Abstract
Thymosin β4 (Tβ4) is a multifunctional N-acetylated peptide with distinct activities important at various stages. Due to its potential multiple therapeutic uses in many fields, there is an increasing need of Tβ4 at lower costs than with the use of chemical synthesis. In this research, we developed a method to produce rhTβ4 with N-acetylation in E. coli. Firstly, the E. coli strain whose chromosome being integrated by the specific N-terminal acetyltransferase ssArd1 was constructed. Secondly, the rhTβ4-Intein was constructed, in which rhTβ4 was fused to the N-terminus of the smallest mini-intein Spl DnaX. The rhTβ4 could be fully acetylated when the rhTβ4-Intein was expressed in the engineering strain. After purification, the rhTβ4-Intein fusion protein was induced with dithiothreitol (DTT) to release rhTβ4 through intein-mediated N-terminal cleavage. Under the optimal conditions, the N-terminal serine residue was shown to be 100% acetylated and the yield of N-acetylated rhTβ4 can reach 200 mg per litre. The N-acetylated rhTβ4 could be stable at 2–8 °C for 24 months in PBS buffer without protein degradation and concentration change. The N-acetylated rhTβ4 also showed the activity of binding with actins from different sources and excellent therapeutic effect on the rats with moderate to severe dry eye disease.
Introduction
Thymosin β4 (Tβ4), an N-acetylated 43-amino acid peptide with a molecular weight of 4960.5 Da and an isoelectric point of 4.6 [Citation1], is widely found in the tissues, organs and cells of mammals and other vertebrates [Citation2]. It is highly conserved in mammals and highly expressed in platelets, leukocytes and macrophages, but not in red cells [Citation3]. Tβ4 has a lot of biological activities, such as reducing inflammation [Citation4–9], preventing infection in wounds [Citation10], blocking apoptosis [Citation11–13] and scar formation [Citation5,Citation11,Citation14], promoting cell migration and angiogenesis [Citation15–17], accelerating collagen deposition and upregulating the production of laminin-5 [Citation16,Citation18–23]. Nonclinical and clinical studies to date have shown that Tβ4 is safe, well-tolerated [Citation24,Citation25] and has important application value in wound repair, tissue protection and regeneration in the skin, eye, heart and central nervous system [Citation26–28]. Tβ4 developed by RegeneRX (http://www.regenerx.com) is currently in the course of advanced clinical trials and the indications include dry eye syndrome, neurotrophic keratopathy, cardiac damage post-acute myocardial infarction, epidermolysis bullosa and venous stasis ulcers. However, the Tβ4 produced by RegeneRX is a chemical synthetic peptide, which requires a large number of toxic reagents, especially acetylated reagents. Another disadvantage is that the production cost is high and the scale of production is difficult to enlarge.
Escherichia coli (E. coli) is one of the widely used hosts in the field of genetic recombination. The most outstanding advantages of using this expression system include short production cycle, low cost, easy to scale up and high level of expression. However, there are two main obstacles of the biosynthesis of Tβ4 in E. coli: the bacterial expression of small peptides is difficult in general and this protein requires N-acetylation. Although there have been reports about the recombinant expression of human Tβ4 (rhTβ4), the expressions are relatively low (∼1 mg/L) and the sequences are not completely consistent with natural Tβ4 [Citation29–31]. Some rhTβ4 are expressed by fusion with the label protein, and the extra amino acids are produced after cutting, some rhTβ4 are expressed in concatemer forms, and all of them are lack of N-acetylation. The non-natural sequence may affect the activity of Tβ4, and the lack of N-acetylation modification may seriously affect the stability of Tβ4. To this day, there is no report of N-acetylated rhTβ4 expressed in bacterial cells.
In this article, we solved two major problems in the biosynthesis of rhTβ4: N-acetylation and high expression. A fusion protein, rhTβ4-Intein, was constructed, in which rhTβ4 was fused to the N-terminus of the smallest mini-intein, Spl DnaX (from Spirulina platensis). The rhTβ4 could be fully acetylated when the rhTβ4-Intein fusion protein was expressed with the N-terminal acetyltransferase ssArd1 in E. coli. After purification and cutting by the dithiothreitol (DTT), rhTβ4 was produced with the N-terminal serine residue 100% acetylated. The yield of the N-acetylated rhTβ4 can reach more than 200 mg per litre culture fluid and about 2 grams of rhTβ4 with the purity ≥95% could be obtained after 30 L volume fermentation. The N-acetylated rhTβ4 prepared by this method showed excellent stability, activity of binding with actins from different sources and therapeutic effect on the rats with moderate to severe dry eye.
