Abstract
Although hyaluronan (HA)-based biomaterials have been proposed to promote mucociliary differentiation of nasal epithelial cells (NECs), the mechanism by which HA affects the growth and differentiation of NECs has not been thoroughly explored. This study investigates the effect and mechanism of HA on the differentiation of NECs. The experiment cultures human NECs in four conditions, namely controls, transforming growth factor (TGF)-β1, TGF-β1 + HA and HA groups. In the TGF group, the NECs become irregular shape without formation of tight junction and mucociliary differentiation of NECs is inhibited. Epithelial–mesenchymal transition (EMT) of NECs also occurs in the TGF group. However, with addition of HA in TGF groups, NECs reveal the mucociliary phenotypes of epithelial cells with tight junction expression. Incubation of TGF-β1 in an NEC culture leads to an increase in phosphorylated type 1 TGF-β receptors (p-TβRI). This increase is attenuated when NECs are cultured in the presence of HA. Similar expressions are observed in phosphorylated smad2/smad3. Additionally, HA-dependent inhibition of TGF-β1 signalling is inhibited by co-incubation with a blocking antibody to CD44. Experimental results indicate that HA can antagonize TGF-β1 effect on EMT and mucociliary differentiation of NECs by down-regulation of TβR I, which is via CD44.
Introduction
The sinonasal epithelium, comprising ciliated epithelial cells, mucous blanket and mucus-producing glands, acts as an important defence mechanism against inhaled toxins, pathogens and particles. The development of chronic rhinosinusitis (CRS) has multiple causes, but its ultimate underlying pathophysiology is stasis of sinonasal secretions, which is due to ineffective sinonasal mucociliary function followed by subsequent inflammation [Citation1]. CRS involves persistent inflammation in the paranasal sinus cavities, and represents a set of disease processes that disrupt mucociliary flow and cause ostial obstruction [Citation2]. Chronic inflammation of sinonasal mucosa can cause nasal epithelial metaplasia, subepithelial fibrosis and bone remodelling, which leads to recurrence of CRS. Endoscopic sinus surgery is now the mainstay surgical treatment for CRS; however, over 15% of patients require a repeat operation wherein postoperative adhesion formation is a major cause of failure [Citation3]. Recent evidence indicates that transforming growth factor (TGF) β-1 is an important regulator involved in inducing epithelial-to-mesenchymal transition (EMT) and fibrosis in airway epithelial cells, especially in sinonasal wound healing process [Citation4,Citation5]. Preventing fibrosis, adhesion formation and restoring mucociliary mucosa are major determinants of the success of endoscopic sinus surgery. Therefore, several treatment modalities have been adopted to reduce adhesion formation and restore mucociliary epithelium following surgery [Citation6].
Hyaluronan (HA), one of the major components of the extracellular matrix, is an anionic, non-sulphated, glycosaminoglycan, and is characterized as non-immunogenic, biocompatible and bioadhesive. Hyaluronan acts as a structure element within extracellular matrix and as a signalling molecule contributing to cell proliferation, migration and differentiation through more than one cell surface receptor. Researchers have suggested that HA has a pivotal role in mucosal host defence by stimulating the ciliary beating of nasal epithelial cells (NECs), and increasing the clearance of foreign material [Citation7]. Recent clinical works have proposed several HA-based biomaterials to promote mucosal healing, reduce postoperative adhesion formation, prevent fibrosis and restore mucociliary epithelium of sinonasal mucosa [Citation8–10]. However, no research has yet thoroughly investigated how HA influences the growth and differentiation of NECs, particularly the relationship between HA and TGF-β1. This study investigates the effect of HA on TGF-β1-related differentiation of NECs.
Materials and methods
Isolation and culture of human NECs
Human NECs were obtained from nasal inferior turbinates during septomeatoplasty according to a protocol detailed previously [Citation8]. Briefly, specimens were digested with 0.5% pronase (type XIV protease, Sigma-Aldrich, St. Louis, MO) in Dulbecco’s modified Eagle’s medium and Ham’s nutrient F12 (DMEM/F12) supplemented with 1% penicillin/streptomycin for 16–20 h at 4 °C. The cell suspension was filtered through a 40 µm cell strainer to remove cell aggregates and debris. After centrifugation, the cells were suspended in DMEM/F12:bronchial epithelial growth medium (BEGM SingleQuots Kit, Clonetics Corp., San Diego, CA) (1:1) supplemented with antibiotics, and adjusted to 105 cells/ml. Then, 1.5 ml cell suspension was added to Transwell inserts with 2.5 ml of the medium deposited on the basolateral side. Cultures were maintained at 37 °C in an atmosphere of 5% carbon dioxide in air. Following confluence, air–liquid interface (ALI) was created by removing the apical medium and incubated with treatments only from the basolateral compartment. The experiment had four groups, which were the control, TGF, TGF plus hyaluronan (TGF + HA) and HA groups. The culture medium was changed every other day thereafter. The concentration of TGF-β1 (Peprotech, Rocky Hill, NJ) and HA (200 kDa, SciVision Biotech Inc., Kaohsiung, Taiwan) was respectively set at 5 ng/ml and 2 mg/ml, which were determined through morphological examination, trypan blue assay and reverse transcription-quantitation polymerase chain reaction (RT-qPCR). Additionally, blocking antibodies to CD44 (Bethyl, Montgomery, AL) with concentration of 5 μg/ml was added in to culture medium after ALI for elucidating the mechanism.
