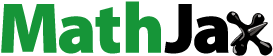
Abstract
Cancer is the largest family of diseases that involve abnormal uncontrolled cell growth which metastasizes to other parts of the body. The most common type of cancers includes lung, liver, colorectal, prostate, stomach, breast and cervical cancer with skin cancer excluding melanoma (contribute up to 40% of the cases). The conventional treatment approaches like surgery, chemotherapy, etc., have several side effects such as severe inflammation and pain. Hence, pharmacotherapeutic approaches of antineoplastic agents can be advantageous for treating various forms of cancer through the skin. Novel transdermal techniques and preparations have been emerged to overcome the limitations of skin and to penetrate inside the cancerous cells by transporting through the deeper tissues of the skin. The transdermal penetration of drugs using different formulations such as nanocarriers, physical penetration enhancement techniques, chemical penetration enhancers and newer technologies such as gels, dendrimers, needle-free injection jet etc., show improved patient compliance, abolition of scars and economic value. The topical delivery of antineoplastic agents is an attractive choice for increasing site-specific delivery, reducing side effects and improving therapeutic effects. The objective of this review is to present insights into pharmacotherapeutic techniques, which can be used for transdermal delivery of anticancer agents through skin due to its potential to create a new frontier in treatment of cancer.
Keywords:
Introduction
Cancer is the 2nd foremost cause of fatality internationally and accounts for 8 million deaths annually. The research suggested that variations in oncogenes, tumour suppressor and micro-RNA genes are the leading causes of cancer [Citation1]. Cancer is characterized by a full-grown tumour with an ability to invade and demolish the mesenchymal cells lying inside. It is capable of resisting cell death, supporting proliferative signaling, eluding growth suppressors and immunity, forming new blood vessels, activating invasion and metastatic growth [Citation2]. It was also found that malignancy involves organization of stroma of tumor, change in cell metabolism and neo-angiogenesis which further express tumour-associated antigens [Citation3]. Researchers have also found the principle cancerous and tumour suppressor genes, for induction or suppression to impart abnormal traits on other normal cells, contribute to their alteration into the cancerous form [Citation4]. Researchers and their contribution in cancer are summarized in . Most familiar types of cancers include cancer of lung, breast, skin, colorectal, prostate, ovarian cancers, squamous cell carcinoma and leukaemia. According to WHO, the world-wide death-rate in 2015 due to various forms of cancer is given in .
Table 1. Researchers and their finding in the field of cancer.
In case of breast cancer, 15% of women are diagnosed and is the foremost reason of death in women during their reproductive age. These cases occur intermittently due to unceasing exposure to several environmental cancer-causing agents. [Citation17] Unlike breast and ovarian cancer, skin cancer is not the life-threatening condition. They are classified based on the cell from which they arise such as squamous cell carcinomas, basal cell carcinomas and malignant melanomas. The most familiar type of skin cancer is ‘basal cell carcinoma’. However, malignant melanoma contributes to very less proportion of skin cancers, it accounts for maximum deaths caused due to skin cancers [Citation18].
The human herpesvirus-8 and immunosuppression are the main causes of Kaposi sarcoma (KS), which is present in different forms such as classic, AIDS-associated and iatrogenic. It is characterized by transitional, non-spreading lesions which have an impact mainly on the visceral organs, skin, lymph nodes and the mucosa [Citation19]. Leukaemia is caused due to the uninhibited rate of division of hematopoietic cells, changes in cancer-causing genes and tumour-suppressor genes. In case of acute myeloid leukaemia, which is a heterogeneous disorder, higher number of immature myeloid cells are produced in the bone marrow and interferes with loss of normal hematopoietic function [Citation20]. The leading causes of cancer of oropharynx are smoking and alcohol, which affect primarily the squamous cells of oropharyngeal mucosa [Citation13]. According to WHO, ‘tobacco use’ is amongst major risk factors for cancer and is contributing for about one-fourth of cancer deaths globally.