Materials and methods
Bacterial strains, plasmids and reagents
E. coli DH5α was used for all plasmid propagation. E. coli BL21 (DE3) was used for expressing proteins. Plasmids pET22b (+) was used as expression vectors. Plasmids pBR322, all the restriction enzymes, T4 DNA ligase, Pyrobest™ DNA polymerase, DNA molecular weight marker were from TaKaRa Biotechnology (Dalian, China). Protein low molecular weight marker was purchased from GE Healthcare. Synthetic Tβ4 (sTβ4) was the product of ProSpec (Ness Ziona, Israel). Actin proteins from human platelet, rabbit skeletal muscle and bovine cardiac muscle were products of Cytoskeleton (Denver, CO). N-(3-Dimethyla minopropyl)-N′-ethylcarbodiimide (EDC), N-hydroxysulfosuccinimide (Sulfo-NHS) and scopolamine hydrobromate were products of Merck (Darmstadt, Germany). Other chemicals used in this study were of analytical or higher grade. The genes synthesis and DNA sequencing were all performed by Shanghai Sangon Biological Engineering Technology and Services (Sangon; Shanghai, China).
Animals and control drugs
Female SPF Wistar rats weighting 1 6 0 ∼ 180 g were purchased from Beijing Laboratory Animal Center, Beijing, China. All animal experiments used in this study were performed according to the protocols approved by the Institutional Animal Care and Use Committee of Beijing Institution of Biotechnology (Identification code for rat: IACUC of AMMS-08-2017-010; Date of approval: 01 June 2017). Carboxymethylcellulose Sodium Eye Drops were products of Allergan company in the United States and Tacrolimus Eye Drops were products of Senju Pharmaceutical Co., Ltd.
Construction of NTA expression strain E. coli BL21 (DE3) lpxM: Kan-ssArd1
ssArd1 is a N-terminal acetyltransferases (NTA) from the archaea which can catalyse the N-terminal serine acetylation of rhTβ4. The nucleotide sequence () that encodes ssArd1 [Citation32] was designed with the E. coli codon bias and synthesized to ensure high expression in E. coli. The recombinant expression cassette expressing ssArd1 was integrated into the host bacterial chromosome by a one-step strategy as shown in . Briefly, the helper plasmid pKDS was formerly constructed in our laboratory, containing Red recombinant enzyme gene and I-SceI endonuclease gene under the control of the arabinose promoter. Vector pBR322 was used as the template to construct the integrated targeting plasmid pBRSS-LPLR-Kan-Ard1. Both sides of the targeting fragment containing kanamycin resistant gene and ssArd1 had restriction sites of I-SceI, and homologous arms were assembled upstream and downstream about 1000 bp of the target gene. According to our reported method [Citation33], the targeting plasmid and the helper plasmid were co-transformed into E. coli BL21 (DE3) and the Red recombinase and I-SceI then co-expressed by being induced by 0.2% L-Arabia sugar. I-SceI could cut off the target DNA fragments from the plasmid pBRSS-LPLR-Kan-Ard1. The ssArd1 gene expression cassette was then integrated into the chromosomes of E. coli under the action of Red recombinant enzyme. The clones which could grow on kanamycin resistant plate, but not on tetracycline and chloramphenicol resistant plate were screened and confirmed by PCR as positive strain E. coli BL21 (DE3) lpxM: Kan-ssArd1.
Construction of plasmid pET-rhTβ4-intein to express rhTβ4-intein
The construction of plasmid pET-Intein which expressions the gene of the intein-Spl DnaX has been described before [Citation33]. Briefly, the gene that encodes the Spl DnaX intein (136 amino acid residues; from Spirulina platensis) contained EcoRI and XhoI restriction enzyme sites was designed with the E. coli codon bias and cloned into the pET22b (+) vector. The C-terminal amino acid was mutated from Asn to Ala, which caused it to lose its self-splicing activity but did not affect the N-teminal cutting activity. The third amino acid at the N-terminus was also mutated from Thr to Ser to introduce an AflII site.
The rhTβ4 gene contained the NdeI 和AflII restriction enzyme sites was designed and synthesized. The gene product and vector pET-Intein were both digested with NdeI and AflII. The fragments were purified after 1% agarose gel electrophoresis, and then ligated using T4 DNA ligase to yield plasmid pET- rhTβ4-Intein. Clones were selected by ampicillin screening, and then, the positive clones were checked by DNA sequencing to ensure they contained the correct rhTβ4-Intein sequence as .