RT-qPCR
The total RNA was extracted from the cultured cells using the Trizol reagent (Invitrogen, Carlsbad, CA). The first-strand cDNA was synthesized with the protocol from SuperScript™ III Reverse Transcription Kits (Thermo Scientific, Waltham, MA). The cDNA was utilized as the template for the qPCR reaction of specific gene expression (Thermo Scientific, Waltham, MA). The primers: FOXJ1: forward: 5′-cccacctggcagaattcaatc-3′, reverse: 5′-tctcccgaggcactttgatg-3′; E-cadherin: forward: 5′-gaacgcattgccacatacac-3′, reverse: 5′-attcgggcttgttgtcattc-3′; glyceraldehyde 3-phosphate dehydrogenase (GAPDH): forward: 5′-actttggtatcgtggaaggact-3′, reverse: 5′-agggatgatgttctggagagc-3′. Finally, the gene expression levels were analysed and normalized to GAPDH for each cDNA sample. Relative quantity of gene expression was calculated with the expression levels.
Morphological examination and measurements of transepithelial electrical resistance (TER)
Living, untreated cultures were observed under an inverse phase contrast microscope (TS-100, Nikon, Tokyo, Japan). The cultures were rinsed, and fixed with 2.5% glutaraldehyde for 1 h. The specimens were then dehydrated in a graded ethanol series and dried with the critical point technique with liquid CO2. Finally, the samples were coated with gold to a thickness of 25 nm, and examined under a scanning electron microscope (SEM). The TER was measured on day 21 after confluence, using a Millicell electrical resistance system epithelial volt-ohmmeter (Millipore, Billerica, MA) as described by the manufacturer. For statistical analyses, the TER readings of the empty inserts were subtracted and normalized to day 0.
Immunocytochemistry
The Transwell inserts were fixed in 4% paraformaldehyde, and permeabilized with 0.1% triton X-100. Cells were incubated with anti-acetylated tubulin (Sigma-Aldrich, St. Louis, MO), anti-MUC5AC, anti-ZO-1 antibodies (Abcam, Cambridge, UK), anti-E-cadherin (Abcam, Cambridge, UK), anti-N-cadherin (Abcam, Cambridge, UK) and anti-vimentin (Abcam, Cambridge, UK) after blocking in 3% bovine serum albumin. Primary antibodies were then visualized by species-appropriate secondary antibody conjugated to Alexa 488 (Invitrogen, Carlsbad, CA). To counterstain nuclear and cytoskeletal markers, DAPI (Invitrogen, Carlsbad, CA) and rhodamine conjugated phalloidin (Molecular Probes, Carlsbad, CA) were utilized. Images were further taken with a confocal microscope (LSM510, Carl Zeiss, Jena, Germany).
Western blot analysis
The NECs cultured on day 21 after confluence were lysed using a radioimmunoprecipitation assay lysis buffer containing Complete Protease Inhibitor Cocktail Tablets and Phosstop Phosphatase inhibitor Cocktail Tablets (Roche Diagnostics, Indianapolis, IN) for 30 min on ice. The supernatant was collected and measured by Quant-iTTM Assay System (Invitrogen, Carlsbad, CA) after centrifugation. The protein samples were denatured, separated by sodium dodecyl sulphate-polyacrylamide gel electrophoresis and then transferred onto the Immobilon-P membrane (Millipore, Billerica, MA). The membranes were blocked in CISblocking buffer (CIS-Biotechnology, Taichung city, Taiwan) at room temperature for 60 s and then probed with anti-acetylated tubulin (Sigma-Aldrich, St. Louis, MO), anti-MUC5AC (Abcam, Cambridge, UK), anti-E-cadherin (Abcam, Cambridge, UK), anti-N-cadherin (Abcam, Cambridge, UK) and anti-vimentin (Abcam, Cambridge, UK), anti-phosphorylated type 1/II TGF-β receptors (p-TβR I/II) (Cell Signalling, Boston, MA), anti-CD44 (Bethyl) and anti-phosphorylated Smad 2/3 (p-Smad 2/3) antibodies (Cell Signalling, Boston, MA) at 4 °C overnight. After washing three times, the membranes were incubated with HRP-conjugated secondary antibodies for 1 h and visualized with an enhanced chemiluminescence reagent (ECL; Millipore, Billerica, MA).
