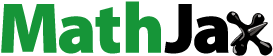
Abstract
Nanoparticles have many advantages such as high biocompatibility, bioavailability and effective therapeutic capabilities. The aim of the present study is to examine whether fluorescent magnetic submicronic polymer nanoparticles (FMSP-nanoparticles) have any impact on human colorectal cancer cells. In the present study, we have tested FMSP-nanoparticles with an average size of 100–200 nm on human colorectal carcinoma cells (HCT-116) to check their cytotoxic and anti-cancer capabilities. The effects of FMSP-nanoparticles on cancer cells were observed after 6 h, 24 h and 48 h intervals. The response of FMSP-nanoparticles-treated cells was evaluated by Trypan Blue, 4lue-diamidino-2-phenylindole (DAPI) and 3-(4,5-dimethylthiazol-2-yl)-2,5-diphenyltetrazolium bromide (MTT) assays. Our MTT analysis results revealed that FMSP-nanoparticles produced dose-dependent effects on cancer cells, FMSP-nanoparticles with dose of 1.25 µg/mL did not decrease cell survivability, whereas dosages of 12.5 µg/mL and 50 µg/mL respectively showed 23.59% and 59.47% decrease in the cancer cell survivability. In conclusion, our results demonstrate FMSP-nanoparticles have a potential anti-cancer capability and hold a great promise for colon cancer treatments.
Background
Approximately, 600,920 people were expected to die due to cancer in United States [Citation1,Citation3]. Colorectal or colon cancer is the 3rd most common type of cancer and the 4th most deadly cancer in the world [Citation2,Citation3]. The cancer is caused due to uncontrolled cell proliferation, and there are several methods through which cancer progress can be controlled and treated. These include, chemotherapy, radiation and surgery [Citation4]. Chemotherapeutic drugs are most widely used treatment to control the progression of cancer. One of the major challenges of the current chemotherapeutic drugs are that they have a poor recovery rate, for example, the recovery rate was found to be only about 57% [Citation5]. There is an urgent need of alternative approach to treat and control the cancer and over past few years, nanoparticles have generated tremendous interests in cancer treatments owing to their precise targeting, biocompatibility, bioavailability and multifunctional capabilities [Citation6–8].
In recent past, several attempts have been made to study the effect of different types of nanoparticles on cancer treatments. Most of the studies suggest that nanoparticles possess and exhibit anti-cancer properties [Citation4,Citation5,Citation9–18], also in being tested in colon cancer [Citation19]. Interestingly, all these nanoparticles have shown anti-cancer properties with mostly quantifiable manner, but still there is no report about the use of both qualitative and quantitative methods to evaluate the nanoparticles effect on cancer cells. In the present study, we have analysed the nanoparticles effects on cancer cells by using both morphometric analysis and MTT, Trypan blue and DAPI staining analysis. We have examined the effect of fluorescent magnetic submicronic polymer nanoparticles (FMSP-nanoparticles) on human colorectal carcinoma cell line (HCT-116). There are several reports of use of fluorescent magnetic nanoparticles in many biological and diagnostic applications [Citation20–26], but not much known about their anti-cancer properties especially in the colorectal cancers. In the present study, we have tested for the first time, the effect of FMSP-nanoparticles on HCT-116. We have used different concentrations of FMSP-nanoparticles and evaluated their cytotoxic effects by both morphometric and quantitative methods after 6 h, 24 h and 48 h post-treatments.
