Abstract
The increasing prevalence of the metabolic syndrome has made it a medical issue that currently affects 1 in 5 Canadians. The metabolic syndrome is defined by risk factors that predispose an individual to diabetes and cardiovascular disease. Current forms of interventions have been inadequate as substantiated by the fact that the prevalence of metabolic syndrome has not reduced over the years. The objective of this study was to investigate the therapeutic benefits of a novel microencapsulated probiotic blend in treating the metabolic syndrome. Three probiotic strains were microencapsulated into alginate-polylysine-alginate (APA) microcapsules: L. rhamnosus NCIMB 6375, L. plantarum NCIMB 8826 and L. fermentum NCIMB 5221. From the results, it was observed that the microencapsulated probiotic blend significantly reduced serum total cholesterol, LDL cholesterol and triglyceride levels (reducing from 516 mg/dL to 379 mg/dL, 314 mg/dL to 231 mg/dL and 580 mg/dL to 270 mg/dL, respectively). In addition, the administration of the microencapsulated probiotic blend was found to favourably influence the gut microbiota, decreasing Firmicutes levels and increasing Bacteroidetes levels. Overall, this work demonstrates the potential a microencapsulated probiotic blend could have in targeting multiple risk factors of the metabolic syndrome; however, greater research is still needed.
Introduction
The metabolic syndrome is characterized by the grouping of risk factors that predispose an individual to cardiovascular disease and type 2 diabetes mellitus [Citation1]. Currently, this syndrome has five risk factors: increased abdominal fat, high blood pressure, high fasting blood glucose, low high-density lipoprotein (HDL) and elevated triglyceride levels [Citation1]. To be diagnosed with the metabolic syndrome, one has to have three of the five risk factors [Citation2]. The primary cause of the metabolic syndrome is rooted in a sedentary life style, and a high-fat diet [Citation3]. Between the years 2009 and 2011, the Canadian Health Measures Survey found that over 20% of the adult Canadian population had this syndrome [Citation2].
Current forms of interventions include lifestyle changes [Citation3–6], medication and surgery for extreme cases. However, even with their benefits, each has their own shortcomings. These limitations include not being effective for different individuals, adverse effects associated with medications, and further complications that can come from surgical intervention [Citation3,Citation7,Citation8]. Therefore, an alternative form of therapy is needed to treat the risk factors of the metabolic syndrome.
Probiotics have gained much attention in recent years for their potential benefits in treating various diseases [Citation9–11], including the metabolic syndrome [Citation12,Citation13]. The definition of probiotics has been recently updated to, “Live microorganisms that, when administered in adequate amounts, confer a health benefit on the host”. Furthermore, a panel of experts also recommended that only those microbial species that have been proven to confer benefits to health through controlled studies can be defined as probiotics. The panel also suggested that live cultures associated with fermented foods as well as undefined faecal microbiota which have no evidence of health benefits should not be included as probiotics [Citation14]. The related term prebiotic has been defined as “a substrate that is selectively utilized by host microorganisms conferring a health benefit” [Citation15]. Of the different types of probiotics studied, strains from the Lactobacillus and Bifidobacterium species have shown the greatest potential in targeting the risk factors that characterize the metabolic syndrome [Citation12,Citation13,Citation16]. The use of multiple probiotic strains simultaneously has also been suggested to bring together the many benefits associated with each individual probiotic [Citation17–19].
Even with the benefits that come with probiotic consumption, their administration is still limited due to their low survivability as they pass through the harsh conditions of the gastrointestinal (GI) tract [Citation20]. Artificial cells, which were first developed by Dr Thomas M.S. Chang, is one method by which therapeutics could be delivered [Citation21]. Alginate-polylysine-alginate (APA) microcapsules have been used extensively for the protection and delivery of several therapeutic agents including probiotics [Citation22,Citation23]. The use of APA microcapsules has been found to significantly increase the viability of probiotics as they pass the GI tract [Citation20].
A small animal model would provide valuable information as to the efficacy of a designed intervention in treating the metabolic syndrome. Of the species that are available for use, hamsters have been found to have a similar lipid profile to humans, and respond similarly to changes in diet [Citation24]. For this reason, hamsters were chosen for this study.
The present study investigated the therapeutic benefits of a microencapsulated probiotic blend in the metabolic syndrome. The blend consisted of three probiotic strains: Lactobacillus rhamnosus NCIMB 6375, Lactobacillus plantarum NCIMB 8826 and Lactobacillus fermentum NCIMB 5221. L. plantarum NCIMB 8826 has been studied extensively, showing to positively interact with the host and influence the immune system. In addition, L. plantarum NCIMB 8826 has been associated with lipid reduction through its bile salt hydrolase activity [Citation25,Citation26]. Studies have shown L. fermentum NCIMB 5221 to be able to reduce insulin resistance, LDL-cholesterol levels and triglyceride levels [Citation13]. Additionally, this strain has ferulic acid esterase activity, which studies have found to have antioxidant and anti-inflammatory properties, along with improving one’s lipid profile [Citation27–31]. Bacterial strains of L. rhamnosus have been found to improve glucose tolerance and reduce cholesterol levels [Citation32,Citation33].
To date, the use of a probiotic blend microencapsulated in APA microcapsules for the treatment of the metabolic syndrome has not been studied and this will be the first of its kind.