Protein expression and purification of rhTβ4
E. coli strain BL21 (DE3) lpxM: Kan-ssArd1was transformed with the plasmid pET-rhTβ4-Intein to co-express ssArd1 and rhTβ4-Intein. The positive clones were selected using an ampicillin and kanamycin resistant agar plate and cultivated in the FMG medium (K2HPO4•3H2O, 7 g/L; NaH2PO4•2H2O, 3 g/L; NaCl, 2.5 g/L; yeast extract, 12 g/L; tryptone, 15 g/L; MgSO4•7H2O, 0.5 g/L; glucose, 2 g/L) containing 100 μg/mL ampicillin and 50 μg/mL kanamycin at 30 °C for 12 h. For a 30 L fermentation, the culture mixture was then 1:30 (v/v) transferred to the auto-inducible medium FML (K2HPO4•3H2O, 7 g/L; NaH2PO4•2H2O, 3 g/L; NaCl, 2.5 g/L; yeast extract, 12 g/L; tryptone, 15 g/L; MgSO4•7H2O, 0.5 g/L; glucose, 2 g/L; lactose, 0.722 g/L) shaking at 30 °C 220 rpm/min for 12 h. Cell pellets were harvested by centrifugation (10,000 × g, 20 min, 4 °C) and resuspended in buffer A (20 mM sodium phosphate, 0.5 M NaCl, 10 mM imidazole, pH 7.4) and lysed by a homogenizer at 800 bar twice. Soluble protein was separated from cell debris by centrifugation at 15,000 × g, 4 °C for 30 min, filtered through a 0.45-μm membrane and loaded onto a 400 mL HisTrap Fast Flow column (GE Healthcare, Boston, USA) pre-equilibrated with buffer A. Bound proteins were eluted with buffer B (20 mM sodium phosphate, 0.5 M NaCl, 400 mM imidazole, pH 7.4). The peak fractions, including rhTβ4-Intein were then incubated with 200 mM d, l-dithiothreitol (DTT) at 42 °C for 24 h. The supernatant contained rhTβ4 was collected and loaded onto a Phenyl Sepharose 6 Fast Flow column (high sub) (GE Healthcare, Aurora, OH) that had been pre-equilibrated with buffer C (50 mM sodium phosphate with 1 M ammonium sulfate, pH 7.0). The rhTβ4 target protein existed in the through fractions. The 3 K and 30 K ultrafiltration membranes (Sartorius, Gottingen, Germany) were subsequently used to replace the buffer solution with PBS (20 mM sodium phosphate, 0.5 M NaCl) and remove the endotoxin. All proteins were detected by 17% SDS-PAGE followed by staining with Coomassie Brilliant Blue.
Characterization of rhTβ4
The accurate molecular mass, whole sequence and N-acetylation of rhTβ4 was determined using Thermo Scientific™ Orbitrap Fusion™ Lumos™ Tribrid™ Mass Spectrometer. The results were then searched and analysed with Mascot software from Matrix Science.
The purity of rhTβ4 were analysed by SEC-HPLC using a TSKgel G2000 SWXL 5-μm column (TOSOH, Japan) with a mobile phase of 20 mM sodium phosphate, 0.15 M NaCl and a 0.8 mL/min flow rate.
For detecting the stability of purified rhTβ4, the protein was kept at 4 °C, −20 °C, −80 °C in PBS buffer, respectively. The stability of rhTβ4 was measured by 12% SDS-PAGE and the protein concentration was measured by a Lowry method (Pierce™ Modified Lowry Protein Assay kits, operated according to the instructions) after being kept for different time.
The binding activity of rhTβ4 to actins from different sources was also determined. 0 ∼ 20 μM of rhTβ4 or sTβ4 was mixed with 5 mg/mL of actin proteins from human platelet or bovine cardiac muscle in general actin buffer (200 μM CaCl2, 200 μM ATP, 200 μM DTT, 5 mM Tris, pH8.0) at 25 °C for 20 min, respectively. EDC and Sulfo-NHS with the final concentration of 5 mM were then added to the mixture and continue reacting at 25 °C for 45 min. 2 × loading buffer was added to terminate the reaction and the percentage of the binding were analysed by 10% SDS-PAGE and ImageJ software.