Methods
Synthesis and characterization of FMSP-nanoparticles
FMSP-nanoparticles were obtained from Systined Macromoloparticle et Physiopathologie Humaine (Lyon Cedex 07, France) and the method of synthesis and characterization has been described in detail in a paper published by (Citation27]. In brief, an organic ferrofluid which is composed of iron oxide nanoparticles was stabilized in octane which was surrounded by oleic acid. First deionized water was added to the anionic magnetic emulsion and the mixture was homogenized. After that, the supernatant was detached and the magnetic droplets were then added in deionized water. Then, deionized water was added and polyethyleneimine solution was added and after 15 min of continuous stirring, the magnetic droplets were washed with deionized water. The amount of polyethyleneimine adsorbed onto the magnetic droplets was construed by using specific amine titration. The obtained fluorescent magnetic nanoparticles were then quantified by using a fluorescence spectrophotometer (LS-50 System, Perkin Elmer, Waltham, MA). The structure and morphology of FMSP-nanoparticles were examined by scanning electron microscopy (SEM) (FEI, INSPECT S50, Brno, Czech Republic), and the size of fluorescent submicron magnetic nanoparticle was measured by transmission electron microscopy (TEM) (FEI, MORGAGNE.68, Brno, Czech Republic), respectively.
Treatment of FMSP-nanoparticles
Human colorectal carcinoma cell line with passage number 44 was obtained from Dr Khaldoon M. Alsamman, Clinical Laboratory Science, College of Applied Medical Science, Imam Abdulrahman Bin Faisal University (Dammam, Saudi Arabia). HCT-116 cells (2 × 105/cm2) were cultured in T25 flask containing the DMEM media with l-glutamine, 10% FBS, selenium chloride, 120 U/mL penicillin and 120 μg/mL streptomycin at 37 °C in 5% CO2 incubator (Heracell 150i, Thermo Scientific, Waltham, MA). The cells were then seeded into 96-well cell culture plates to be used for drug treatments. The cells with more than 80% confluence were used for fluorescent submicron magnetic nanoparticle treatment. Before treatments, fluorescent submicron magnetic nanoparticle was autoclaved for 30 min to remove the contamination from the sample. The temperature of magnetic nanoparticles was brought to room temperature before treatment. The cancer cells were treated to different concentrations of FMSP-nanoparticles (1.25 µg/mL, 12.5 µg/mL and 50 µg/mL) and cells were observed after 6 h, 24 h and 48 h intervals. For all the treatments, triplicate samples were taken for statistical calculations.
Cell morphology
At the end of each treatment (6 h, 24 h and 48 h), the cells from different concentrations of FMSP-nanoparticles were removed from the incubator and were observed under an inverted microscope (TS100F Eclipse, Nikon, Tokyo, Japan) equipped with a digital camera. Each sample was observed under 10X, 20Xeand 40Xemagnification to examine the anatomy and structure of the cells. The cell morphology of control samples and treated samples were observed and their images were recorded for the analysis.
Trypan blue staining
The viability of cancer cells was estimated by trypan blue exclusion test. Trypan blue staining was performed where nanoparticle-treated cells were first washed with phosphate-buffered saline (PBS), and then stained with Trypan blue (MPXX Biochemicals, Eschwege, Germany) prepared in a 0.4% solution in PBS. After 20 min, the samples were processed for manual counting using a haematocytometer under an inverted microscope (TS100F Eclipse, Nikon, Tokyo, Japan). The difference between live cells and dead cells (blue colour) were calculated for both control and FMSP-treated groups.
DAPI staining
The DAPI staining assay was carried out to examine the effect of nanoparticles on cell death and nuclear disintegration. Both control and treated cells were pre-treated with the freshly prepared ice-cold (4%) paraformaldehyde. The cells were treated with 0.1% Triton X-100 in PBS for 5 min for permeabilization of cell membranes. After that cells were stained with DAPI with concentration 1 μg/mL prepared in PBS for 5 min in the dark environment. Thereafter, the cells were washed with 0.1% Triton X-100 prepared in PBS. The cell morphology was analysed by confocal microscope (Zeiss, Oberkochen, Germany) equipped with digital camera.