In topical drug delivery systems, transdermal penetration of antineoplastic agents can be advantageous for treating various forms of skin cancer, especially non-melanoma type of cancers. Although conventional treatment approaches such as surgery, chemotherapy, etc. are available, they may show several side effects such as severe inflammation and pain. Several adjuvant therapies such as immunotherapy, gene therapy and photodynamic therapy are also used to treat cancer. Nevertheless, transdermal delivery of drugs shows improved patient compliance, abolition of scars, and lower cost. In case of paediatric patients, transdermal delivery of antineoplastic drugs can be considered beneficial. However, few features of skin of infants and neonates such as delicate, resilient skin due to which they need lesser doses than adults. Therefore, only some drugs can be delivered transdermally for pediatric unlike adults. [Citation21] In case of children, researchers have found that transdermal patches show better drug delivery which can be effectively extrapolated for neonates and infants.
The topical delivery of anticancer agents is an attractive choice for increasing site-specific delivery, reducing side effects and improving therapeutic effects. The most important challenge of therapy is to augment the penetration of cytotoxic drug up to adequate levels to kill cancer cells. Many techniques and preparations have been invented to overcome limitations of skin and to penetrate inside cancerous cells by passing through the deep layers of skin. The penetration enhancers such as chemical and physical cause disruption of the skin reversibly and thus improve the penetration of drug into the lesions. Likewise, more research has been conducted on nanoparticulate delivery techniques that can guard antineoplastic agents against degradation and used with other physical methods, which can notably enhance the penetration of cancerous cells. The intent of this review is to present the various pharmacotherapeutic approaches for transportation of anticancer agents through skin which can be used to achieve therapeutic concentration of antineoplastics.
Structure of skin
The largest and the most heterogeneous organ of our body, skin is made up of three layers: epidermis, dermis and hypodermis. Epidermis, the outermost avascular layer made up of keratinocytes, plays a key role in delivering substances across the skin. () The differentiation of epidermis from inside to outside towards the outer surface of skin gives rise to stratum corneum which forms the prime barrier for the delivery of drugs due to its composition. The stratum corneum is made up of packed layer of corneocytes with the lipid matrix composed of cholesterol, free fatty acids and ceramides. These lipids are present in two co-existing lamellar phases: one is long (with repeat distance of 13 nm) and second is short (with constant distance of 6 nm). The space between two head and tail domains is less than 5 nm. The deep within the keratinocytes, several melanocytes (produce melanin) and Langerhans cells (antigen presenting cells) are embedded which are involved mainly in development of skin tumours [Citation22]. The exposure to environmental factors such as UV-rays causes tumour lesions ensuing into hyperkeratinization, which impedes the delivery of drug through the skin [Citation23]. To initiate the immune response to treat actinic keratosis, it is imperative to pass the anti-cancer or immunomodulator drugs through this hyperkeratotic stratum corneum, which further act on Langerhans cells to release pro-inflammatory mediators. Hence, it is necessary to optimize the topical formulations, which will penetrate through the deep layers of skin to reach the tumours.
The topical delivery of anti-cancer drugs
The penetration of drugs through skin takes place through three routes: intercellular (through stratum corneum), intracellular (through lipid matrix) and through skin appendages (sweat glands or hair follicles). The adequate log P values (between 1 and 3) and molecular weight (less than 600 Daltons) is necessary to pass the drug through stratum corneum [Citation24]. The diffusion of drug through skin follows Fick’s second law which is expressed as follows:
where J: Diffusion flux across the skin,Dm: Coefficient of diffusion of the drug through skin,Cv: Concentration of drug in the vehicle,P: Partition coefficient of drug and
L: Thickness of skin.
The hydrophilic nature, low log P values, ionic nature and high molecular weight emphasize the penetration of anti-cancer drug through skin is troublesome. Considering the factors governing the penetration of anti-cancer drug through skin, several approaches can be used for effective delivery like use of nanocarriers or nanoformulations, use of chemical enhancers or use of physical penetration enhancement techniques such as electroporation, iontophoresis, etc. to facilitate the transdermal anti-cancer drugs delivery [Citation25].
Strategies for topical anti-cancer drug delivery
The classification of different strategies used for anti-cancer treatment is shown in This following section discusses the different formulation techniques studied to treat cancer.