Statistical analysis
Data were expressed as mean ± standard deviation (SD). Statistical significance was evaluated using repeated measures analysis of variance (ANOVA) followed by Tukey’s post hoc test with p < .05 being considered significant. This study was approved by an institutional review board and all patients gave informed consent.
Results
Dose–response relationship between hyaluronan and TGF-β1
After confluence, the apical medium was removed to create the ALI culture, which was an important factor in inducing differentiation of primary culture of NECs [Citation11]. We defined “day 0” as the day when NECs reached confluence and the ALI was created. First, to determine the inflammatory signalling with an optimal dosage of TGF-β1 in this in vitro system, the medium on the basolateral side was supplemented with different concentrations of TGF-β1 for 21 day on day 0 (). The morphology of NECs changed from polygonal to irregular, detached and even aggregative shape with incubation of TGF-β1 (). Particularly, 7.5 ng/ml TGF-β1 seriously caused aggregations and death cells (83.2% ± 1.5%), assessed with trypan blue assay. The cell viability at 5 ng/ml TGF-β1 was 45.6% ± 2.1% (). Further, the expressions of FOXJ1 and E-cadherin were respectively used to evaluate cilia differentiation and epithelial integrity under 5 ng/ml TGF-β1 plus various concentration of HA ranging from 0 mg/ml to 3 mg/ml. Compared with TGF-β1 alone, adding HA with more than 2 mg/ml concentration significantly increased the expressions of FOXJ1 and E-cadherin (,H)).
Figure 1. Dose–response relationship between hyaluronan and TGF-β1. The morphology of NECs changes from polygonal to irregular, detached and even aggregative shape with incubation of TGF-β1 (B–E). Scale bar =100 μm. Cell viability (F). RT-qPCR of FOXJ1 and E-cadherin under 5 ng/ml TGF-β1 plus various concentration of hyaluronan ranges from 0 mg/ml to 3 mg/ml (G, H). Results are expressed as the mean ± SD. Different letters (a, b) indicate a significant difference (p < .05), while the same letter indicates no significant difference (p > .05).
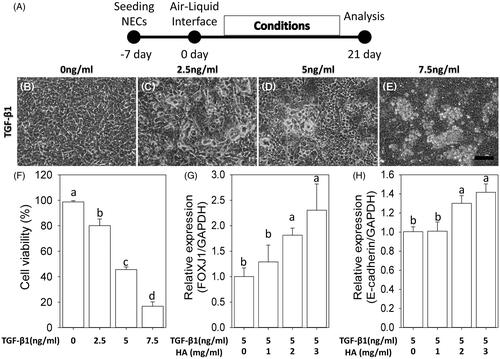
Evaluation of cellular morphology and epithelial integrity
On ALI day 21, SEM revealed a polygonal morphology with cilia differentiation in controls and HA groups, compared with an irregular morphology without cilia formation in the TGF group (). However, NECs revealed polygonal morphology with cilia differentiation with addition of HA in the TGF group (). The epithelial integrity of tight junction dynamics was further evaluated with TER. As shown in , TER value significantly fell in the TGF group (31.5 ± 0.8%), compared to 95.2 ± 14.3% in the TGF + HA group and 130.2 ± 22.9% in the HA group (p < .05).
Figure 2. Morphology of NECs treated with TGF-β1 (5 ng/ml), TGF-β1 plus hyaluronan (2 mg/ml) and hyaluronan (A–D). Scale bar = 100 μm. Scanning electron micrographs (magnification ×2000) (E–H). TER is measured and normalized to controls (I). Results are expressed as the mean ± SD. Different letters (a, b) indicate a significant difference (p<.05), while the same letter indicates no significant difference (p>.05). TER: transepithelial electrical resistance.