Moderate to severe dry eye model in rats and animal groups
Thirty-five female Wistar rats of six-weeks old were randomly divided into seven groups with different treatment as following: (a) Normal group without any treatment; (b) Dry eyes group without any treatment; (c) Dry eyes group treated with PBS; (d) Dry eyes group treated with Carboxymethylcellulose Sodium Eye Drops; (e) Dry eyes group treated with Tacrolimus Eye Drops; (f) Dry eyes group treated with 0.05% rhTβ4; (g) Dry eyes group treated with 0.1% rhTβ4. Each group has five rats (10 eyes). The b ∼ g groups were injected subcutaneously with 3 mg/mL scopolamine hydrobromide solution, four times per day with a volume of 0.5 mL each time. Each group of drugs was treated three times per day with a volume of 25 μL each time for 28 days. Both eyes from each rat were evaluated.
Evaluation of tear volume using Schirmer I test (SIt)
The phenol red threads were used to test the tear secretion of the rat eyes at the treatment days 0, 7, 14, 21 and 28. The thread ends were placed gently at the 1/3 of the lower eyelid conjunctival sac with tweezers. The cotton threads were then taken out after staying for 30 s. The length of the cotton thread turned from yellow to red was measured.
Measurement of tear film break up time (but)
The break up time of the tear film after treatment was detected. One microlitre of fluorescein sodium with the concentration of 10g/L was dripped to the conjunctival sac, and the eyelids were then closed. The break up time was recorded from the third blink to the appearance of the first spots in cornea using a slit-lamp microscope equipped with a cobalt blue light.
Corneal fluorescein staining
Fluorescein sodium (1 μL of 10g/L solution) was instilled onto the central cornea of each eye using a micropipette. Ninety seconds after the application, staining was evaluated under magnification and digital photographs were taken to document the response. The severity of fluorescein staining was graded according to a modified 0–4 grading scale (Ora Scale, modified from NEI), similar to that used in clinical dry eye studies, where the average cornea was divided into four quadrants and graded respectively. All the scores were summed to the final score. Standard for evaluation: 0 point: no staining; 1 point: punctate staining is less than 30; 2 points: punctate staining is more than 30 but no diffusion; 3 points: diffuse colour serious but has not yet formed plaque; 4: patchy staining.
Haematoxylin-eosin (HE) staining and periodic acid schiff (PAS) staining
After evaluations on day 28, the animals were terminated and the eyeballs were separated for the histopathological examination including HE staining and PAS staining. The tissue sections were fixed in 4% paraform-aldehyde and embedded in paraffin. The HE staining was performed as described elsewhere [Citation33] to observe the changes in corneal histopathology. The sections were also stained with Periodic acid-Schiff reagent. Sections from each group were examined and photographed with a microscope. Goblet cells in the conjunctiva were counted in three sections from each eye.
Statistical analysis
All eyes of each group were averaged within a group to obtain an average ± SD for each treatment group. A Student’s t test was used to determine the significance of the difference between two independent data, and two-way ANOVA was used to determine the significance between the two groups. p < .05 was deemed as a statistical difference.
Results
Expression and purification of N-acetylated rhTβ4
A one step recombinant strategy was used to integrate the acetyltransferase gene from Archaea to the chromosome of E. coli and the NTA ssArd1 expression strain BL21 (DE3) lpxM: Kan-ssArd1 was constructed. The expression plasmid was constructed by fusion of the target peptide rhTβ4 to the N-terminal of Spl DnaX intein while the His-tag fused to the C-terminal of the intein. When transferred into the BL21 (DE3) lpxM: Kan-ssArd1, the positive clone was selected using an ampicillin and kanamycin resistant agar plate. FMG medium was used to amplify the culture and FML medium contained lactose was used to induce the expression of the rhTβ4-Intein fusion protein. The expression of the fusion protein was controlled by the T7 promoter and induced by lactose resulting in the N-acetylation. The fusion protein was purified by a HisTrap Fast Flow column and the target N-acetylated rhTβ4 was released after the self-cutting effect of intein by DTT. The purified rhTβ4 was obtained after hydrophobic chromatography, 3 K and 30 K ultrafiltration (. Under the 30 L fermentation scale, the soluble fusion protein accounted for about 60% of the total protein of the homogenate supernatant with an expression level of about 200 mg/L. About 2 grams of purified N-acetylated rhTβ4 could be acquired after 30 litres of fermentation.