MTT assay
The cells were first cultured in the media containing DMEM supplemented with 10% FBS and 1% penicillin (100 IU/mL) and streptomycin (100 μg/mL) in the CO2 incubator with 37 °C and 5% CO2 condition. Briefly, the cells were plated at a density of 8.5 × ty4 cells/well into 96 well plates and incubated in CO2 incubator. Upon reaching 80% confluency, the cells were treated with different concentrations of FMSPNs-207. In control group, FMSP-nanoparticles were not added. After 24 h treatment, 20 μg of MTT (5 mg/mL) was added to each well and incubated for 4 h. The media were changed with DMSO and each well was measured by using an ELISA plate reader (Synergy NEO2, Biotek Instruments, Winooski, VT) at 570 nm wavelength. Percentage (%) of cell viability (%) was calculated as per given formula:
Statistical analysis
All data were presented as mean ± standard deviation (SD) from triplicate experiments. Statistical analysis was performed using ANOVA followed by Dunnettddapost hoc test of GraphPad Prism software (GraphPad, La Jolla, CA). *p < .05, **p < .01 and ***p < .001 were considered statistically significant.
Results
Characterization of FMSP-nanoparticles
The structure of FMSP-nanoparticles was determined by both SEM and TEM analyses. The nanoparticles appeared to be crystallized, and spherical in shape (). TEM analysis revealed nanoparticles have an average diameter of 100–200 nm ().
Figure 1. The structure of FMSP-nanoparticles through scanning electron microscope (SEM) with 50,000 magnifications (a) and with 100,000 magnification (b). The structure of Fluorescent submicron magnetic nanoparticle through transmission electron microscope (TEM) with 70,000 magnifications (c) and with 180,000 magnification (d).
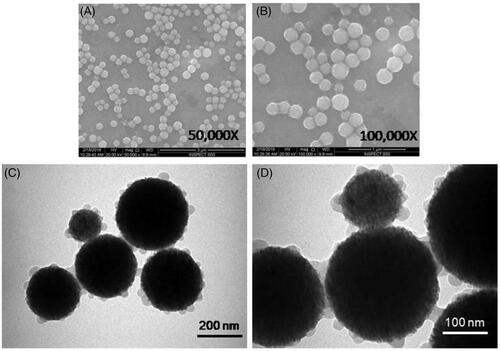
FMSP-nanoparticles severely impacted morphology of the HCT-116 cells
Treated cells were observed in 200Xrand 400Xrmagnifications to evaluate morphological changes in the nanoparticle-treated cells.
Treatment with 1.25 µg/mL: 6 h post-treatment, there was no cell death observed () as compared to control cells () and cell nucleus showed an early nucleus augmentation (). Twenty four hours post-treatment, no cell death was observed with an early indication of nuclear disintegration (). Forty eight hours post-treatment, no cell death was observed but there was significant nuclear disintegration observed in the cells ().
Treatment with 12.5 µg/mL: 6 h post-treatment, there was a significant level of nucleus augmentation and disintegration in the nanoparticles-treated cells () as compared to control cells (). Twenty four hours post-treatment, there was an increase in cell death and showed nuclear condensation and nuclear fragmentation. Also, few cells were found to be floated in the media (). Forty eight hours post-treatment, there was loss of cell population and loss of nuclear materials, few dead cells were found to be floated in the culture media ().
Treatment with 50 µg/mL: 6 h post-treatment, there was a strong indication of cell death observed () as compared to control cells (). The cells showed nuclear condensation and nuclear fragmentation (). After 24 h post-treatment, cell morphology changed with more cell death. Also, cells showed strong nuclear condensation and nuclear fragmentation (). After 48 h post-treatment, there was significant loss of cell numbers. We also observed many dead cells and their debris in the culture media ().
Cell viability by trypan blue staining
Trypan blue staining was done to determine the toxicity effects of FMSP-nanoparticles on cancer cells. Number of live cells were calculated in both control and FMSP-nanoparticles-treated groups. There was no impact on cell viability when cells were treated with 1.25 µ5/mL dose of nanoparticles (). When cancer cells were treated with 12.5 µg/mL, the cell viability was 88.83%, 83.83% and 76.41% after 6 h, 24 h and 48 h, respectively (). The treatment with 50 µ0/mL dose caused significant impact on cell viability, as cell viability was 58.13%, 51.59% and 40.53% after 6 h, 24 h and 48 h, respectively ().