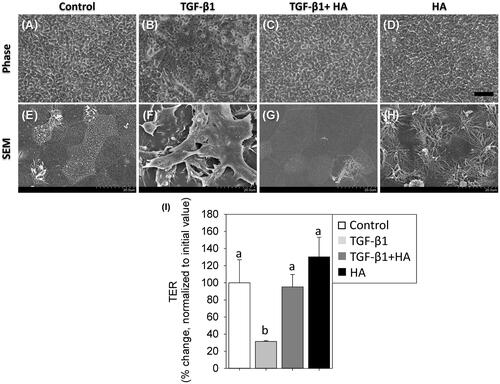
Immunocytochemistry of tight junction and epithelial–mesenchymal transition
Tight junctions were further evaluated with ZO-1 protein expression. The NECs in the TGF group did not show integrated tight junctions, and had less organized actin cytoskeletons than controls (). In contrast, these NECs developed tight junction formation with ZO-1 protein expression with addition of HA (). The development of EMT was evaluated by measuring the loss of E-cadherin as well as acquisition of N-cadherin and vimentin. Confocal images revealed NECs in the TGF group expressed scanty E-cadherin but abundant N-cadherin (). However, adding HA to the TGF group, the NECs increased the expression of E-cadherin and decreased N-cadherin expression compared to controls (). The expression level of vimentin had similar trends to N-cadherin (), indicating that HA can antagonize the effect of TGF-β1 on EMT.
Western blots analyses of tight junction and EMT
Western blots revealed adding HA significantly increase the expression of ZO-1. The expression level of ZO-1 was 0.47 ± 0.09 in TGF, compared with 0.97 ± 0.13 in controls, 0.82 ± 0.08 in TGF + HA and 0.96 ± 0.04 in HA groups (, p<.05). Western blots also confirmed the observation of EMT of NECs in confocal images. NECs in the TGF group have the smallest expression of E-cadherin (0.15 ± 0.02) among these groups (). In contrast, NECs expressed the highest level of N-cadherin and vimentin in the TGF group. However, the expression levels of N-cadherin and vimentin are decreased while adding HA into the TGF group, without significant differences from those in controls (,E)).
Mucociliary differentiation of NECs
Confocal images and SEM on day 21 after ALI showed that the NECs had scanty cilia in the TGF group, but had developed ciliated cells in the TGF + HA and HA groups (. Western blot analysis with the cilia maker, acetylated tubulin, further clarified this difference. The expression level of acetylated tubulin was the lowest (0.14 ± 0.06) in the TGF group, compared to 0.33 ± 0.09 in the TGF + HA group. The highest expression level of acetylated tubulin (0.44 ± 0.03) was in the HA group (). Mucus plays an important role in the defence mechanisms of the sinonasal system. Many mucin genes have been identified and sequenced to date. This study examined the major mucin, MUC5AC, expressed by airway epithelium [Citation12]. Confocal images of the NECs revealed mucous differentiation in controls in comparison with scanty mucus differentiation in the TGF group. However, NECs show mucous differentiation while adding HA in the TGF group (). Western blot analysis confirmed that MUC5AC expression was significantly lower in the TGF group compared with that in TGF + HA and HA groups ().
Figure 5. Observations of cilia using scanning electron micrographs (magnification ×15,000, scale bar = 3 μm) (A–D) and immunofluorescent confocal images of acetylated tubulin (E–H). Cellular cytoskeletal shape (F-actin) is outlined by rhodamine phalloidin. Nuclei are labelled with DAPI. Scale bar = 20 μm. Western blots and densitometric analyses of acetylated tubulin/GAPDH ratios are shown (I). Results are expressed as the mean ± SD and significant differences are indicated by different letters (p < .05).
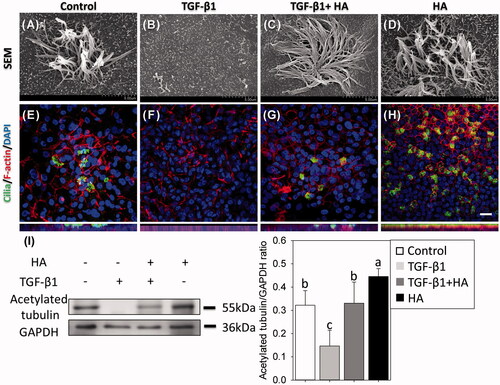
Figure 6. Mucin-secreting cells are virtualized using immunofluorescent confocal images of MUC5AC (A–D) and evaluated by western blots at day 21 after confluence. Cellular cytoskeletal shape (F-actin) is outlined by rhodamine phalloidin. Nuclei are labelled with DAPI. Scale bar = 20 μm. Densitometric analysis of MUC5AC/GAPDH ratios is shown (E, bottom). Results are expressed as the mean ± SD and significant differences are indicated by different letters (p < .05).