Figure 4. Expression and purification of N-acetylated rhTβ4. (A) SDS-PAGE analysis of the expression of rhTβ4-Intein fusion protein in three batches of 30L fermentation broth in E. coli. (B) SDS-PAGE analysis of the purified rhTβ4 from three batches fermentation. Lane M: low molecular protein Marker; Lane A1–3: ultrasonic supernatant s of three batches; Lane B1–3: purified rhTβ4 of three batches.
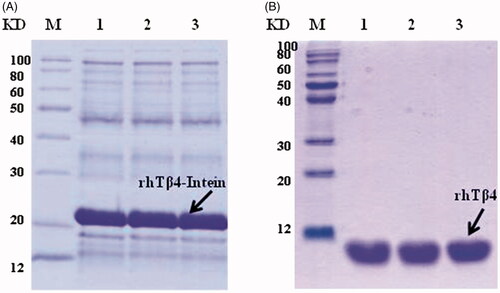
Characterization of N-acetylated rhTβ4
The accurate molecular mass, whole sequence and N-acetylation of rhTβ4 were detected by Mass Spectrometer. The results were searched and analysed with Mascot software from Matrix Science as shown in . The molecular mass of the purified N-acetylated rhTβ4 was 4960.465 Da, which fully chimed with the theoretical value 4960.5 Da. The whole sequence was consistent with the natural rhTβ4 sequence and the coverage was 100%. The Mascot search result also showed that the N-terminal of the rhTβ4 was fully acetylated.
Figure 5. The accurate molecular mass, whole sequence and N-acetylation of rhTβ4 detected by Mass Spectrometer. (A) High resolution precise molecular weight (after deconvolution); (B) Mascot search result of the whole sequence and the coverage was 100%; (C) Mascot search result of the 1–22 Aa of rhTβ4 showed the N-terminal was fully acetylated.
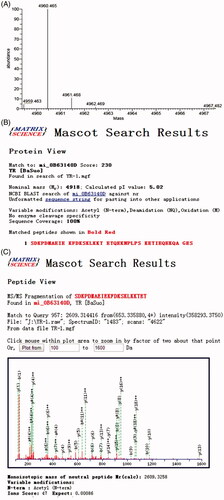
The purity of rhTβ4 purified after four batches fermentation were detected by SEC-HPLC (. As the result shown, the peak time of rhTβ4 was exactly the same as that of sTβ4, and the purity of each batch of rhβ4 was above 98%, which can totally meet the requirement of drug preparation.
The stability of rhTβ4 were analysed by SDS-PAGE after being kept at 2 ∼ 8 °C, –20 °C and –80 °C for different time and no protein degradations or polymers were found after 24 months of placement even at 2 ∼ 8 °C (. The protein concentrations were also constantly monitored by the Lowry method and no changes were found during the test period.
Figure 7. Stability analysis of the of rhTβ4 by SDS-PAGE. (A) rhTβ4 kept at different temperature on month 0; (B): rhTβ4 kept at different temperature on month 12;: rhTβ4 kept at different temperature on month 24. Lane M, low molecule protein marker; Lane 1, 2 ∼ 8 °C; Lane 2, –20 °C; Lane 3, –80 °C.
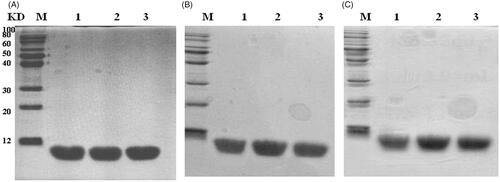
Tβ4 can binding to actins and form a complex with stable peptide in the presence of EDC and Sulfo-NHS. This binding activities of rhTβ4 with actin from human platelet and bovine cardiac muscle were analysed and the percent bindings were compared with sTβ4. After detected by 10% SDS-PAGE, rhTβ4 and actin could form a complex with a molecular weight of 47KDa, which could be separated from the actin of 42KDa. As the concentration of Tβ4 increased, the proportions of the complex were also increased. The rhTβ4 showed higher binding activities than sTβ4 with actins from human platelet or bovine cardiac muscle (.
rhTβ4 significantly reduces the signs of dryness in a rat model of moderate to severe dry eye
The rat model of moderate to severe dry eye disease was established by injecting subcutaneously with 3 mg/mL scopolamine hydrobromide solution and the efficacy of various doses rhTβ4 treatment was evaluated. From the 14th day after treatment, the tear secretion volume and tear film break up time in the treatment groups including PBS vehicle, Carboxymethylcellulose Sodium Eye Drops, Tacrolimus Eye Drops, 0.05% rhTβ4 and 0.1% rhTβ4 were significantly higher than those in the untreated group (p < .05). On the 14th day, there was no significant difference between the treatment groups. On days 21 and 28, the tear volume and tear film break up time in the 0.1% rhTβ4 treatment group were significantly higher than those in the PBS vehicle group (p < .05) (.