Materials and methods
Preparation of selected bacterial cells from bacterial glycerol stock
The bacteria strains, Lactobacillus rhamnosus NCIMB 6375, Lactobacillus plantarum NCIMB 8826 and Lactobacillus fermentum NCIMB 5221, were streaked onto separate De Man, Rogosa, and Sharpe (MRS) agar plates from their respective glycerol stock and incubated for 16 h at 37 °C. The MRS solution was prepared in lab according to previous methods [Citation34]. To make the agar plates, 15 g of agar (Bioshop Canada, Ontario, Canada) was added per liter of MRS solution. After a 16-h incubation, a single colony from each plate was seeded in separate tubes containing MRS solution. The seeded solution was then incubated for 16 h at 37 °C, and then stored at 4 °C.
Preparation of the probiotic blend
The probiotic blend was prepared according to the following procedure. Each of the bacteria species were grown individually by taking 6 ml from their respective stock solution and adding it to 600 ml of sterile MRS solution (1% (v/v) inoculum). The three solutions were then kept at 37 °C for 8–9 h to allow the bacteria to grow in numbers. To determine the approximate number of bacteria cells (in terms of colony forming units, CFU), a 1 ml sample was removed from the solution and was serially diluted. The absorbance of the serially diluted solutions was then measured using the Evolution 60 s UV–Visible Spectrophotometer (Thermo Scientific). A previously made standard curve that relates absorbance values with CFUs/mL was used to associate the measured absorbance value with a CFU/mL value.
The remainder of each solution was centrifuged at 4000 rpm for 10 min at 4 °C. The MRS solution was decanted, and the bacterial pellet was washed. The washing procedure consisted of resuspending the bacteria in 0.9% saline and then centrifuging with the same parameters. This was repeated twice, after which the bacteria pellet was resuspended in <10 ml of 0.9% saline.
Microencapsulation of selected probiotic blend in APA microcapsules
Equal proportions of L. rhamnosus NCIMB 6375, L. plantarum NCIMB 8826 and L. fermentum NCIMB 5221 were added to sterilized 1.6% (w/v) sodium alginate (Sigma-Aldrich, Ontario, Canada) solution. The final probiotic concentration in the alginate solution was set to 2.15 × 1010 CFU/mL (combined dosage of all three strains). Once the alginate/bacteria solution was homogeneous, it was transferred to a 60-ml syringe and hooked up to the inotech encapsulator IER-20. The parameters were set at a speed of 20, frequency of 1800 Hz, and a voltage of 1.000 kV, and a 200 µm nozzle was used. The solution formed a chain of beads as it exited the machines nozzle and entered a gently stirred bath containing 100 mM of CaCl2 (Anachemia, Quebec, Canada). The beads were allowed to harden in the bath for five minutes, after which, the beads were washed by removing 2/3 of the solution and adding the same amount of sterile 0.9% saline. Washing was done a total of three times. Following the last wash, 300 ml of filter sterilized 0.1% (w/v) E-polylysine (Zhejian Silver Elephant Bioengineering Co., Ltd, China) was added to the solution, and was gently stirred at 150 rpm for 20 min to allow the formation of the first layer around the alginate core. The microcapsules were then washed again as before. Finally, a sterile solution containing 0.1% sodium alginate was added, and the solution was gently stirred for another 20 min. The microcapsules were then washed again three times, resulting in the final APA capsules. A solution made up of 90% saline and 10% MRS media was used to store the microcapsules for a period up to two weeks as mentioned by previous studies [Citation35,Citation36].
Experimental hamster model for the investigation of the APA microencapsulated probiotic blend
Twenty 8-week-old male Golden Syrian Hamsters weighing approximately 80 g were obtained from Charles Rivers Laboratories. The hamsters were separated into cages, each of which had two hamsters. The hamsters remained in a controlled environment with alternating light and dark cycles (12 h each changing at 7 am and 7 pm), and a temperature of 22 °C. All experimental protocols were in accordance with the Animal Care Committee of McGill University and Canadian Council on Animal Care guidelines.
Experimental protocol for hamster model
After arriving, the hamsters were acclimatized for two weeks, having free access to food and water. The hamsters were then separated into two groups: five hamsters remained on the normal diet (Standard Rodent LabDiet 5001), while the other 15 hamsters were put on a high fat diet (HFD), which was made in lab according to previous mentioned studies [Citation37–39]. The HFD consisted of 10% fructose water (Fisher Scientific, Ottawa, Ontario, Canada) and food made up of: Standard Rodent LabDiet 5001, 11.5% corn oil, 11.5% coconut oil, 0.5% cholesterol (Sigma-Aldrich, Oakville, Ontario, Canada) and 0.25% deoxycholate (Sigma-Aldrich, Ontario, Canada). The hamsters remained on their respective diets for the remainder of the study. After five weeks, the hamsters that were on the HFD showed an increase in weight, when compared to the hamsters that were kept on the normal diet. Furthermore, previous studies have found the selected HFD to elevate serum cholesterol levels, increase triglyceride levels and increase blood glucose levels within five weeks [Citation37–39]. The hamsters were now considered to have the metabolic syndrome and the six-week treatment period began. The hamsters in the high-fat diet group were divided into three groups for the remainder of the study. From this point onwards, a total of four groups of hamsters existed: (1) Normal diet, (2) HFD with 0.9% saline, (3) HFD with empty APA microcapsules and (4) HFD with microencapsulated probiotic blend. Each form of treatment was given daily at the same time over the next six weeks. The probiotic dosage was kept constant at 2.86×1010 total CFU/kg daily. For saline and empty microcapsule treatment, a volume of 800 µL was given, which was roughly the same volume as the probiotic treatment. Following the treatment period, daily probiotic treatment was suspended, and a four-week washout period followed. At the end of this period, the study was terminated, and the hamsters were sacrificed, with their blood being collected through cardiac puncture.