Nanocarrier systems
The topical formulation for effective cancer treatment should be a simple application which would suffice and allow the drug to penetrate into the deep layers of skin to reach the tumour site. This drug penetration is possible with the use of nanocarriers owing to their properties such as ability to reach deeply through the skin and provide site-targeting activity. However, it reduces the skin irritation by preventing the direct contact of potent anti-cancer drugs to skin. Moreover, they also improve the stability of drug [Citation26].
Polymeric nanoparticles and lipid nanocarriers
Nanoparticles are especially effective in case of anti-cancer drugs as it can improve the safety and efficacy of the drugs with dose reduction, thereby preventing unwanted harmful side-effects. Nanocapsules, nanospheres, nanostructured lipid carriers and solid-lipid nanoparticles are types of nanocarrier that are often used in the anti-cancer formulations. The drug from topical route penetrates the skin through an intercellular pathway. The polymerssuch as poly (dl-lactic acid), poly (lactic-co-glycolic acid) and poly-ε-caprolactone are mainly used in preparation of nanoparticles [Citation27]. These polymeric nanoparticles are gaining wide attention from scientists due to their enhanced permeation and retention effect at the site of action. This is important especially due to the multidrug-resistance (MDR) occurring in presence of p-glycoprotein on surface of tumour which eventually exudes the drug out. However, nanoparticles of size less than 5 nm can only pass through intercellular route. Hence, it is suggested that these nanoparticles larger in size penetrate through deep layers of skin through follicular route. In addition, skin damage caused in case of some pathological condition increases the penetration of the nanoparticles. Hence, it is controversial to state the capacity and route of penetration of intact nanoparticles across the stratum corneum.
Solid lipid nanoparticles (SLN) are used for sustained release of the anti-cancer drug due to its structural properties. Unlike nanoemulsions, in SLN, the lipid, which is solid in nature, is then dispersed into water to form nanoparticles [Citation28]. This imparts better stability, release profile and scalability to SLN as compared with any other lipid formulation. Studies suggest that they penetrate through junctions of corneocytes and get accumulated for a number of hours and then allow the release of anti-cancer agents in a sustained manner. Moreover, SLN also prevents the degradation of drug, thus allowing the topical application of anti-cancer drug [Citation29,Citation30]. However, issues of ideal mean diameter, surface charge, storage, stability and drug loading still limit their use in anti-cancer treatment. In nanocapsules, the drug is surrounded by shell whereas nanospheres are a matrix type of system in which the drug is either associated with the surface or entrapped inside the matrix uniformly. Various types of nanoparticles of cytotoxic drugs have been prepared such as 5-Fluorouracil (5-FU), 5-Aminolevulinic acid (5-ALA) and tretinoin. The in vitro release of the 5-Aminolevulinic acid microparticles showed temperature-based controlled release. Especially particles loaded with 10% w/w, ALA in propylene glycol gel produced the highest fluorescence levels in mice skin and reduced the tumour growth rate by 105%. However, this formulation improved the stability and penetration of the drug through stratum corneum. The same effect resulted in case of SLN of tretinoin. Moreover, the in vivo studies of 5-Aminolevulinic acid microparticles decrease in growth rate of skin tumour [Citation31]. The poly(dl-lactic acid) nanoparticles of prodrug 5-FU using nanoprecipitation method showed increased loading efficiencies as compared with the 5-FU and linear release for 6 h without initial burst release [Citation32]. In addition, for the topical nitric oxide release, the SLN of the nitrosyl-ruthenium complex was prepared using micro-emulsification technique, which showed better release profile in vitro without degrading the complex. These SLN protect the degradation of NO during in vitro which shows potential in carrying NO during topical delivery [Citation30]. The SLN of resveratrol also showed better cellular uptake. SLN can form depot of drug after penetrating through skin on topical application and thus prevents its penetration through skin. This was observed in in vitro studies of podophyllotoxin SLN in which drug was retained on skin surface. Garg and co-workers studied in in vitro studies that the transdermal flux of methotrexate is more when given in nano lipid carrier prepared using hot micro-emulsion method [Citation33]. Bromelain is a promising anti-tumour agent with proteolytic activity but requires higher dose at tumour site. Hence Bhatnagar validated the increased anti-cancer activity of bromelain nanoparticles as compared with its free form. These poly (lactic-co-glycolic acid) nanoparticles of bromelain showed better chemotherapeutic effects by changing the expression of P53, Bax and Bcl2 genes in mice. The number of tumours was reduced by 2.3 folds and volume of tumour was reduced by 2.5 folds. [Citation34] Researchers have demonstrated the topical iontophoretic delivery of nanoparticles of tyrosine kinase inhibitor-Imatinib. The functionalised gold nanoparticles of imatinib mesylate, layer-by-layer (LBL) coated with anionic poly (styrene sulfonate) and cationic (polyethyleneimine) polymers were prepared showing improved diffusion of imatinib through the skin.