Corneal fluorescein staining score can be used to assess the extent of damage. As the result shown (), the scores of 0.05% and 0.1% rhTβ4 treatment group were significantly lower than that in the untreated group from the days 14. On days 21, the scores of 0.05% and 0.1% rhTβ4 treatment group were both significantly lower than that of PBS vehicle group. On days 28, the scores of 0.1% rhTβ4 group was not only significantly lower than that in the PBS vehicle group, but also lower than that in Carboxymethylcellulose Sodium Eye Drops and Tacrolimus Eye Drops groups (p < .05). The digital photographs taken after the corneal fluorescein staining showed that the normal corneal surface was smooth and no obvious pigmentation, while severe corneal staining including thick spot and patchy diffuse could be observed in the untreated group and the PBS treatment group. The corneal surfaces were visible in a fewer scattered punctate staining in the treatment groups compared with the untreated group. 0.1% rhTβ4 group showed the most obvious effect, which suggested that it could effectively promote the repair of corneal injury ().
Figure 10. Corneal fluorescein staining (A) and scores (B). a: Normal group without any treatment; b: Dry eyes group without any treatment; c: Dry eyes group treated with PBS; d: Dry eyes group treated with Carboxymethylcellulose Sodium Eye Drops; e: Dry eyes group treated with Tacrolimus Eye Drops; f: Dry eyes group treated with 0.05% rhTβ4; g: Dry eyes group treated with 0.1% rhTβ4.
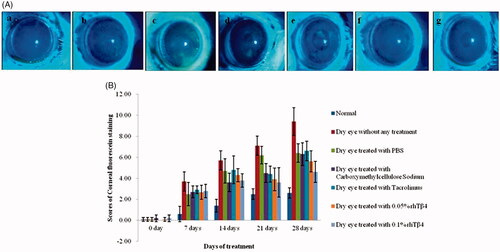
The results of the corneal histopathology detected by HE and PAS staining also showed that the rhTβ4 treatment groups could significantly improve the corneal oedema and the corneal epitheliums were smoother than the other groups (). The corneal histopathology in the rhTβ4 treatment groups also showed a weakening trend of pathological changes, regular arrangement of cells in all layers of epithelial cells and decreasing of the disordered arrangement of fibroblasts in the stromal layer. The PAS staining result showed that the density of goblet cells in the conjunctiva increased and the arrangement of the goblet cells were more regular in the rhTβ4 treatment groups.
Figure 11. Haematoxylin-eosin (HE) staining (A) and periodic acid schiff (PAS) (B) staining of corneal tissue sections. a: Normal group without any treatment; b: Dry eyes group without any treatment; c: Dry eyes group treated with PBS; d: Dry eyes group treated with Carboxymethylcellulose Sodium Eye Drops; e: Dry eyes group treated with Tacrolimus Eye Drops; f: Dry eyes group treated with 0.05% rhTβ4; g: Dry eyes group treated with 0.1% rhTβ4.
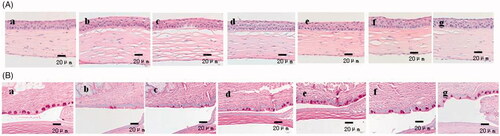
Discussion
Acetylation is a ubiquitous protein modification, which has an important influence on the protein activity and stability. In some cases, the lack of acetylation can lead to the decrease of protein thermal stability, complex assembly efficiency and the change of dynamic parameters [Citation34]. For polypeptide drugs, the acetylation modification can improve the half-life of peptides in vivo [Citation35]. Although in vitro studies indicated that non-acetylated Tβ4 also had partial biological activity, the acetylation modification could greatly increase the stability of Tβ4 in vivo. The rhTβ4 produced by Prospec (catalogue number: HOR-003), a recombinant expression product in E. coli without N-acetylation, could be stored at 4 °C only between 2 ∼ 7 days upon reconstitution, while the recombinant N-acetylated rhTβ4 produced in this study could be stable at 2–8 °C for 24 months in PBS buffer without protein degradation and concentration change. Accordingly, N-acetylation has an important influence on the stability of rhTβ4, which is the key point for rhTβ4 being developed into a drug.