Animal food and water consumption as well as their weight were monitored and recorded. Blood samples were taken through the saphenous vein, with serum being collected by allowing the blood to coagulate and then centrifuging at 4000 rpm at 4 °C for 25 min. Fecal samples were collected by leaving the hamsters in clean cages for 18 h, and then collecting the fecal samples in the cage.
Investigation of impact of microencapsulated probiotic blend on hamster serum total cholesterol levels
Serum total cholesterol levels were quantified through the use of a method suggested by Rudel L.L. and Morris M.D. with some modifications. First, 50 µL of KOH (Fisher Scientific, Ontario, Canada), 100 µL of absolute ethanol (Commercial Alcohols, Ontario, Canada) and 10 µL of serum were combined and vortexed for 1 min, followed by a 15 min incubation at 37 °C. Following incubation, the solution was taken out of the incubator and left to cool at room temperature for 10 min. To each solution, 100 µL of deionized water and 200 µL of hexane (ACP Chemicals, Quebec, Canada) were added, followed by 1 min of vortex. Once the two layers had separated, 50 µL of the hexane layer (top layer) was extracted and put into a clean tube. Nitrogen gas was then used to evaporate the hexane solution. Next, 200 µL of the reagent (0.05 g FeCl3 (Sigma-Aldrich, Ontario, Canada) dissolved in 100 ml of acetic acid (Fisher Scientific, Ontario, Canada)) and 125 µL of concentrated H2SO4 (Fisher Scientific, Ontario, Canada) were added to each tube. The samples were vortexed for 1 min and kept at room temperature for 20 min in the dark. The absorbance was then read at 570 nm [Citation40,Citation41].
Investigation of impact of microencapsulated probiotic blend on hamster serum HDL and LDL/VLDL-cholesterol levels
To determine hamster serum HDL cholesterol and LDL/VLDL cholesterol levels, the method suggested by Russell W. G. was used. A reagent was made by combining a 10 ml solution containing 0.2 g of dextran sulfate (Mr 40 000, Alfa Aesar, West Virginia, U.S.A.), with a 10 ml solution containing 2.03 g of MgCl2·6 H2O (Fisher Scientific, Ontario, Canada), adjusting the pH to 7.0. After the sample and reagent reached room temperature, 2 µL of the prepared reagent was added to 20 µL of the serum sample. The sample was then vortexed for roughly 3 s and was kept at room temperature for 10 min. Next, the sample was centrifuged for 30 min at 4500 rpm and 4 °C. The pellet was removed from the solution and resuspended in 20 µL of 0.9% saline. The cholesterol assay procedure mentioned above was used to quantify HDL cholesterol levels and LDL/VLDL cholesterol levels. The cholesterol present in the pellet solution represents the LDL/VLDL cholesterol levels, whereas the cholesterol present in the supernatant represents the HDL cholesterol levels. [Citation42].
Investigation of impact of microencapsulated probiotic blend on hamster serum triglyceride levels
Through the use of a triglyceride reagent (Stanbio Laboratory Triglyceride Liquicolor), hamster serum triglyceride was quantified according to the procedure provided with the reagent (Thermo Scientific).
Investigation of impact of microencapsulated probiotic blend on hamster serum inflammatory marker C-reactive protein
Hamster Serum CRP was measured with an ELISA kit (MyBioSource, Hamster High Sensitivity C-Reactive Protein Elisa Kit).
Hamster gut microbiota population quantification
Hamster gut bacteria populations were quantified through the use of Polymerase Chain Reaction (PCR). The first step was extracting bacteria DNA from fecal samples. To do so, one fecal sample was homogenized in 500 µL Tris-EDTA (0.1 M Tris (Bio-Rad Laboratories, Quebec, Canada), 1 mM EDTA (Bioshop Canada, Ontario, Canada (pH 7.4)), followed by the addition of 20 µL of 25% (v/v) Triton X-100 (Sigma-Aldrich, Ontario, Canada). Next, 50 µL 200 mg/mL lysozyme (Sigma-Aldrich, Ontario, Canada) was added. The sample was then vortexed for roughly three seconds and incubated for 3–4 h at 42 °C. Post incubation, the One-4-All Genomic DNA Mini-Preps Kit (Bio Basic Canada Inc., Ontario, Canada) was used, following the procedure provided. A Nanodrop (NanoDrop 2000 spectrophotometer, Thermoscientific) was used to quantify the final DNA concentration.
The second step of this method was to use conventional PCR. In a 100 µL tube, a sample volume containing 1 µg of DNA (made during the prior step) was topped off with ultrapure distilled water to a volume of 13.5 µL. This was followed with the addition of 6.5 µL of the master mix (2 µL 10× Taq Reaction Buffer, 2 µL of 2 mmol magnesium sulfate, 0.4 µL of 25 mM dNTPs, 0.4 µL of 3 µM PI, 0.4 µL of 3 µM PC and 0.1 µL of Taq DNA Polymerase (all from Bio Basic Canada Inc., Ontario, Canada)). The tubes were inserted into the PCR (Mastercycler gradient, Eppendorf) for 10 cycles.
On the completion of conventional PCR, each sample was cleaned through the use of EZ-10 Spin Column PCR Products Purification Kit (Bio Basic Canada Inc., Ontario, Canada), was used.
