (). However, cysLTR-2 was expressed in BMMs at neither the gene () nor the protein levels ().
Figure 1. CysLTR-1 but not cysLTR-2 is expressed in BMM cells. Human SH-SY5Y cells were used as a positive control. (A) Gene expression of cysLTR-1 could be detected in BMMs; (B) Protein expression of cysLTR-1 could be detected in BMMs; (C) Gene expression of cysLTR-2 could not be detected in BMMs; (D) Protein expression of cysLTR-2 could not be detected in BMM cells.
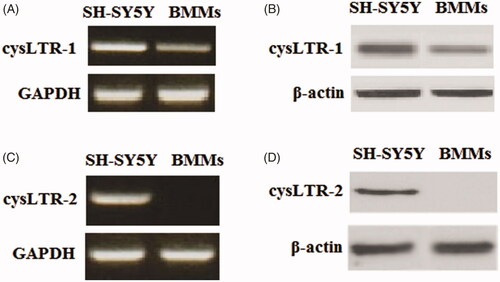
To induce osteoclast differentiation, isolated BMMs were treated with 50 ng/ml RANKL and 20 ng/ml M-CSF for 1, 3 and 6 days. The real-time PCR results shown in indicate that the expression of NFATc1 and TRAP was significantly increased in response to treatment with RANKL and M-CSF. Interestingly, we found that cysLTR-1 was significantly increased at both the mRNA () and protein levels () during the process of osteoclast differentiation. These data suggest that cysLTR-1 may be involved in osteoclast differentiation.
Figure 2. CysLTR-1 expression was increased during the process of osteoclast differentiation. BMMs were cultured with 50 ng/ml RANKL and 20 ng/ml M-CSF for 1, 3, or 6 days. (A) Expression of NFATc1 and TRAP; (B) CysLTR-1 was significantly upregulated in response to RANKL and M-CSF treatment at the mRNA level; (C) Western blot analysis revealed that cysLTR-1 was significantly upregulated in response to RANKL and M-CSF treatment at the protein level (*, p < .01 vs. vehicle group).
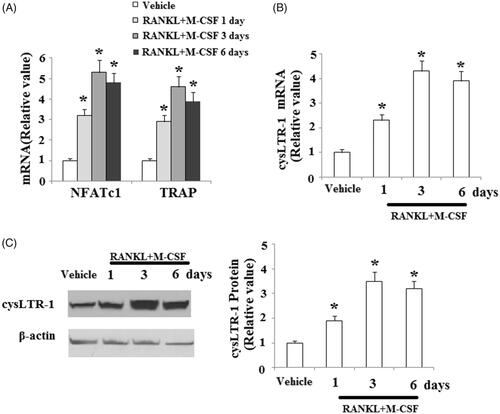
To investigate whether cysLTR-1 influenced the process of osteoclast differentiation, BMMs were transfected with cysLTR-1. Overexpression of cysLTR-1 is shown in . At day 3 after incubation with RANKL and M-CSF, the expressions of NFATc1 and TRAP were significantly higher in cysLTR-1 overexpressing cells (). To further confirm the effects of cysLTR-1 in osteoclast differentiation, the cysLTR-1-specific antagonist montelukast was used. The results in indicate that montelukast significantly reduced RANKL and M-CSF-induced expression of NFATc1 and TRAP.
Figure 3. Overexpression of cysLTR-1 promoted osteoclast differentiation. (A) Successful overexpression of cysLTR-1 is shown in Figure 1(A); (B) Overexpression of cysLTR-1 markedly increased NFATc1 and TRAP at the mRNA level; (C) Overexpression of cysLTR-1 markedly increased NFATc1 and TRAP at the protein level (*, #, p < .01 vs. previous column group).

Figure 4. The cysLTR-1 specific inhibitor montelukast inhibited osteoclastogenesis of BMMs. (A) Montelukast prevented the RANKL- and M-CSF-induced increase in the expression of NFATc1 and TRAP at the mRNA level in BMMs; (B) Montelukast prevented the RANKL- and M-CSF-induced increase in the expression of NFATc1 and TRAP at the protein level in BMMs (*, #, p < .01 vs. previous column group).