Figure 5. Cell viability analysis by trypan blue staining. Viability of HCT-116 cell line was decreased on concentration-dependent manner in comparison with control group (*p < .05, **p < .01 and ***p < .001).
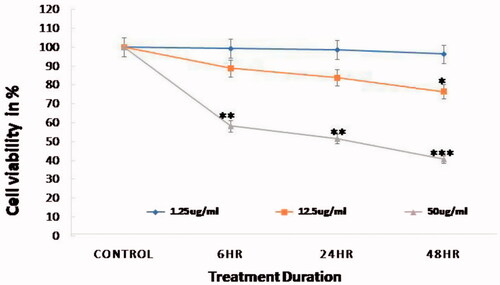
Cell viability by MTT assay
MTT assays were carried out to examine cell viability post FMSP-nanoparticle treatments. MTT assay was carried with different concentrations of FMSP-nanoparticles for 24 h. Treatment with 1.25 µ5/mL dose showed no impact on the cell viability, whereas treatment with 12.5 µ2/mL dose showed 83.30% cell viability. The 50 µ0/mL dose showed 51.49% cell survivability as compared to control cells ().
Figure 6. Cell viability analysis by MTT assay. The HCT-116 cells were treated with FMSP-nanoparticles (1.25 μg/mL, 12.50 μg/mL and 50 μg/mL) for 24 h and then subjected to the MTT staining assay. Data are the means ± SD of three different experiments. Difference between two treatment groups were analysed by Student’s t test where (*p < .05, **p < .01 and **p < .001), p values were calculated by Student’s t-test.
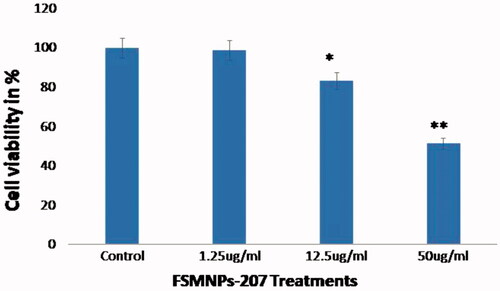
Nuclear condensation, fragmentation and disintegration by DAPI analysis
DAPI staining was done to evaluate effect of FMSP-nanoparticles on nuclear condensation, fragmentation and disintegration of cancer cells. DAPI staining was carried out with 1.25 µ5/mL, 12.5 µl/mL and 50 µ5/mL of FMSP-nanoparticles dosages for 48 h post-treatment. Treatment with 1.25 µ5/mL dose showed no nuclear disintegration () as compared to control cells (), whereas treatment with 12.5 µ2/mL showed significant amount of nuclear death (). The dose 50 µ0/mL showed highly significant amount of nuclear disintegration and cell death ().
Discussion
One of the biggest challenge of current cancer treatment modalities is that most of anti-cancer drugs are either less effective in controlling cancer progression or produce a significant level of side-effects. To solve the problem, studies have performed using nanoparticles to be able to better control cancer progression and development. In the present study, we have used HCT-116 cancer cells to evaluate anti-cancer capability of the FMSP-nanoparticles. Our findings showed that FMSP-nanoparticles can significantly decrease cancer cells viability as revealed by both morphological and quantitative analysis. Among all nanoparticles, magnetic nanoparticles have an edge over other nanoparticles owing to better biological activities and drug delivery. There is no information about the use of magnetic nanoparticles in cancer treatment as most of the magnetic nanoparticles have been either used in diagnostic or in drug delivery applications [Citation28–33]. This is the first study, where FMSP-nanoparticles were used in colorectal cancer cells. As we were specifically interested in the colorectal cancer, we have chosen HCT-116 to evaluate anti-cancer properties of FMSP-nanoparticles. HCT-116 cells have been extensively used as model for testing the anti-cancer drugs and molecules [Citation34–40].