The samples were now ready for the final step, real time PCR. A master mix was prepared and contained the following: 5 parts EvaGreen 2× qPCR (Applied Biological Materials, British Columbia, Canada), 1 part forward primer, 1 part reverse primer and 1 part ultrapure distilled water. Using a 48-well plate, a volume of 8 µL of master mix was first added first followed by the addition of 2 µL of sample. The plate was then covered and placed into the Eco Ilumina (MBI Lab Equipment), which ran for 40 cycles. Two bacteria populations were quantified: Firmicutes and Bacteroidetes. All samples were normalized using the housekeeping gene (Uni2), with the final results relative to the control group at the start of the study. Their corresponding primers and annealing temperatures are listed in . To determine relative gene expression, 2−ΔΔCT method was used.
Table 1. Primers used for quantifying bacteria gene expression.
Statistical analysis
GraphPad Prism 7.03 was used for statistical analysis. Data were represented as mean ± standard deviation. Two-way ANOVA (Tukey’s correction) was employed to verify if there was a significant change in the mean of the treatment group with previous time points or the control groups. Differences between various groups were considered significant at a 95% confidence interval (p < .05).
Results
Production of APA microcapsules containing the probiotic blend
The microencapsulation of the probiotic blend was performed to protect the probiotic blend from harsh conditions of the GI tract. APA microencapsulation was successful using the mentioned parameters. A light microscope was used to visualize the microcapsules at the various stages of APA microcapsule formation. illustrates the final APA microcapsule as well as the intermediate steps at 10× magnification. It can be observed that the microcapsules were of uniform size and shape, having an average size of 400 ± 18 µm.
Figure 1. Steps in producing APA microcapsules. APA microcapsule formation consisted of three steps as illustrated. Final microcapsules were found to be spherical with an approximate diameter of 400 ± 18 µm.
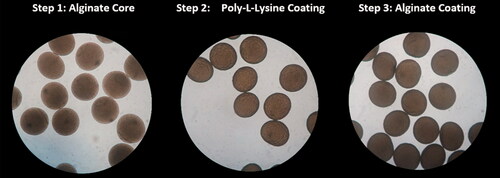
Hamster weight throughout the span of the study
The metabolic syndrome is characterized with increased abdominal obesity which correlates with weight gain. From , it can be seen that prior to the treatment phase, the hamsters that were on a high-fat diet were heavier than their counterparts that received a normal diet. Focussing on the 6-week intervention period, the hamsters that received the microencapsulated probiotic blend were noted to have an initial weight loss followed by a weight gain, however, the findings were not significant. During the final washout period, no significant change was observed either.
Investigation of impact of microencapsulated probiotic blend on hamster serum lipid profile
Abnormal cholesterol and triglyceride levels are defining characteristics of the metabolic syndrome. Monitoring changes in hamster serum lipid profile would enable a better understanding as to the efficacy of the designed treatment in countering the metabolic syndrome. Total cholesterol levels, LDL-cholesterol, HDL-cholesterol and triglyceride levels of the different hamster groups at selected time points of the study can be observed in . By the end of the 5-week period following the acclimatization period, the hamsters on the high-fat diet showed a significant increase in serum total cholesterol levels, LDL-cholesterol levels and triglyceride levels (p < .05). Following the 6-week treatment period, the hamsters that received to microencapsulated probiotic blend treatment had a significant reduction is serum total cholesterol, LDL-cholesterol and triglyceride levels (515 ± 46 mg/dL to 379 ± 27 mg/dL (p < .05), 314 ± 46 mg/dL to 231 ± 22 mg/dL (p < .05) and 580 ± 211 mg/dL to 270 ± 21 mg/dL (p < .05), respectively). Serum total cholesterol, LDL-cholesterol and triglyceride levels of the hamsters that received the microencapsulated probiotic blend were also significantly lower compared to the control group that received empty microcapsules (p < .05), indicating that the microcapsule material is not causing any therapeutic effect in relation to these parameters. Following the 4-week wash out period, serum total cholesterol, LDL-cholesterol and triglyceride levels of the hamsters that received the microencapsulated probiotic blend remained significantly lower than the high-fat control groups (p < .05). The microencapsulated probiotic treatment did not show to significantly alter HDL-cholesterol levels as observed in .
Figure 3. Impact of microencapsulated probiotic blend treatment on hamster serum total cholesterol levels. The microencapsulated probiotic blend showed a significant reduction in total cholesterol levels when compared to the high-fat diet control groups (p < .05). (*) – Significant reduction compared to high-fat control group at the same time point. (N = 5 hamsters).
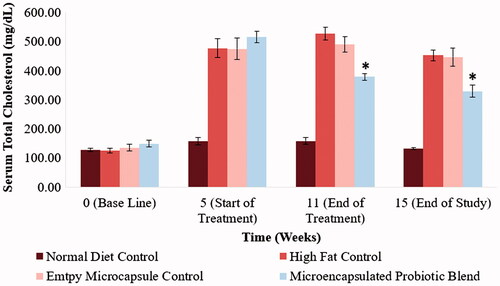
Figure 4. Effect of microencapsulated probiotic blend on hamster serum LDL-cholesterol levels at selected time points. The microencapsulated probiotic blend caused a significant reduction in LDL-cholesterol levels (p < .05). (N = 5 hamsters). (*) – Significant reduction compared to high-fat control group at the same time point.
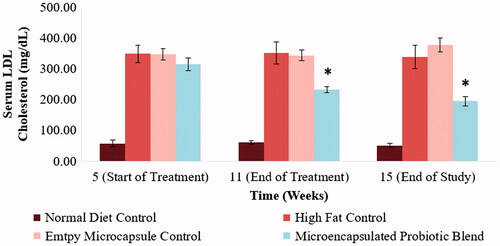
Figure 5. Effect of microencapsulated probiotic blend treatment on hamster serum HDL-cholesterol. No significant change was observed amongst the treatment groups. (N = 5 hamsters).