Liposomes and ultra-deformable liposomes
Liposomes are widely used for topical anti-cancer treatment due to its biocompatibility with stratum corneum. These biodegradable colloid particles comprise a number of lipid bilayers. The conventional liposomes are prepared by dispersing phospholipid vesicular structures into aqueous media, which orient themselves to form lipid bilayers. This arrangement allows the entrapment of both hydrophilic and hydrophobic drugs inside vesicles. To improve the penetration of drugs through skin, the composition of conventional liposomes is altered. For example, in ultra-deformable liposomes, which are also known as elastic or ultra flexible liposomes, surfactants or amphiphiles or ethanol is added as edge activator to stabilize and impart stability to the liposomes. The first, second and third generations of flexible liposomes are known as transfersomes, niosomes and ethosomes respectively. In 1992, first-generation liposomes were introduced composing of sodium cholate and phosphatidylcholine. In ethosomes, sodium cholate is replaced by ethanol and in niosomes, non-ionic surfactants are used. The property of these vesicles to deform and pass through narrow gaps is responsible for their penetration. The in vivo studies conducted by Fang et al., 2008, suggested that liposomes and ethosomes increase the diffusion of 5-ALA and lead to persistent retention in skin layers on topical application [Citation35]. The anti-cancer activity of 5-FU-loaded niosomes showed 8 times increase transdermally as compared with its aqueous solution. However, instability and leakage during storage of liposomes limit its use in anti-cancer treatment. The elastic nature of methotrexate liposomes show higher transcutaneous flux as compared with methotrexate solution. The di-indolylmethane derivative 1,1-bis(3-indolyl)-1-(p-chlorophenyl) methane has possessed log P values more than 5 which hinders its entry through stratum corneum. Hence, ultra-flexible nanocarriers were prepared using ethanol-injection method, which allowed it to enter through skin pores owing to their flexibility. The in vitro studies of deformable liposomes of cytarabine were conducted on rat skin. This study showed increased transdermal flux and deposition in the skin layers in comparison with conventional liposomes. Ma et al. prepared the transethosomes of imiquimod (FDA approved drug used for managing basal cell carcinoma) showed slight better accumulation of drug in skin layers than control. Cosco and co-workers formulated ultra-flexible liposomes of two anti-cancer drugs (5-fluorouracil and resveratrol) for skin cancer treatment showed better transdermal delivery as compared with free drugs. Moreover, co-encapsulation of two antiproliferative drugs showed strong synergistic anti-tumour activity. These multidrug encapsulated liposomes showed wide application in the treatment of squamous cell carcinoma (SCC) [Citation36].
Microemulsion
Microemulsions are transparent, thermostable, isotropic mixtures of oil and surfactant frequently admixed with co-surfactant. This emulsion can be o/w or w/o or bi-continuous type with ability to solubilize weakly soluble drugs. In this system, saturated and unsaturated fatty acids form oil phase and are used as penetration enhancers. It can also be used as an efficient vehicle for solubilization of some drugs and protect them against degradation due to atmospheric condition. It provides sustained release of the active ingredient and prevents skin irritation irrespective of toxicity. However, it lacks few important features like dilution capability in water, stability and solubilization capacity. The topical preparation of curcumin is effective in the treatment of cancer due to its capacity to induce apoptosis by instigating cytotoxicity. However, its poor solubility and low bioavailability limit its transdermal delivery. Liu et al., studied the solubilisation effect of microemulsion on curcumin with different co-surfactants and limonene and eucalyptol as oil phase. In both cases, transcutaneous flux was found to be increased in comparison with control. The infiltration rate of curcumin was found be increased by 15.7-fold. Results show that microemulsion is a promising technique for the transdermal delivery of curcumin [Citation37].