In the present study, we have examined the cell morphology and structure under microscope. We have found that FMSP-nanoparticles with low dose (1.25 µ5/mL) produced no significant effect on the cell morphology, whereas the dosages 12.5 µ2/mL and 50 µ0/mL produced significant effects on HCT-116 cell morphology. The major morphological changes observed after 12.5 µ2/mL was chromatin condensation, and nuclear condensation. Strikingly, the dose with 50 µ0/mL showed a strong effect on both cell morphology and cell death. The cells showed high degree of chromatin condensation, nuclear condensation and nuclear fragmentation, and with strong presence of cellular debris of dead cells. There was lone study done which has shown morphology changes due to nanoparticle treatment [Citation41].
During our morphometric analysis, we found that FMSP-nanoparticles-treated cells undergo nuclear disintegration and fragmentation and to examine this phenomenon we have stained FMSP-nanoparticles-treated cells with DAPI. We have found that the cells treated with 1.25 µ5/mL dose showed no nuclear condensation, disintegration or fragmentation as compared to control group cells. The 12.5 µ2/mL and 50 µ0/mL dosages caused a significant nuclear condensation, fragmentation and disintegration. The nuclear fragmentation and disintegration is a characteristic of apoptosis [Citation42]. There are also several studies which have reported the use of DAPI as early indicator of apoptotic cell death [Citation42–45]. We do not know the mechanism of action of FMSP-nanoparticles in eliciting cell death, the possibility of apoptotic pathways cannot be ruled.
With a view to examine the impact of FMSP-nanoparticles on cancer cell viability, we have examined cell viability by both trypan blue and MTT analysis. Both trypan blue and MTT analysis showed that the FMSP-nanoparticles have dose-dependent effect on the cancer cells. The dose of 1.25 µ5/mL caused no impact on cell viability, whereas dosages of 12.5 µ2/mL and 50 µ0/mL caused significant decrease in cell viability. These results confirm that FMSP-nanoparticles have dose-dependent effects on the cancer cells. It has been previously observed that nanoparticles such as silver nanoparticles showed a strong inhibitory effect on the growth of lung tumour cells, human tongue squamous carcinoma, human breast cancer cells and chronic myeloid leukaemia cells [Citation46,Citation47].
During our study, we also measured an average size of the FMSP-nanoparticles to be able to understand how these nanoparticles produced cytotoxic effect on cancer cells. In our findings, the SEM and TEM analyses revealed that FMSP-nanoparticles with 100–200 nm size caused significant cell death of cancer cells. While we do not know the mechanism of interaction of FMSP-nanoparticles with cancer cells, the possibility of cell internalization cannot be ruled out. There are several reports of internalization of nanoparticles studied in various cancerous cells [Citation48–50] which led to cell death.
Conclusions
Both morphometric and quantitative analyses confirmed that FMSP-nanoparticles induced concentration-dependent effects on the cancer cells. The dose of 1.25 µ5/mL produced no significant effect cancer cell viability, whereas dosages of 12.5 µ2/mL and 50 µ0/mL significantly decreased the cancer cell viability. Interestingly, we have found that cells treated with FMSP-nanoparticles showed remarkable low DAPI staining in concentration-dependent manner. Finally, the success of cancer therapy depends on nanoparticles that not only have strong anti-cancer properties but also produce desirable therapeutic response on low concentration, and without the use of radiation, and magnetic field activation. The experimental results reported in this paper demonstrate that the FMSP-nanoparticles are potential biomaterials to be used in the treatment of colon cancer.
Availability of data and material
The data analysed are available from the corresponding author upon a request.