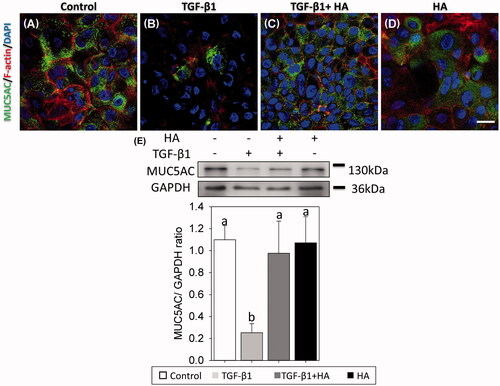
Alterations in phosphorylated TGF-β receptors and Smad of NECs
To understand the early molecular mechanism involved in the HA-regulated mucociliary differentiation of NECs, the activation of TGF-β receptors and Smad was examined by analysing the phosphorylation status of its molecule using a phospho-specific antibody. The expressions of phosphorylated type 1 TGF-β receptors (p-TβRI) and type 2 TGF-β receptors (p-TβRII) are significantly decreased in HA groups (). Incubation of TGF-β1 in NEC cultures led to a rise in p-TβRI (). This increase was attenuated when NECs cells were cultured in the presence of HA. Scanning densitometry revealed that the expression level of p-TβRI ratio was 1.35 ± 0.14 in TGF group, compared to 0.86 ± 0.13 in TGF + HA group (, p<.05). Conversely, the expression levels of p-TβRII were not significantly different between the TGF and TGF + HA groups (). Similar expressions were observed in phosphorylated smad2/smad3. Addition of TGF-β1 alone led to a significant increase in phosphorylated Smad2/3. In contrast, the expression of phosphorylated Smad2/3 was attenuated in the TGF + HA group (, p<.05).
Figure 7. Hyaluronan down-regulates phosphorylation of TβR1 and reduces TGF-β/Smad signalling of NECs. Phospho-TβR1, TβR1, phospho-TβR2, TβR2, phospho-Smad2, phospho-Smad3 and Smad2/3 expression are examined by western blot analyses (A). Densitometric analysis is shown (B–E). Results are expressed as the mean ± SD and significant differences are indicated by different letters (p < .05). TβR1: type 1 transforming growth factor receptor. TβRII: type 2 transforming growth factor receptor.
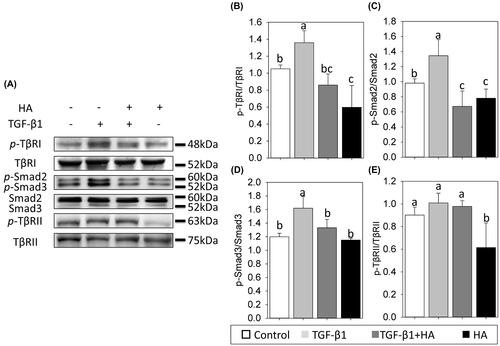
Effects of CD44 blocking antibodies (α-CD44) on the activation of TβRI
The relationship between HA and activation of TβRI was further evaluated with CD44 blocking antibodies. As shown in , incubation of TGF-β1 in NEC cultures led to a rise in p-TβRI. This increase was attenuated when NECs cells were cultured in the presence of HA. However, the expression level of p-TβRI re-increased with addition of CD44 blocking antibodies in the presence of HA and TGF-β1 (, p<.05). It indicated that blocking antibodies to CD44 prevented the antagonistic effect of HA on the activation of TβRI.
Figure 8. Hyaluronan-mediated down-regulation of phosphorylation of TβR1 is prevented with blocking antibodies to CD44. CD44, phospho-TβR1 and TβR1 expressions are examined by western blot analyses (A). Densitometric analysis is shown (B). Results are expressed as the mean ± SD and significant differences are indicated by asterisk (p< .05). α-CD44: blocking antibodies to CD44. TβR1: type 1 transforming growth factor receptor.