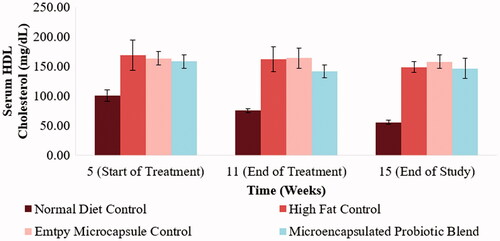
Figure 6. Effect of microencapsulated probiotic blend treatment on hamster serum triglyceride levels. The microencapsulated probiotic blend caused a significant reduction of triglyceride levels (p < .05). (N = 5). (*) – Significant reduction compared to high-fat control group at the same time point.
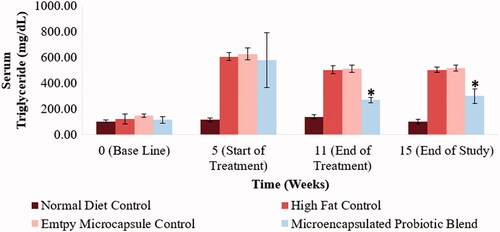
Investigation of impact of microencapsulated probiotic blend on hamster serum C-reactive protein levels
The metabolic syndrome has also been characterized by a pro-inflammatory state. The inflammatory marker C-Reactive Protein (CRP) was chosen as the marker of choice to quantify inflammation. At baseline values (Week 0), all the hamsters were found to have relatively equal values of serum CRP levels. At the end of the 5-week high-fat diet period, the hamsters on the high-fat diet showed an increase in CRP levels, however, not significantly higher than the normal diet control group (p > .05). Following the 6-week treatment period, the microencapsulated groups (both the empty microcapsule and the microencapsulated probiotic blend), showed an increase in CRP levels after being administered, however, not significantly (p > .05). These results can be visualized in .
Impact of selected probiotic treatments on gut microbiota
The role of the gut microbiota on metabolism has been well documented, as well as the role dysbiosis can play on the development of the metabolic syndrome. Changes in the gut bacterial populations would provide information as to whether the administered treatment had a favourable influence on the gut microbiota.
Impact of microencapsulated probiotic blend on Firmicutes
Previous studies have found that as one loses weight, a reduction in gut Firmicutes levels is also observed. This study verified if the designed microencapsulated probiotic blend caused a similar trend on hamsters on a high-fat diet. From , changes in hamster gut Firmicutes levels can be observed. Following an initial 5-week period, the hamsters that were on a high-fat diet showed a significant increase in gut Firmicutes levels. The hamsters that remained on the normal diet throughout the study did not show significant changes in Firmicutes levels throughout the study. It should be noted that the high-fat control group as well as the empty microcapsule control group did not have significant changes in gut Firmicutes levels following the initial 5-week period. At the end of the 6-week treatment period, the microencapsulated probiotic blend showed a significant reduction (p < .001) in gut Firmicutes levels. However, gut Firmicutes levels showed to increase after the washout period.
Figure 8. Influence of microencapsulated probiotic blend treatment on hamster gut Firmicutes. The microencapsulated probiotic blend caused a significant reduction in Firmicutes levels by the end of the treatment period (p < .05). (N = 3). (*) – Significant reduction compared to values at the start of probiotic treatment.
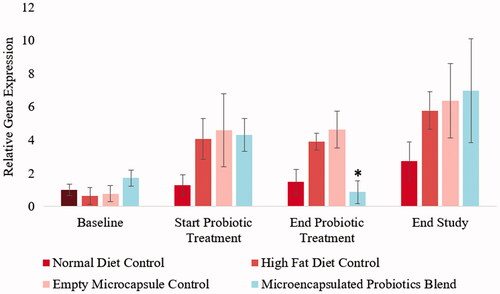
Impact of microencapsulated probiotic blend on bacteroidetes
A trend towards weight loss has been associated with an increase in Bacteroidetes population in the gut. The current study quantified changes in hamster gut Bacteroidetes levels at four different time points. Hamsters in the normal diet control group did not show significant changes in Bacteroidetes levels throughout the study. During the initial 5-week period following acclimatization, the hamsters that were on a high-fat diet showed an increase in gut Bacteroidetes levels. At the end of the 6-week treatment period, the hamsters that received the treatment did not show to have significantly higher Bacteroidetes levels, however, a trend towards significance was noted (p = .0762). Furthermore, a significant increase in Bacteroidetes was observed after the washout period (p < .001). These results are illustrated in .
Figure 9. Influence of microencapsulated probiotic blend treatment on hamster gut Bacteroidetes. The microencapsulated probiotic blend also showed to increase the probiotic blend, but not significantly (p = .0762, trend towards significance) by the end of the treatment period. The treatment did however have a significant increase in Bacteroidetes levels by the end of the study (p < .05). (N = 3). (*) – Significant increase compared to values at the start of probiotic treatment.
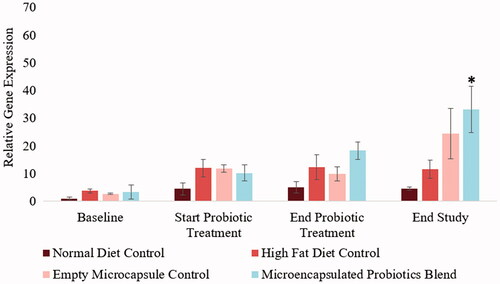
Discussion
Being diagnosed with the metabolic syndrome increases once chances of developing both cardiovascular disease and type 2 diabetes [Citation1]. As the prevalence of this syndrome amongst Canadians has only increased over the years [Citation43], alternative forms of treatment need to be researched to address this problem. Probiotics have gained much attention in recent years as a possible solution; however, they are still limited due to their low survival through the gastrointestinal tract. For this reason, the current study investigated APA microcapsules as a method of delivery for a probiotic blend.