Abbreviations | ||
CO2 | = | Carbon dioxide |
DAPI | = | 4′,6-diamidino-2-phenylindole |
DMSO | = | Dimethyl sulphoxide |
DMEM | = | Dulbecco's modified Eagle medium |
ELISA | = | Enzyme-linked immunosorbent assay |
FBS | = | Foetal bovine serum |
IU | = | International Unit |
LNCap | = | Epithelial cell line derived from a human prostate carcinoma |
FMSP-nanoparticles | = | Fluorescent magnetic submicronic polymer nanoparticles |
MNPs | = | Magnetic nanoparticles |
MTT | = | 3-(4,5-Dimethylthiazol-2-yl)-2,5-diphenyltetrazolium bromide |
NPs | = | Nanoparticles |
OD | = | Optical density |
SEM | = | Scanning electron microscopy |
TEM | = | Transmission electron microscopy |
Acknowledgements
Authors are thankful to the entire management of the Institute for Research & Medical Consultations (IMRC), Imam Abdulrahman Bin Faisal University, Dammam, Kingdom of Saudi Arabia for their support and encouragement. We are thankful to Mr. Dionecio Jr. Bagon Dela Roca for assisting the cell culture and bioassay related work. We are also thankful to Dr. Khaldoon M. Alsamman, Clinical Laboratory Science, College of Applied Medical Science, Imam Abdulrahman Bin Faisal University, Dammam, Saudi Arabia for providing HCT-116 cell line.
Disclosure statement
The authors declare that they have no competing interests.
Additional information
Funding
References
- National Cancer Institute at National Institutes of Health (NIH). Cancer stat facts: ovarian cancer, 2016. Available from: https://seer.cancer.gov/statfacts/html/ovary.html
- Siegel R, Ma J, Zou Z, et al. Cancer statistics, 2014. CA Cancer J Clin. 2014;64:9–29.
- American Association for Cancer Research (AACR), Cancer Progress Report. 2017. Available from: https://www.aacrfoundation.org/Pages/cancer-progress-report.aspx
- Smalley KS, Herlyn M. Towards the targeted therapy of melanoma. Mini Rev Med Chem. 2006;6:387–393.
- Yaffee P, Osipov A, Tan C, et al. Review of systemic therapies for locally advanced and metastatic rectal cancer. J Gastrointest Oncol. 2015;6:185–200.
- Aftab S, Shah A, Nadhman A, et al. Nanomedicine: an effective tool in cancer therapy. Int J Pharm. 2018;540:132–149.
- Afrimzon E, Deutsch A, Shafran Y, et al. Intracellular esterase activity in living cells may distinguish between metastatic and tumor-free lymph nodes. Clin Exp Metastasis. 2008;25:213–224.
- Zhao J, Castranova V. Toxicology of nanomaterials used in nanomedicine. J Toxicol Environ Health B Crit Rev. 2011;14:593–632.
- Gottlieb E, Armour SM, Harris MH, et al. Mitochondrial membrane potential regulates matrix configuration and cytochrome c release during apoptosis. Cell Death Differ. 2003;10:709–717.
- Wood A, Schneider J, Shilatifard A. Cross-talking histones: implications for the regulation of gene expression and DNA repair. Biochem Cell Biol. 2005;83:460–467.
- Unfried K, Albrecht C, Klotz LO, et al. Cellular responses to nanoparticles: target structures and mechanisms. Nanotoxicology. 2007;1:52–71.
- Hanley C, Layne J, Punnoose A, et al. Preferential killing of cancer cells and activated human T cells using ZnO nanoparticles. Nanotechnology. 2008;19:295103.
- Turcotte S, Chan DA, Sutphin PD, et al. A molecule targeting VHL-deficient renal cell carcinoma that induces autophagy. Cancer Cell. 2008;14:90–102.
- Wang H, Wick RL, Xing B. Toxicity of nanoparticulate and bulk ZnO, Al2O3 and TiO2 to the nematode Caenorhabditis elegans. Environ Pollut. 2009;157:1171–1177.
- Rasmussen JW, Martinez E, Louka P, et al. Zinc oxide nanoparticles for selective destruction of tumor cells and potential for drug delivery applications. Expert Opin Drug Deliv. 2010;7:1063–1077.