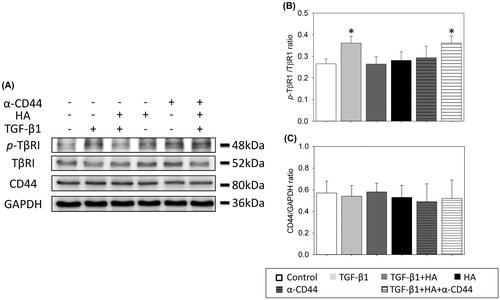
Discussion
TGF-β1 is a key cytokine involved in the pathogenesis of fibrosis in many organs and has been found in chronic wounds that heal with scar formation [Citation13]. Several cell types, including macrophages, fibroblasts and human airway epithelial cells, secrete TGF-β1 [Citation14,Citation15]. During the wound healing process, TGF-β1 regulates inflammatory cell activation [Citation16], enhances fibrosis formation [Citation17] and accelerates cell migration [Citation18]. However, in the respiratory system, TGF-β1 impedes mucociliary differentiation of respiratory epithelial cells in an autocrine or paracrine manner during the repair process [Citation19–21]. In order to restore the mucociliary function of airway after surgery or injury, attenuating the TGF-β1 effect is critical in the regeneration of respiratory epithelium. Hyaluronan appears to modulate the inflammatory response during healing process by altering TGF-β1 secretion and function. Additionally, HA has been demonstrated to promote mucociliary differentiation of NECs in vitro [Citation8,Citation10]. Hyaluronan, a highly hydrated polyanionic macromolecule, exists with molecular weights from 100k in serum to 8000k Da in the vitreous. The molecular size of HA plays an important role in its biological activity. Hyaluronan with low molecular weight less than 300 kDa stimulates cell migration, proliferation and differentiation. On the contrary, HA with high molecular weight more than 1000 kDa has no such effect [Citation22]. In the study, the HA is used as a biomaterial in the form of viscoelastic solution with MW of 200 kDa. Therefore, this study first evaluated the effects of TGF-β1 supplementation on the differentiation of NECs, and then investigated whether HA can antagonize the reaction of TGF-β1.
NECs become irregularly shaped without formation of tight junction during a culture period with incubation of TGF-β1 ( and ). This finding is compatible with a previous report that TGF-β1 reduced expression of ZO-1 protein and results in cellular morphology change [Citation23]. TER is a widely accepted quantitative method to measure the integrity of tight junction dynamics in cell culture models of endothelial and epithelial monolayers [Citation24]. The TER measurement further confirms these results (). Additionally, TGF-β1 promotes airway fibrosis by inducing EMT in epithelial cells, which is characterized by the change of the phenotypes from epithelial cells to mesenchymal cells [Citation8]. Fibrosis of sinonasal mucosa results in dysfunction of mucociliary epithelium, causing adverse effects such as mucus stagnation, graft constriction, desquamation and unpleasant odour [Citation25]. Loss of E-cadherin and acquisition of N-cadherin and vimentin are essential steps in EMT [Citation26]. Experimental results reveal that TGF-β1 induces EMT of NECs and HA can antagonize the effect of TGF-β1. NECs can express phenotypes of epithelial cells with a tight junction formation in the presence of TGF-β1 and hyaluronan.
The mucociliary differentiation of NECs also had similar results. The mucociliary system, including ciliated respiratory epithelium, mucous blanket and periciliary fluid, serves as a major defence mechanism in the respiratory system. Restoration of mucociliary function is important in sinonasal surgery as well as in respiratory tract reconstruction. During the cascade of wound healing, TGF-β1 is an important signal for the initiation of the healing cascade by attracting macrophages and stimulates them to secrete additional cytokines. TGF-β1 further improves fibroblast and smooth muscle cell chemotaxis and modulates collagen and collagenase expression. However, TGF-β1 inhibits mucociliary differentiation of NECs and concentrations of TGF-β1 as low as 1 pM inhibit the growth of primary cultures of respiratory epithelial cells, which accumulate in the G0-G1 phases of the cell cycle and tend to become squamous with formation of cross-linked envelopes [Citation27]. The results show that HA promote mucociliary differentiation of NECs, which is compatible with previous reports [Citation8]. Additionally, mucociliary differentiation of NECs is inhibited by TGF-β1, and HA prevents the TGF-β1 effect on the differentiation of NECs.