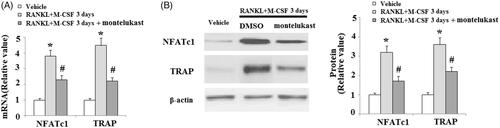
We then set out to define the molecular mechanisms through which montelukast prevented osteoclast formation by assessing M-CSF- and RANKL-induced signalling cascades on osteoclast differentiation. Our results indicate that the phosphorylation of EKR, JNK, p38 () and Akt () were increased in the vehicle group. However, montelukast significantly reduced the phosphorylation of EKR, JNK and p38 () but not Akt (). Additionally, we found that montelukast significantly reduced phosphorylation of IκBα (the upstream inhibitor of NF-κB) and p65 (a subunit of NF-κB) (). These results implicate that the suppressive impact of montelukast on osteoclast differentiation was attributed to the amelioration of RANKL-induced MAPK and NF-κB signalling cascades.
Figure 5. Effects of montelukast on the osteoclastogenesis-related signalling pathway. Mouse BMMs were incubated in serum-free medium for 5 h and pretreated with vehicle or montelukast (10 µM) for 2 h. Cells were treated with RANKL (50 ng/ml) and M-CSF (20 ng/ml) for 30 min. (A). Phosphorylation of MAPK family members EKR, JNK and p38; (B). Phosphorylation of AKT; (C). Phosphorylation of IκBα and p65 (*, #, p < .01 vs. previous column group).
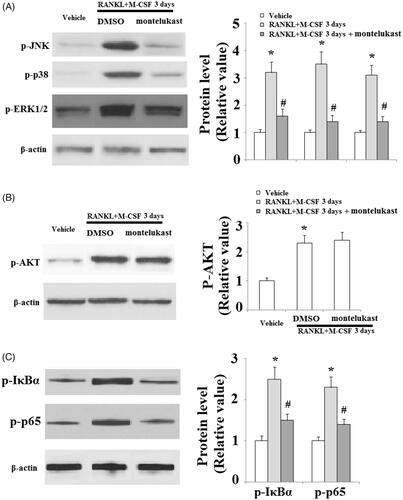
The generation of ROS and activation of Ca2+ signalling are essential for osteoclast differentiation. Here, we measured the effects of montelukast on osteoclast differentiation. The DCFH-DA assay demonstrated that incubation with RANKL and M-CSF significantly increased intracellular ROS levels, which were inhibited by montelukast (). Ca2+ signalling triggered by the phosphorylation of PLCγ2 is indispensable for osteoclastogenesis. Here, our findings indicate that the phosphorylation of PLCγ2 was elevated in response to RANKL and M-CSF treatment, which was clearly blocked by montelukast ().
Figure 6. Effects of montelukast on the generation of osteoclastogenesis-related reactive oxygen species (ROS) and calcium signalling activation. Mouse BMMs were incubated in serum-free medium for 5 h and pretreated with vehicle or montelukast (10 µM) for 2 h, followed by incubation with 50 ng/ml RANKL and 20 ng/ml M-CSF for 30 min. (A) Representative images for DCF fluorescence; (B) Phosphorylation of PLCγ2 (*, #, p < .01 vs. previous column group).
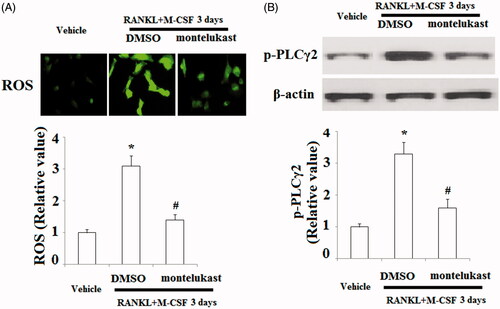
RANKL-induced intracellular signalling cascade is mediated by the association of RANK and TRAF6. The co-immunoprecipitation assay demonstrated that treatment with RANKL and M-CSF significantly increased the association of RANK and TRAF6, which were prevented by montelukast (.