- Mathew R, White E. Autophagy in tumorigenesis and energy metabolism: friend by day, foe by night. Curr Opin Genet Dev. 2011;21:113–119.
- Wang X, Wang W, Li L, et al. Oxidative stress and mitochondrial dysfunction in Alzheimer's disease. Biochim Biophys Acta. 2014;1842:1240–1247.
- Pati R, Das I, Mehta RK, et al. Zinc-oxide nanoparticles exhibit genotoxic, clastogenic, cytotoxic and actin depolymerization effects by inducing oxidative stress responses in macrophages and adult mice. Toxicol Sci. 2016;150:454–472.
- Jiang H, Shi X, Yu X, et al. Hyaluronidase enzyme-responsive targeted nanoparticles for effective delivery of 5-fluorouracil in colon cancer. Pharm Res. 2018;35:73.
- Bollhorst T, Shahabi S, Wörz K, et al. Chemical-induced apoptosis: formation of the Apaf-1 apoptosome. Drug Metab Rev. 2003;35:337–363.
- Kaewsaneha C, Bitar A, Tangboriboonrat P, et al. Fluorescent-magnetic Janus particles prepared via seed emulsion polymerization. J Colloid Interface Sci. 2014;424:98–103.
- Long G, Zhang Y, Yang X, et al. Facile characterization of the immobilization of streptavidin on magnetic submicron particles with a fluorescent probe of streptavidin. Appl Spectrosc. 2013;67:688–691.
- Grafton MM, Wang L, Vidi PA, et al. Breast on-a-chip: mimicry of the channeling system of the breast for development of theranostics. Integr Biol (Camb). 2011;3:451–459.
- Govindaiah P, Jung YJ, Lee JM, et al. Monodisperse and fluorescent poly(styrene-co-methacrylic acid-co-2-naphthyl methacrylate)/Fe3O4 composite particles. J Colloid Interface Sci. 2010;343:484–490.
- Huang H, Dong CY, Kwon HS, et al. Three-dimensional cellular deformation analysis with a two-photon magnetic manipulator workstation. Biophys J. 2002;82:2211–2223.
- Xu G, Yan Q, Lv X, et al. Imaging of colorectal cancers using activatable nanoprobes with second near-infrared window emission. Angew Chem Int Ed England. 2018;57(14):3626–3630. doi:10.1002/anie.201712528.
- Lansalot M, Sabor M, Elaissari A, et al. Elaboration of fluorescent and highly magnetic submicronic polymer particles via a stepwise heterocoagulation process. Colloid Polym Sci. 2005;283:1267–1277.
- Matthaiou EI, Barar J, Sandaltzopoulos R, et al. Shikonin-loaded antibody-armed nanoparticles for targeted therapy of ovarian cancer. Int J Nanomedicine. 2014;9:1855–1870.
- Barar J, Omidi Y. Surface modified multifunctional nanomedicines for simultaneous imaging and therapy of cancer. Bioimpacts. 2014;4:3–14.
- Heidari MM, Barar J, Asgari D, et al. Targeted fluoromagnetic nanoparticles for imaging of breast cancer mcf-7 cells. Adv Pharm Bull. 2013;3:189–195.
- Khosroushahi AY, Naderi-Manesh H, Yeganeh H, et al. Novel water-soluble polyurethane nanomicelles for cancer chemotherapy: physicochemical characterization and cellular activities. J Nanobiotechnol. 2012;10:2.
- Omidi Y. Smart multifunctional theranostics: simultaneous diagnosis and therapy of cancer. Bioimpacts. 2011;1:145–147.
- Moogooee M, Ramezanzadeh H, Jasoori S, et al. Synthesis and in vitro studies of cross-linked hydrogel nanoparticles containing amoxicillin. J Pharm Sci. 2011;100:1057–1066.
- Sudeep HV, Gouthamchandra K, Venkatesh BJ, et al. Viwithan, a standardized Withania somnifera root extract induces apoptosis in murine melanoma cells. Pharmacogn Mag. 2018;13:S801–S806.