Hydrogels
Hydrogels are prepared by functional cross-linking of hydrophilic monomers to form a network holding large amount of water inside. Various studies have been carried out for application of hydrogels in topical delivery of anti-cancer drugs. Collaud and colleagues prepared the hydrogel of hexylaminolevilunate (derivative of 5-aminolevulinic acid) using which photodynamic therapy which is given to patients for the treatment of cervical intraepithelial neoplasia. 5-ALA provides local delivery, but the limited stability of these formulations at 258 °C, the preparation of hydrogel treating neoplasia can be beneficial. As a result, thermosetting gel of poloxamer 407, which is in liquid state at room temperature, changes to semi-solid form while passing through female genital tract. This polymer can entrap the lipophilic drugs efficiently and deliver it to provide site-specific delivery [Citation38]. A compound obtained from Glycyrrhiza uralensis, isoliquiritigenin can retard the growth of prostate cancer. Kong and co-workers prepared a hydrogel of this drug using hyaluronic acid-hydroxyethylcellulose, which showed much higher penetration through mouse skin [Citation39]. Zheng prepared micelles of Pluronic F127 for honokiol, a hydrophobic drug obtained from Magnolia officinalis, which then loaded into thermosetting F127 hydrogel. It showed anti-cancer activity on variety of cancer cell lines. [Citation40]
Prodrugs
The prodrug concept of variety of drugs enhances the percutaneous delivery of drugs with poor partition coefficients. This technique involves adding of a pro-moiety to augment partition coefficient and therefore the solubility and carry the original drug through deep skin layers. After penetration in epidermis, enzymes release the drug by hydrolysis thereby improving the solubility in the epidermal layer of skin [Citation41]. Ara-C (primary treatment of acute myeloid leukaemia) is administered every day through i.v. route due to short half-life. It is quickly metabolized in plasma by deoxycytidine deaminase to inactive metabolite uracil arabinoside. Hence to overcome this problem, Lagoabe et al. prepared transdermal patch of Ara-C using its prodrug. The cytarabine alkyl amide derivative of Ara-C was prepared by acylation of amino group. This prodrug approach showed improvement in percutaneous penetration in in vitro studies. However, the second attempt of preparing the carbamate derivative of the same drug did not show any significant increase in percutaneous penetration. This might be due to its H-bonding of functional group with polar ends of lipid bilayers of skin [Citation42].
Peptide carriers
Transdermal delivery of small interfering RNA (siRNA) is a remarkable advancement for the treatment of cancer. But, due to difficulties like hydrophilic nature, negative charge and large peptides, it is impermeable to the deep skin layers. Some biological peptides can improve the delivery of macromolecular agents through stratum corneum. It facilitates the movement of them across by reversible opening of skin barrier. Researchers prepared a peptide carrier system to increase the penetration of siRNA across dermal layers, to support the target-specific delivery to malignant cells. Hence, the complex formation showed that SPACE-EGF increased the permeation across skin by the penetration of siRNA [Citation43].
The advantages and limitations of all above techniques have been mentioned in .
Table 2. Advantages and limitations of different techniques.