Figure 7. Montelukast inhibited interaction between TRAF6 and RANK. Mouse BMMs were incubated in serum-free medium for 5 h and pretreated with vehicle or montelukast (10 µM) for 2 h, followed by incubation with 50 ng/ml RANKL and 20 ng/ml M-CSF for 6 h. Representative images for co-immunoprecipitation of TRAF6 and RANK (*, #, p < .01 vs. previous column group).
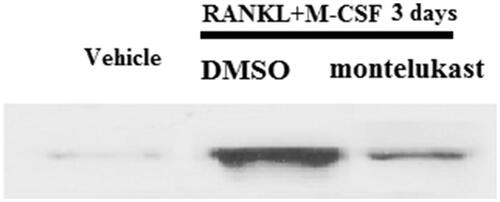
We then investigated the in vivo effects of montelukast on bone metabolism by using a 9-week-old ovariectomy mouse model. Ovariectomized mice were orally administered with 30 mg/kg montelukast or vehicle control (2 days each time for 4 weeks). As expected, our data indicate that administration of montelukast rescued the reduction of bone volume () and trabecular number () at the femur after ovariectomy. Additionally, we assessed whether montelukast could inhibit OVX-induced bone loss by suppressing osteoclast activity through performing morphometric analysis of the osteoclastic parametric quantity. The results in indicate that the ratio of eroded surface to bone surface was obviously elevated in OVX modelled mice, which was prevented by montelukast. Importantly, TRAP staining demonstrated that montelukast administration decreased TRAP activity of OVX mice ().
Figure 8. Montelukast ameliorated ovariectomy-induced bone loss by suppressing osteoclast activity. Ovariectomized mice were intraperitoneally injected with montelukast or vehicle control (2 days each time for 4 weeks). (A) Bone volume/tissue volume was analyzed; (B) Bone trabecular number was analyzed; (C) Eroded surface/bone surface was analyzed; (D) TRAP staining of the proximal tibiae of Sham, OVX and montelukast-treated OVX mice (*, #, p < .01 vs. previous column group).
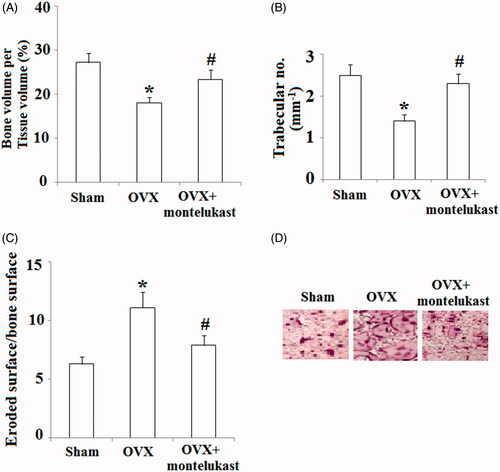
Discussion
Bone remodelling is regulated by the processes of both the bone formation and bone resorption [Citation21]. A balance in the ratio of osteoclasts to osteoblasts plays an important role in maintaining bone homeostasis [Citation22]. Abnormal differentiation of osteoclasts causes excessive bone resorption and subsequent bone destruction, which are associated with the pathological development of bone diseases, including osteoporosis, Paget’s disease and rheumatoid arthritis (RA) [Citation3]. The formation of functional osteoclasts is dependent on two factors, M-CSF and RANKL, both of which could be produced by osteoblast-lineage cells and osteocytes [Citation23]. M-CSF stimulates the proliferation of the osteoclast precursor cells by initiating the ERK1/2 intracellular signalling cascade [Citation5]. Additionally, RANKL is required for osteoclast differentiation and binds to its specific receptor RANK, causing recruitment of TRAF6 and activating multiple downstream targets such as MAPKs and AKT [Citation24]. Also, RANKL and M-CSF treatment resulted in the activation of NF-κB and NFATc1, which stimulated the expression of TRAP, an important osteoclast marker gene [Citation6]. A better understanding of the molecular mechanisms involved in osteoclast differentiation is beneficial for the development therapies for bone diseases. Blockage of accelerated bone-resorbing osteoclast differentiation has been considered as an important effective strategy for the treatment of osteoporosis. Here, we attempted to investigate the physiological roles of cysLTR-1 and its specific antagonist montelukast in RANKL- and M-CSF-induced osteoclast differentiation. Our findings include: (1) cysLTR-1 but not cysLTR-2 is expressed in BMMs; (2) Treatment with RANKL and M-CSF increased the expression of cysLTR-1; (3) Overexpression of cysLTR-1 promoted osteoclast differentiation by upregulating NFATc1 and TRAP. In contrast, blockage of cysLTR-1 by montelukast suppressed RANKL- and M-CSF-induced osteoclast differentiation; (4) Montelukast treatment prevented activation of the MAPK family members EKR, JNK and p38 as well as the NF-κB signalling pathway; (5) Montelukast suppressed the generation of ROS and activation of Ca2+ signalling; (6) Montelukast reduced the interaction of RANK and TRAF6; (7) Administration of montelukast rescued bone resorption in an ovariectomized mice model.