Researchers are still not clear whether HA interferes directly or indirectly with TGF-β signalling, despite several observations of the antagonistic activities of HA and TGF-β. TGF-β binds to transmembrane receptors with intrinsic serine/threonine kinase activity [Citation28]. The type II TGF-β receptor (TβRII) binds TGF-β, and then the type I TGF-β receptor (TβRI) is recruited into a heteromeric complex. TβRII transphosphorylates the glycine/serine-rich domain of TβRI kinase [Citation29]. Following the phosphorylation of Smad2 or Smad3 by the activated TβRI, a heteromeric complex is formed with Smad4, resulting in translocation of the complex into the nucleus [Citation30,Citation31]. CD44 is considered to be one of the major HA receptors [Citation32]. Interactions between HA and CD44 lead to ligand-induced clustering, and activation of intracellular signalling pathways [Citation33]. Koehler et al.’s study demonstrates that HA can modulate TGF-β1 receptor complex formation [Citation34]. CD44 interacts physically with TβRI and has a negative regulatory effect on the signalling of TβRI in dermal fibroblasts [Citation35]. In proximal renal tubular cells, HA regulates the TβR compartmentalization and HA-dependent inhibition of TGF-β1 signalling is inhibited by co-incubation with a blocking antibody to CD44 [Citation36]. The experimental results reveal that HA down-regulates the expression levels of TβRI and TβRII (). However, only the expression level of p-TβRI/TβRI decreases in the TGF + HA group. It indicates that HA down-regulates the expression of TβRI in the presence of TGF-β1, and then attenuates phosphorylated Smad2/3. Additionally, the expression level of p-TβRI re-increases with addition of CD44 blocking antibodies in the presence of HA and TGF-β1. Therefore, the HA-mediated down-regulation of TβRI in NECs is via CD44 (. Hyaluronan can antagonize the effect of TGF-β1 on EMT and mucociliary differentiation of NECs. To our knowledge, this is the first report to demonstrate that HA has antagonistic effects on TGF-β1 through down-regulation of TβRI in NECs.
Another benefit of applying HA in patients with CRS may be to lower the recurrence rate of CRS. Chronic inflammation in patients with CRS leads to structural changes, including various polyps, angiogenesis, goblet cell hyperplasia, epithelial shedding, subepithelial fibrosis and bone remodelling [Citation37]. TGF-β signalling pathway is up-regulated and activated during the bone remodelling process in CRS with nasal polyps. Therefore, due to the anti-TGF-β effect, HA not only improves mucociliary restoration of NECs, but also impedes epithelial fibrosis and persistent inflammation in bone tissue. However, whether our in vitro findings can be translated in vivo needs to be evaluated extensively in future work.
Conclusions
TGF-β1 induces EMT as well as inhibits mucociliary differentiation of NECs. Hyaluronan antagonizes the TGF-β1 effect through down-regulation of TβRI, which is via CD44. Analytical results of this study pave the way for potential applications of HA in mucociliary differentiation investigations and further treatment of sinonasal diseases.
Disclosure statement
No potential conflict of interest was reported by the authors.
Additional information
Funding
References
- Cohen NA. Sinonasal mucociliary clearance in health and disease. Ann Otol Rhinol Laryngol Suppl. 2006;196:20–26.
- Benninger MS, Ferguson BJ, Hadley JA, et al. Adult chronic rhinosinusitis: definitions, diagnosis, epidemiology, and pathophysiology. Otolaryngol Head Neck Surg. 2003;129:S1–S32.
- Ramadan HH. Surgical causes of failure in endoscopic sinus surgery. Laryngoscope. 1999;109:27–29.
- Van Bruaene N, C PN, Van Crombruggen K, et al. Inflammation and remodelling patterns in early stage chronic rhinosinusitis. Clin Exp Allergy. 2012;42:883–890.
- Yasukawa A, Hosoki K, Toda M, et al. Eosinophils promote epithelial to mesenchymal transition of bronchial epithelial cells. PLoS One. 2013;8:e64281.
- Fang KM, Wang CT, Chen YW, et al. Reduction of adhesions and antrostomy stenosis with topical vitamin A after endoscopic sinus surgery. Am J Rhinol Allergy. 2015;29:430–434.
- Forteza R, Lieb T, Aoki T, et al. Hyaluronan serves a novel role in airway mucosal host defense. FASEB J. 2001;15:2179–2186.
- Huang TW, Cheng PW, Chan YH, et al. Regulation of ciliary differentiation of human respiratory epithelial cells by the receptor for hyaluronan-mediated motility on hyaluronan-based biomaterials. Biomaterials. 2010;31:6701–6709.
- Huang TW, Chan YH, Cheng PW, et al. Increased mucociliary differentiation of human respiratory epithelial cells on hyaluronan-derivative membranes. Acta Biomater. 2010;6:1191–1199.
- Huang TW, Chan YH, Su HW, et al. Increased mucociliary differentiation and aquaporins formation of respiratory epithelial cells on retinoic acid-loaded hyaluronan-derivative membranes. Acta Biomater. 2013;9:6783–6789.
- Gruenert DC, Finkbeiner WE, Widdicombe JH. Culture and transformation of human airway epithelial cells. Am J Physiol. 1995;268:L347–L360.
- Thornton DJ, Gray T, Nettesheim P, et al. Characterization of mucins from cultured normal human tracheobronchial epithelial cells. Am J Physiol Lung Cell Mol Physiol. 2000;278:L1118–L1128.
- Border WA, Noble NA. Transforming growth factor beta in tissue fibrosis. N Engl J Med. 1994;331:1286–1292.