Through the use of microencapsulation, it was anticipated that probiotic delivery to the intestine, and hence its survivability, would be improved, as suggested in previous work [Citation20]. This study found the use of the microencapsulated probiotic blend on hamsters on a high-fat diet to have numerous therapeutic benefits. After a six-week treatment period, the daily administration of this therapeutic was observed to significantly reduce total cholesterol levels, LDL cholesterol levels and triglyceride levels by approximately 36.1%, 37.8% and 48.0%, respectively.
The amelioration of hamster lipid profile associated with the consumption of the microencapsulated probiotic blend can be attributed to the probiotics that make up the blend. One mechanism by which probiotics reduce cholesterol levels is through their production of bile salt hydrolase enzymes (BSH). The production of BSH has been reported in numerous probiotics [Citation44,Citation45] including L. plantarum NCIMB 8826 [Citation46]. Furthermore, the presence of ferulic acid esterase activity in L. fermentum NCIMB 5221 has also been associated with a number of benefits [Citation13,Citation20]. This enzyme is responsible for freeing ferulic acid from its bonded state in the cell wall of plants, allowing a larger amount to be absorbed by the body [Citation29,Citation47–49]. The benefits associated with the consumption and absorption of ferulic acid include reduction in total cholesterol levels [Citation30], antioxidant [Citation29] and anti-inflammatory properties [Citation31,Citation50].
In addition to the risk factors of the metabolic syndrome, this syndrome has also been associated with a proinflammatory state [Citation51]. The present study investigated the anti-inflammatory properties of the microencapsulated probiotic blend by quantifying serum CRP levels. The administration of APA microcapsules was observed to cause no significant shift in serum CRP levels, however, when compared to the CRP levels of the high-fat control group, it was observed that the latter stayed almost constant throughout the treatment and washout period, whereas the microcapsule group did not. The hamsters that received microcapsules showed to have an increase in CRP levels by the end of the treatment period (however not significant), and then a reduction in CRP levels after the treatment ended. These results were surprising as both L. fermentum NCIMB 5221 and L. plantarum NCIMB 8826, which are present in the blend, have been found to have anti-inflammatory properties [Citation26,Citation50]. These findings postulate that APA microcapsules could be causing an inflammatory response, but due to the findings not being significant, no concrete conclusion can be made. It has been suggested that polylysine could induce an inflammatory response [Citation52]. However, the same study also found that combining polylysine with alginate, and maintaining a low content of polylysine, as done in the present study, would reduce the inflammatory response [Citation52]. Further investigation would need to be done to have a better understanding as to why this was observed.
An unfavourable alteration in gut bacteria populations, known as dysbiosis, has been related to the metabolic syndrome, diabetes and non-alcoholic fatty liver disease [Citation53]. A study has found that the relative size of the two largest phyla of bacteria in the gut, Firmicutes and Bacteroidetes, differ amongst obese and lean individuals. Firmicutes levels have been found to be higher in obese individuals when compared to lean individuals [Citation54]. Whereas Bacteroidetes have been observed to increase in numbers as one loses weight [Citation55]. The designed microencapsulated treatment was found to significantly reduce Firmicutes levels by the end of the treatment period while significantly increasing Bacteroidetes levels by the end of the study. This would indicate that the designed treatment has a positive influence on the gut microbiota.
Overall, the present study found the use of the novel microencapsulated probiotic blend to be beneficial in addressing the different risk factors associated with the metabolic syndrome. Greater work is still required to have a better understanding as to how this treatment works and if the administration of APA capsules to the GI tract causes an inflammatory response. The aim of microencapsulation was to enhance probiotic efficacy, and the current treatment employed was found to significantly improve hamster lipid profile and have a favourable influence on the gut microbiota.
Conclusions
This work demonstrated the use of a novel microencapsulated probiotic blend for the treatment of the metabolic syndrome. This treatment was found to improve hamster lipid profile, positively influence the gut microbiota, and covered factors that characterize the metabolic syndrome. Further research would need to be done to have a better understanding as to how this treatment works and how it can be optimized for maximal efficacy.
Disclosure statement
No potential conflict of interest was reported by the authors.
Additional information
Funding
References
- Alberti KG, Eckel RH, Grundy SM, et al. Harmonizing the metabolic syndrome: a joint interim statement of the International Diabetes Federation Task Force on Epidemiology and Prevention; National Heart, Lung, and Blood Institute; American Heart Association; World Heart Federation; International Atherosclerosis Society; and International Association for the Study of Obesity. Circulation. 2009;120:1640–1645.
- Statistics-Canada. Metabolic syndrome in Canadians, 2009 to 2011. In: Division HS, editor. Canada: Minister of Industry; 2012.
- Park YW, Zhu S, Palaniappan L, et al. The metabolic syndrome: prevalence and associated risk factor findings in the US population from the Third National Health and Nutrition Examination Survey, 1988-1994. Arch Intern Med. 2003;163:427–436.
- Lindstrom J, Louheranta A, Mannelin M, et al. The Finnish Diabetes Prevention Study (DPS): lifestyle intervention and 3-year results on diet and physical activity. Diabetes Care. 2003;26:3230–3236.
- Alberti KG, Zimmet P, Shaw J . The metabolic syndrome – a new worldwide definition. Lancet. 2005;366:1059–1062.