The MAPK and AKT signalling pathways are the key pathways for regulating bone resorption and contributing to osteoclast differentiation [Citation25]. Stimulation with RANKL induced the interaction of RANK and TRAF6, which then promoted the activation of MAPKs and AKT [Citation26]. Importantly, activation of cysLTR-1 by its ligands such as leukotriene D4 has been shown to induce the phosphorylation of MAPKs [Citation27]. In this study, we found that treatment with montelukast inhibited phosphorylation of ERK, JNK and p38 in the MAPK pathway by preventing the association of RANK and TRAF6, suggesting a novel molecular mechanism. Intracellular ROS acts as a second messenger regulating the receptor-mediated signalling cascades in diverse cells and tissues [Citation28,Citation29]. Increased production of ROS has been found in the pathological development process of osteoclastogenesis caused by RANKL and M-CSF in OC precursor cells [Citation30,Citation31]. Elevated production of ROS reinforces activation of downstream signalling molecules mediated by RANKL and M-CSF. In the current study, we found that montelukast ameliorated the generation of ROS, which is consistent with previous investigations demonstrating that administration of montelukast suppressed H2O2-induced ROS production [Citation32].
In summary, our results display that montelukast could act as a novel inhibitor of osteoclastogenesis by regulating multiple signalling pathways. It is suggested that montelukast might be used as a beneficial alternative preventive and therapeutic option for osteoclast-related disorders.
Disclosure statement
No potential conflict of interest was reported by the authors.
References
- Xiong J, O'Brien CA. Osteocyte RANKL: new insights into the control of bone remodeling. J Bone Miner Res. 2012;27:499–505.
- Karsenty G, Wagner EF. Reaching a genetic and molecular understanding of skeletal development. Dev Cell. 2002;2:389–406.
- Walsh MC, Kim N, Kadono Y, et al. Osteoimmunology: interplay between the immune system and bone metabolism. Annu Rev Immunol. 2006;24:33–63.
- Kim WS, Kim H, Jeong EM, et al. Transglutaminase 2 regulates osteoclast differentiation via a Blimp1-dependent pathway. Sci Rep. 2017;7:10626.
- Gingery A, Bradley E, Shaw A, et al. Phosphatidylinositol 3-kinase coordinately activates the MEK/ERK and AKT/NFkappaB pathways to maintain osteoclast survival. J Cell Biochem. 2003;89:165–179.
- Takayanagi H. Osteoimmunology: shared mechanisms and crosstalk between the immune and bone systems. Nat Rev Immunol. 2007;7:292–304.
- Kim YG, Kang JH, Kim HJ, et al. Bortezomib inhibits osteoclastogenesis and porphyromonas gingivalis lipopolysaccharide-induced alveolar bone resorption. J Dent Res. 2015;94:1243–1250.
- Ihn HJ, Kim JA, Cho HS, et al. Diphlorethohydroxycarmalol from Ishige Okamurae suppresses osteoclast differentiation by downregulating the NF-κB signaling pathway. IJMS. 2017;18:2635.
- Austen KF. The cysteinyl leukotrienes: where do they come from? What are they? Where are they going? Nat Immunol. 2008;9:113–115.
- Liu M, Yokomizo T. The role of leukotrienes in allergic diseases. Allergol Int. 2015;64:17–26.
- Kim DC, Hsu FI, Barrett NA, et al. Cysteinyl leukotrienes regulate Th2 cell-dependent pulmonary inflammation. J Immunol. 2006;176:4440–4448.