- Ueno H, Nakamura F, Murakami M, et al. Evaluation effects of chitosan for the extracellular matrix production by fibroblasts and the growth factors production by macrophages. Biomaterials. 2001;22:2125–2130.
- Wong T, McGrath JA, Navsaria H. The role of fibroblasts in tissue engineering and regeneration. Br J Dermatol. 2007;156:1149–1155.
- Letterio JJ, Roberts AB. Regulation of immune responses by TGF-beta. Annu Rev Immunol. 1998;16:137–161.
- Boxall C, Holgate ST, Davies DE. The contribution of transforming growth factor-beta and epidermal growth factor signalling to airway remodelling in chronic asthma. Eur Respir J. 2006;27:208–229.
- Howat WJ, Holgate ST, Lackie PM. TGF-beta isoform release and activation during in vitro bronchial epithelial wound repair. Am J Physiol Lung Cell Mol Physiol. 2002;282:L115–L123.
- Sacco O, Romberger D, Rizzino A, et al. Spontaneous production of transforming growth factor-beta 2 by primary cultures of bronchial epithelial cells. Effects on cell behavior in vitro. J Clin Invest. 1992;90:1379–1385.
- Lazard DS, Moore A, Hupertan V, et al. Muco-ciliary differentiation of nasal epithelial cells is decreased after wound healing in vitro. Allergy. 2009;64:1136–1143.
- Wu R, Zhao YH, Chang MM. Growth and differentiation of conducting airway epithelial cells in culture. Eur Respir J. 1997;10:23: 2398–2403.
- Huang L, Cheng YY, Koo PL, et al. The effect of hyaluronan on osteoblast proliferation and differentiation in rat calvarial-derived cell cultures. J Biomed Mater Res A. 2003;66:880–884.
- Willis BC, Liebler JM, Luby-Phelps K, et al. Induction of epithelial–mesenchymal transition in alveolar epithelial cells by transforming growth factor-beta1: potential role in idiopathic pulmonary fibrosis. Am J Pathol. 2005;166:1132–1321.
- Srinivasan B, Kolli AR, Esch MB, et al. TEER measurement techniques for in vitro barrier model systems. J Lab Autom. 2015;20:107–126.
- Toohill RJ. Autogenous graft reconstruction of the larynx and upper trachea. Otolaryngol Clin North Am. 1979;12:909–917.
- Willis BC, Borok Z. TGF-beta-induced EMT: mechanisms and implications for fibrotic lung disease. Am J Physiol Lung Cell Mol Physiol. 2007;293:L525–L534.
- Jetten AM, Shirley JE, Stone G. Regulation of proliferation and differentiation of respiratory tract epithelial cells by TGF beta. Exp Cell Res. 1986;167:539–549.
- Attisano L, Wrana JL. Signal transduction by members of the transforming growth factor-beta superfamily. Cytokine Growth Factor Rev. 1996;7:327–339.
- Wrana JL, Attisano L, Wieser R, et al. Mechanism of activation of the TGF-beta receptor. Nature. 1994;370:341–347.
- Heldin CH, Miyazono K, ten Dijke P. TGF-beta signalling from cell membrane to nucleus through SMAD proteins. Nature. 1997;390:465–471.
- Massagué J. TGF-beta signal transduction. Annu Rev Biochem. 1998;67:753–791.
- Banerji S, Ni J, Wang SX, et al. LYVE-1, a new homologue of the CD44 glycoprotein, is a lymph-specific receptor for hyaluronan. J Cell Biol. 1999;144:789–801.
- Jiang D, Liang J, Noble PW. Hyaluronan as an immune regulator in human diseases. Physiol Rev. 2011;91:221–264.
- Koehler L, Samsonov S, Rother S, et al. Sulfated hyaluronan derivatives modulate TGF-β1:receptor complex formation: possible consequences for TGF-β1 signaling. Sci Rep. 2017;7:1210.
- Porsch H, Mehić M, Olofsson B, et al. Platelet-derived growth factor β-receptor, transforming growth factor β type I receptor, and CD44 protein modulate each other's signaling and stability. J Biol Chem. 2014;289:19747–19757.
- Ito T, Williams JD, Fraser D, et al. Hyaluronan attenuates transforming growth factor-beta1-mediated signaling in renal proximal tubular epithelial cells. Am J Pathol. 2004;164:1979–1988.
- Wang M, Ye T, Liang N, et al. Differing roles for TGF-β/Smad signaling in osteitis in chronic rhinosinusitis with and without nasal polyps. Am J Rhinol Allergy. 2015;29:e152–e159.