- Ford ES, Giles WH, Dietz WH. Prevalence of the metabolic syndrome among US adults: findings from the third National Health and Nutrition Examination Survey. Jama. 2002;287:356–359.
- Shettar V, Patel S, Kidambi S. Epidemiology of obesity and pharmacologic treatment options. Nutrition in Clinical Practice: USA. 2017.
- Miller ML, Wright CC. Use of lipid lowering medications in youth. In: De Groot LJ, Chrousos G, Dungan K, Feingold KR, Grossman A, Hershman JM, et al., editors. Endotext. South Dartmouth (MA): MDText.com, Inc.; 2000.
- Lollo PCB, Morato PN, de Moura CS, et al. Ultra-high temperature plus dynamic high pressure processing: an effective combination for potential probiotic fermented milk processing which attenuate exercise-induced immune suppression in Wistar rats. J Funct Foods. 2015;14:541–548.
- Moura CS, Lollo PCB, Morato PN, et al. Assessment of antioxidant activity, lipid profile, general biochemical and immune system responses of Wistar rats fed with dairy dessert containing Lactobacillus acidophilus La-5. Food Res Int. 2016;90:275–280.
- Lollo PCB, Morato PN, Moura CS, et al. Hypertension parameters are attenuated by the continuous consumption of probiotic Minas cheese. Food Res Int. 2015;76:611–617.
- Li Z, Jin H, Oh SY, et al. Anti-obese effects of two Lactobacilli and two Bifidobacteria on ICR mice fed on a high fat diet. Biochem Biophys Res Commun. 2016;480:222–227.
- Tomaro-Duchesneau C, Saha S, Malhotra M, et al. Effect of orally administered L. fermentum NCIMB 5221 on markers of metabolic syndrome: an in vivo analysis using ZDF rats. Appl Microbiol Biotechnol. 2014;98:115–126.
- Hill C, Guarner F, Reid G, et al. Expert consensus document. The International Scientific Association for Probiotics and Prebiotics consensus statement on the scope and appropriate use of the term probiotic. Nat Rev Gastroenterol Hepatol. 2014;11:506–514.
- Gibson GR, Hutkins R, Sanders ME, et al. Expert consensus document: The International Scientific Association for Probiotics and Prebiotics (ISAPP) consensus statement on the definition and scope of prebiotics. Nat Rev Gastroenterol Hepatol. 2017;14:491–502.
- Chen JJ, Wang R, Li XF, et al. Bifidobacterium longum supplementation improved high-fat-fed-induced metabolic syndrome and promoted intestinal Reg I gene expression. Exp Biol Med (Maywood). 2011;236:823–831.
- Tsai CC, Lin PP, Hsieh YM, et al. Cholesterol-lowering potentials of lactic acid bacteria based on bile-salt hydrolase activity and effect of potent strains on cholesterol metabolism in vitro and in vivo. ScientificWorldJournal. 2014;2014:1.
- Ahn HY, Kim M, Chae JS, et al. Supplementation with two probiotic strains, Lactobacillus curvatus HY7601 and Lactobacillus plantarum KY1032, reduces fasting triglycerides and enhances apolipoprotein A-V levels in non-diabetic subjects with hypertriglyceridemia. Atherosclerosis. 2015;241:649–656.
- Yoo SR, Kim YJ, Park DY, et al. Probiotics L. plantarum and L. curvatus in combination alter hepatic lipid metabolism and suppress diet-induced obesity. Obesity (Silver Spring). 2013;21:2571–2578.
- Tomaro-Duchesneau C, Saha S, Malhotra M, et al. Probiotic ferulic acid esterase active Lactobacillus fermentum NCIMB 5221 APA microcapsules for oral delivery: preparation and in vitro characterization. Pharmaceuticals (Basel, Switzerland). 2012;5:236–248.
- Chang TMS. Artificial cells: biotechnology, nanomedicine, regenerative medicine, blood substitutes, bioencapsulation, cell/stem cell therapy. Singapore: World Scientific Publishing Co.; 2007.
- Bhathena J, Martoni C, Kulamarva A, et al. Oral probiotic microcapsule formulation ameliorates non-alcoholic fatty liver disease in Bio F1B Golden Syrian hamsters. PloS One. 2013;8:e58394.
- Prakash S, Chang TM. Microencapsulated genetically engineered live E. coli DH5 cells administered orally to maintain normal plasma urea level in uremic rats. Nat Med. 1996;2:883–887.
- Spady DK, Dietschy JM. Dietary saturated triacylglycerols suppress hepatic low density lipoprotein receptor activity in the hamster. Proc Natl Acad Sci USA. 1985;82:4526–4530.
- van den Nieuwboer M, van Hemert S, Claassen E, et al. Lactobacillus plantarum WCFS1 and its host interaction: a dozen years after the genome. Microb Biotechnol. 2016;9:452–465.
- Vesa T, Pochart P, Marteau P. Pharmacokinetics of Lactobacillus plantarum NCIMB 8826, Lactobacillus fermentum KLD, and Lactococcus lactis MG 1363 in the human gastrointestinal tract. Aliment Pharmacol Ther. 2000;14:823–828.
- Tomaro-Duchesneau C, Saha S, Malhotra M, et al. Lactobacillus fermentum NCIMB 5221 and NCIMB 2797 as cholesterol-lowering probiotic biotherapeutics: in vitro analysis. Benef Microbes. 2015;6:861–869.
- Tomaro-Duchesneau C, Jones ML, Shah D, et al. Cholesterol assimilation by Lactobacillus probiotic bacteria: an in vitro investigation. BioMed Res Int. 2014;2014:1.