- Busse W, Kraft M. Cysteinyl leukotrienes in allergic inflammation: strategic target for therapy. Chest 2005;127:1312–1326.
- Currie GP, Srivastava P, Dempsey OJ, et al. Therapeutic modulation of allergic airways disease with leukotriene receptor antagonists. Q J Med. 2005;98:171–182.
- Henderson WR, Jr, Chiang GK, Tien YT, et al. Reversal of allergen-induced airway remodeling by CysLT1 receptor blockade. Am J Respir Crit Care Med. 2006;173:718–728.
- Peters-Golden M, Henderson WR. Leukotrienes. N Engl J Med. 2007;357:1841–1854.
- Zhao R, Shi WZ, Zhang YM, et al. Montelukast, a cysteinyl leukotriene receptor-1 antagonist, attenuates chronic brain injury after focal cerebral ischaemia in mice and rats. J Pharm Pharmacol. 2011;63:550–557.
- Chang E-J, Ha J, Oerlemans F, et al. Brain-type creatine kinase has a crucial role in osteoclast-mediated bone resorption. Nat Med. 2008;14:966–972.
- Plotkin LI, Manolagas SC, Bellido T. Dissociation of the pro-apoptotic effects of bisphosphonates on osteoclasts from their anti-apoptotic effects on osteoblasts/osteocytes with novel analogs. Bone 2006;39:443–452.
- Hayashi M, Nakashima T, Taniguchi M, et al. Osteoprotection by semaphorin 3A. Nature 2012;485:69–76.
- Hayashi M, Nakashima T, Kodama T, et al. Ly49Q, an ITIM-bearing NK receptor, positively regulates osteoclast diferentiation. Biochem Biophys Res Commun. 2010;393:432–438.
- Chen Q, Hou T, Luo F, et al. Involvement of toll-like receptor 2 and pro-apoptotic signaling pathways in bone remodeling in osteomyelitis. Cell Physiol Biochem. 2014;34:1890–1900.
- Boyle WJ, Simonet WS, Lacey DL. Osteoclast differentiation and activation. Nature 2003;423:337–3342.
- Park JH, Lee NK, Lee SY. Current understanding of RANK signaling in osteoclast differentiation and maturation. Mol Cells. 2017;40:706–713.
- Lee ZH, Kim HH. Signal transduction by receptor activator of nuclear factor kappa B in osteoclasts. Biochem Biophys Res Commun. 2003;305:211–214.
- Walsh MC, Choi Y. Biology of the RANKL-RANK-OPG system in immunity, bone, and beyond. Front Immunol. 2014;5:511.
- Kadono Y, Okada F, Perchonock C, et al. Strength of TRAF6 signalling determines osteoclastogenesis. EMBO Rep. 2005;6:171–176.
- Boehmler AM, Drost A, Jaggy L, et al. The CysLT1 ligand leukotriene D4 supports alpha4beta1- and alpha5beta1-mediated adhesion and proliferation of CD34+ hematopoietic progenitor cells. J Immunol. 2009;182:6789–6798.
- Kim MS1, Yang YM, Son A, et al. RANKL-mediated reactive oxygen species pathway that induces long lasting Ca2+ oscillations essential for osteoclastogenesis. J Biol Chem. 2010;285:6913–6921.
- Rezaei-Sadabady R, Eidi A, Zarghami N, et al. Intracellular ROS protection efficiency and free radical-scavenging activity of quercetin and quercetin-encapsulated liposomes. Artif Cells Nanomed Biotechnol. 2016;44:128–134.
- Lee NK, Choi YG, Baik JY, et al. A crucial role for reactive oxygen species in RANKL-induced osteoclast differentiation. Blood 2005;106:852–859.
- Guo X, Wei S, Lu M, et al. RNA-Seq investigation and in vivo study the effect of strontium ranelate on ovariectomized rat via the involvement of ROCK1. Artif Cells Nanomed Biotechnol. 2018;30:1–13.
- Tsai MK, Lin YC, Huang MY, et al. The effects of asthma medications on reactive oxygen species production in human monocytes. J Asthma 2017;11:1–9.