- Mazewski C, Liang K, Gonzalez de Mejia E. Comparison of the effect of chemical composition of anthocyanin-rich plant extracts on colon cancer cell proliferation and their potential mechanism of action using in vitro, in silico, and biochemical assays. Food Chem. 2018;242:378–388.
- Matsuoka K, Kobunai T, Nukatsuka M, et al. Improved chemoradiation treatment using trifluridine in human colorectal cancer cells in vitro. Biochem Biophys Res Commun. 2017;494:249–255.
- Lin YY, Lee IY, Huang WS, et al. Danshen improves survival of patients with colon cancer and dihydroisotanshinone I inhibit the proliferation of colon cancer cells via apoptosis and skp2 signaling pathway. J Ethnopharmacol. 2017;209:305–316.
- Asif M, Shafaei A, Abdul Majid AS, et al. Mesua ferrea stem bark extract induces apoptosis and inhibits metastasis in human colorectal carcinoma HCT 116 cells, through modulation of multiple cell signalling pathways. Chin J Nat Med. 2017;15:505–514.
- Zeng M, Zhu L, Li L, et al. miR-378 suppresses the proliferation, migration and invasion of colon cancer cells by inhibiting SDAD1. Cell Mol Biol Lett. 2017;22:12.
- Sinha A, Banerjee K, Banerjee A, et al. Induction of apoptosis in human colorectal cancer cell line, HCT-116 by a vanadium-Schiff base complex. Biomed Pharmacother. 2017;92:509–518.
- Zielinska E, Zauszkiewicz-Pawlak A, Wojcik M, et al. Silver nanoparticles of different sizes induce a mixed type of programmed cell death in human pancreatic ductal adenocarcinoma. Oncotarget. 2018;9:4675–4697.
- Fan YX, Liang ZX, Liu QZ, et al. Cell penetrating peptide of sodium-iodide symporter effect on the I-131 radiotherapy on thyroid cancer. Exp Ther Med. 2017;13:989–994.
- Darfarin G, Salehi R, Alizadeh E, et al. The effect of SiO2/Au core-shell nanoparticles on breast cancer cell's radiotherapy. Artif Cells Nanomed Biotechnol. 2018;1–11. doi:10.1080/21691401.2018.1470526.
- Bai Aswathanarayan J, Rai Vittal R, Muddegowda U. Anticancer activity of metal nanoparticles and their peptide conjugates against human colon adenorectal carcinoma cells. Artif Cells Nanomed Biotechnol. 2018;46(7)1444–1451 doi:10.1080/21691401.2017.1373655.
- Jahanfar F, Hasani A, Shanebandi D, et al. Enhanced in vitro anti-tumor activity of 5-azacytidine by entrapment into solid lipid nanoparticles. Adv Pharm Bull. 2016;6:367–375.
- He Y, Du Z, Ma S, et al. Effects of green-synthesized silver nanoparticles on lung cancer cells in vitro and grown as xenograft tumors in vivo. Int J Nanomedicine. 2016;11:1879–1887.
- Locatelli E, Naddaka M, Uboldi C, et al. Targeted delivery of silver nanoparticles and alisertib: in vitro and in vivo synergistic effect against glioblastoma. Nanomedicine (London). 2014;9:839–849.
- Thomas TP, Huang B, Choi SK, et al. Polyvalent dendrimer-methotrexate as a folate receptor-targeted cancer therapeutic. Mol Pharm. 2012;9:2669–2676.
- Serpe L, Gallicchio M, Canaparo R, et al. Targeted treatment of folate receptor-positive platinum-resistant ovarian cancer and companion diagnostics, with specific focus on vintafolide and etarfolatide. Pharmacogenom Pers Med. 2014;7:31–42.
- Chen H, Zhang T, Zhou Z, et al. Enhanced uptake and cytotoxicity of folate-conjugated mitoxantrone-loaded micelles via receptor up-regulation by dexamethasone. Int J Pharm. 2013;448:142–149.