- Ou S, Kwok K-C. Ferulic acid: pharmaceutical functions, preparation and applications in foods. J Sci Food Agric. 2004;84:1261–1269.
- Kim HK, Jeong TS, Lee MK, et al. Lipid-lowering efficacy of hesperetin metabolites in high-cholesterol fed rats. Clin Chim Acta. 2003;327:129–137.
- Sakai S, Kawamata H, Kogure T, et al. Inhibitory effect of ferulic acid and isoferulic acid on the production of macrophage inflammatory protein-2 in response to respiratory syncytial virus infection in RAW264.7 cells. Mediators Inflam. 1999;8:173–175.
- Park KY, Kim B, Hyun CK. Lactobacillus rhamnosus GG improves glucose tolerance through alleviating ER stress and suppressing macrophage activation in db/db mice. J Clin Biochem Nutr. 2015;56:240–246.
- Schoemaker MH, Kleemann R, Morrison MC, et al. A casein hydrolysate based formulation attenuates obesity and associated non-alcoholic fatty liver disease and atherosclerosis in LDLr-/-.Leiden mice. PloS One. 2017;12:e0180648.
- Watson D, O'Connell Motherway M, Schoterman MH, et al. Selective carbohydrate utilization by lactobacilli and bifidobacteria. J Appl Microbiol. 2013;114:1132–1146.
- Bhathena J, Kulamarva A, Urbanska AM, et al. Microencapsulated bacterial cells can be used to produce the enzyme feruloyl esterase: preparation and in-vitro analysis. Appl Microbiol Biotechnol. 2007;75:1023–1029.
- Martoni C, Bhathena J, Jones ML, et al. Investigation of microencapsulated BSH active lactobacillus in the simulated human GI tract. J Biomed Biotechnol. 2007;2007:13684
- Wang PR, Guo Q, Ippolito M, et al. High fat fed hamster, a unique animal model for treatment of diabetic dyslipidemia with peroxisome proliferator activated receptor alpha selective agonists. Eur J Pharmacol. 2001;427:285–293.
- Connolly BA, O’Connell DP, Lamon-Fava S, et al. The high-fat high-fructose hamster as an animal model for niacin's biological activities in humans. Metabolism. 2013;62:1840–1849.
- Pinkosky SL, Filippov S, Srivastava RA, et al. AMP-activated protein kinase and ATP-citrate lyase are two distinct molecular targets for ETC-1002, a novel small molecule regulator of lipid and carbohydrate metabolism. J Lipid Res. 2013;54:134–151.
- Rudel LL, Morris MD. Determination of cholesterol using o-phthalaldehyde. J Lipid Res. 1973;14:364–366.
- Zak B, Luz DA, Fisher M. Determination of serum cholesterol. Am J Med Technol. 1957;23:283–287.
- Warnick GR, Benderson J, Albers JJ. Dextran sulfate-Mg2+ precipitation procedure for quantitation of high-density-lipoprotein cholesterol. Clin Chem. 1982;28:1379–1388.
- Metabolic syndrome in adults, 2012 to 2013 2015. Available from: http://www.statcan.gc.ca/pub/82-625-x/2014001/article/14123-eng.htm#n1.
- Tanaka H, Doesburg K, Iwasaki T, et al. Screening of lactic acid bacteria for bile salt hydrolase activity. J Dairy Sci. 1999;82:2530–2535.
- Pithva S, Shekh S, Dave J, et al. Probiotic attributes of autochthonous Lactobacillus rhamnosus strains of human origin. Appl Biochem Biotechnol. 2014;173:259–277.
- Lambert JM, Bongers RS, de Vos WM, et al. Functional analysis of four bile salt hydrolase and penicillin acylase family members in Lactobacillus plantarum WCFS1. Appl Environ Microbiol. 2008;74:4719–4726.
- Kroon PA, Faulds CB, Ryden P, et al. Release of covalently bound ferulic acid from fiber in the human colon. J Agric Food Chem. 1997;45:661–667.
- Andreasen MF, Kroon PA, Williamson G, et al. Intestinal release and uptake of phenolic antioxidant diferulic acids. Free Radic Biol Med. 2001;31:304–314.
- Iiyama K, Lam T, Stone BA. Covalent cross-links in the cell wall. Plant Physiol. 1994;104:315–320.
- Hirabayashi T, Ochiai H, Sakai S, et al. Inhibitory effect of ferulic acid and isoferulic acid on murine interleukin-8 production in response to influenza virus infections in vitro and in vivo. Planta Med. 1995;61:221–226.
- Ahmad R, Thomas R, Kochumon S, et al. Increased adipose tissue expression of IL-18R and its ligand IL-18 associates with inflammation and insulin resistance in obesity. Immun Inflamm Dis. 2017;5(3):318–335
- Strand BL, Ryan TL, In't Veld P, et al. Poly-L-Lysine induces fibrosis on alginate microcapsules via the induction of cytokines. Cell Transplant. 2001;10:263–275.
- Creely SJ, McTernan PG, Kusminski CM, et al. Lipopolysaccharide activates an innate immune system response in human adipose tissue in obesity and type 2 diabetes. Am J Physiol Endocrinol Metab. 2007;292:E740–E747.
- Peng Z, Chen L, Xiao J, et al. Review of mechanisms of deoxynivalenol-induced anorexia: the role of gut microbiota. J Appl Toxicol. 2017;37(9):1021–1029
- Ley RE, Turnbaugh PJ, Klein S, et al. Microbial ecology: human gut microbes associated with obesity. Nature. 2006;444:1022–1023.