There are a variety of intracellular NATs, which are able to catalyse different types of amino acid sequence of the polypeptide. According to the simulation results of the crystal structure and the computer, the NATs from prokaryotic genomes, only can presumably catalyse a protein substrate. NATs from eukaryotic sources can catalyse a variety of substrates, but the single subunit has no catalytic activity. According to the report, ssArd1, the N-acetylation enzyme from halophilic archaea, which is closer to eukaryotes from the evolutionary perspective, has many substrates and it is supposed to catalyse the N-acetylation of rhTβ4 [Citation32]. Our results showed that the fully N-acetylation modification of rhTβ4 could be obtained when co-expressed with the ssArd1 gene in E. coli. This method can also be applied to acquire recombinant expression of other acetylated proteins.
The chemical synthesis of peptides has many drawbacks such as low purity, high production cost and the environment pollution, which could not fully meet the needs of commercial purposes. The biosynthesis of recombinant polypeptide with unlimited scale, low production cost and controllable quality is the preferred preparation method of polypeptide drugs. In this study, N-acetylated rhTβ4 was produced in E. coli, and its expression level reached at about 200 mg/L. About 2 grams of rhTβ4 with the purity of greater than 98% could be ultimately obtained after 30 L fermentation. The rhTβ4 could bind with actins from different sources and the binding percentage at the same concentration was higher than that of sTβ4. The N-acetylated rhTβ4 also showed excellent biological activity in a moderate to severe dry eye model of rats. The rhTβ4 with the concentration of 0.1% could significantly increase the rat tear secretion volume, prolong the tear film break-up time and effectively repair the corneal injury. Accordingly, our study provided a method for the preparation of N-acetylated rhTβ4, which had a broad application prospect in the commercial drug development.
Disclosure statement
No potential conflict of interest was reported by the authors.
Reference
- Hannappel E. Thymosin beta4 and its posttranslational modifications. Ann N Y Acad Sci. 2010;1194:27–35.
- Hu SK, Low TL, Goldstein AL. Modulation of terminal deoxynucleotidyl transferase activity by thymosin. Mol Cell Biochem. 1981;41:49–58.
- Safer D, Golla R, Nachmias VT. Isolation of a 5-kilodalton actin-sequestering peptide from human blood platelets. Proc Natl Acad Sci USA. 1990;87:2536–2540.
- Badamchian M, Fagarasan MO, Danner RL. Thymosin beta(4) reduces lethality and down-regulates inflammatory mediators in endotoxin-induced septic shock. Int Immunopharmacol. 2003;3:1225–1233.
- Cavasin MA. Therapeutic potential of thymosin-beta4 and its derivative N-acetyl-seryl-aspartyl-lysyl-proline (Ac-SDKP) in cardiac healing after infarction. Am J Cardiovasc Drugs. 2006;6:305–311.
- Sosne G, Szliter EA, Barrett R, et al. Thymosin beta 4 promotes corneal wound healing and decreases inflammation in vivo following alkali injury. Exp Eye Res. 2002;74:293–299.
- Sosne G, Qiu P, Christopherson PL, et al. Thymosin beta 4 suppression of corneal NFkappaB: a potential anti-inflammatory pathway. Exp Eye Res. 2007;84:663–669.
- Sosne G, Christopherson PL, Barrett RP, et al. Thymosin-beta4 modulates corneal matrix metalloproteinase levels and polymorphonuclear cell infiltration after alkali injury. Invest Ophthalmol Vis Sci. 2005;46:2388–2395.
- Young JD, Lawrence AJ, Mac Lean AG, et al. Thymosin beta 4 sulfoxide is an anti-inflammatory agent generated by monocytes in the presence of glucocorticoids. Nat Med. 1999;5:1424–1427.
- Huang LC, Jean D, Proske RJ, et al. Ocular surface expression and in vitro activity of antimicrobial peptides. Curr Eye Res. 2007;32:595–609.
- Bock-Marquette I, Saxena A, White MD, et al. Thymosin beta4 activates integrin-linked kinase and promotes cardiac cell migration, survival and cardiac repair. Nature. 2004;432:466–472.
- Ho JH, Tseng KC, Ma WH, et al. Thymosin beta-4 upregulates anti-oxidative enzymes and protects human cornea epithelial cells against oxidative damage. Br J Ophthalmol. 2008;92:992–997.
- Ho JH, Chuang CH, Ho CY, et al. Internalization is essential for the antiapoptotic effects of exogenous thymosin beta-4 on human corneal epithelial cells. Invest Ophthalmol Vis Sci. 2007;48:27–33.
- Ehrlich HP, Sprague WH. Thymosin beta4 enhances repair by organizing connective tissue and preventing the appearance of myofibroblasts. Ann N Y Acad Sci. 2010;1194:118–124.
- Smart N, Risebro CA, Melville AAD, et al. Thymosin beta4 induces adult epicardial progenitor mobilization and neovascularization. Nature. 2007;445:177–182.
- Malinda KM, Goldstein AL, Kleinman HK. Thymosin beta 4 stimulates directional migration of human umbilical vein endothelial cells. FASEB J. 1997;11:474–481.
- Grant DS, Kinsella JL, Kibbey MC, et al. Matrigel induces thymosin beta4 gene in differentiating endothelial cells. J Cell Sci. 1995;108:3685–3694.
- Sosne G, Siddiqi A, Kurpakus-Wheater M. Thymosin-beta4 inhibits corneal epithelial cell apoptosis after ethanol exposure in vitro. Invest Ophthalmol Vis Sci. 2004;45:1095–1100.
- Qiu P, Kurpakus-Wheater M, Sosne G. Matrix metalloproteinase activity is necessary for thymosin beta 4 promotion of epithelial cell migration. J Cell Physiol. 2007;212:165–173.
- Roy P, Rajfur Z, Jones D, et al. Local photorelease of caged thymosin beta4 in locomoting keratocytes causes cell turning. J Cell Biol. 2001;153:1035–1048.
- Sosne G, Hafeez S, Greenberry AL II, et al. Thymosin beta4 promotes human conjunctival epithelial cell migration. Curr Eye Res. 2002;24:268–273.
- Sosne G, Xu L, Prach L, et al. Thymosin beta 4 stimulates laminin-5 production independent of TGF-beta. Exp Cell Res. 2004;293:175–183.
- Sosne G, Qiu P, Goldstein AL, et al. Biological activities of thymosin beta4 defined by active sites in short peptide sequences. FASEB J. 2010;24:2144–2151.
- Crockford D, Turjman N, Allan C, et al. Thymosin beta4: structure, function, and biological properties supporting current and future clinical applications. Ann N Y Acad Sci. 2010;1194:179–189.
- Ruff D, Crockford D, Girardi G, et al. A randomized, placebo-controlled, single and multiple dose study of intravenous thymosin beta4 in healthy volunteers. Ann N Y Acad Sci. 2010;1194:223–229.
- Smart N, Bollini S, Dube KN, et al. De novo cardiomyocytes from within the activated adult heart after injury. Nature. 2011;474:640–644.
- Xiong Y, Mahmood A, Meng Y, et al. Treatment of traumatic brain injury with thymosin β4 in rats. J Neurosurg. 2011;114:102–115.
- Zhang J, Zhang ZG, Morris D, et al. Neurological functional recovery after thymosin beta4 treatment in mice with experimental auto encephalomyelitis. Neuroscience. 2009;164:1887–1893.
- Kozaczuk A, Selmi A, Bednarek R. Bacterial expression, purification and angiogenesis-promoting activity of human thymosin β4. Protein Expr Purif. 2013;90:142–152.
- Li T, Ma SY, Tang XC, et al. Production and characterization of highly purified recombinant thymosin beta 4 in Escherichia coli. Protein Expr Purif. 2013;90:90–95.
- Tsuji Y, Kitahara-Tanabe N, Noguchi K, et al. Production in Escherichia coli of human thymosin beta 4 as chimeric protein with human tumor necrosis factor. Biochem Int. 1989;18:501–508.
- Mackay DT, Botting CH, Taylor GL, et al. An acetylase with relaxed specificity catalyses protein N-terminal acetylation in Sulfolobus solfataricus. Mol Microbiol. 2007;64:1540–1548.
- Ren Y, Yao X, Dai H, et al. Production of Nα-acetylated thymosin α1 in Escherichia coli. Microb Cell Fact. 2011;10:26.
- Urbancikova M, Hitchcock-DeGregori SE. Requirement of amino-terminal modification for striated muscle alpha-tropomyosin function. J Biol Chem. 1994;269:24310–24315.
- Rudman D, Hollins BM, Kutner MB, et al. Three type of α-melanocyte-stimulating hormone: bioactivity and half-lives. Am J Physiol. 1983;245:E47–E54.