Physical permeation enhancement techniques
Iontophoresis
Iontophoresis has been used extensively to increase percutaneous penetration of a drug over the past few decades. It is a non-invasive physical permeation method, which includes the application of a low electric current that increases the penetration of drug through bilayer into the bloodstream. () This technique is used to improve the skin diffusion of ALA. For specific delivery, a steady low electrical current of less than or equal to 0.5 mA per sq.cm is applied using a solution of electrolyte drug. Two oppositely charged (anode and cathode) and insulated electrodes are employed to provide the current. On application of electric current, cations present in the solution move towards the negatively charged cathode, whereas anions from cathode move towards the positively charged anode. All ionized molecules of an anti-cancer drug are diffused in the solution of electrolyte which transports an electrical current, known as the transport number (with maximum transport number of 1). Hence, numerous factors associated to electrical properties of the structure require to be measured while improving the drug diffusion across the skin using iontophoresis, such as current intensity, electrode, ionic strength of electrolyte and pH. Electroneutrality of arrangement should not change the pH of electrolyte or change the properties of drug. The frequently used electrodes are reversible silver chloride electrodes as the reactions are fast and need lower voltage in comparison with water for electrolysis, thus avoid fluctuations of pH. When current is applied, chloride ions from solution react with the silver electrode, donating one electron to the circuit. This electron goes to the cathode, and reducing the silver chloride electrode [Citation44].
Figure 4. Assembly of iontophoresis demonstrating the mechanism of delivery anti-cancer drugs through skin.
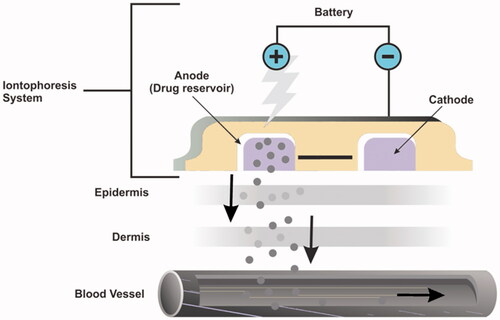
Skin is an alternative route for transporting anti-cancer drugs using two mechanisms: electromigration and electro-osmosis. These mechanisms work in amalgamation to increase drug skin penetration. Hence, positively charged anti-cancer agent, when immersed in the negatively charged compartment, they drift away from the electrode due to presence of similar charges into the skin. The skin penetration of drug depends on the amount and the electrical movement of the drug. High-molecular-weight drugs, including most anti-cancer drugs, have a low electric movement, which decrease the migration. In physiological conditions, electroosmosis takes place from the negative-to-positive charged electrode due to the skin’s permeability and selectivity for cations (twice as compared with anions). This is due to the negative charges on the skin formed when it comes in contact with a solution at physiological pH. This resulted due to unidirectional flow of solvent towards cation, thus improving the movement of positively charged particles and decelerating the movement of negatively charged particles. It is the main mechanism for skin permeability of drug as solvent flux pushes the molecules across the skin. Therefore, uncharged and bulky anti-cancer agents can utilise the solvent flow and pass through the skin by iontophoresis. The advantages of iontophoresis are the exact delivery of drug by adjusting electrical current. The density and the duration of current application, other formulation features, may quickly target the antineoplastic agents to the tumour in higher amounts. Taveira et al. confirmed that this technique considerably increases the permeability of doxorubicin. But, due to its positively charge at a body pH, it comes in contact with the negatively charged skin that decreases the distribution into the deep dermal layers. Nevertheless, researchers proved that the inclusion of chitosan (cationic polymer) decreases the negative charges of skin on application of electric current, thus delivering doxorubicin and enhancing the diffusion into skin [Citation45–47].
Electroporation
In this technique high-voltage pulses (100–1500 V) are applied () in cells or membranes to improve the drug diffusion. In this technique, events take place in the following order: (i) newer hydrophilic pathways are created in the cell membrane, (ii) molecules pass through them due to the local electric field and (iii) pores remain open for considerable time. On application, high-voltage pulses produce reversible pores in the skin by placing electrodes on skin surface. These pores increase the permeability of drugs, thus improving the penetration of macromolecules and hydrophilic drugs. Different types of electrodes can be used like needle-electrodes which are inserted into the tumour. This technique improves the topical delivery of various active agents such as metoprolol, fentanyl, insulin and piroxicam. The delivery of antineoplastic drugs, using electroporation, is known as electrochemotherapy. This technique involves the administration of the antineoplastic agent, mainly by i.v. route after which the high voltage is applied at site of action. It increases the penetration of drug through skin. Application of electroporation with other physical techniques such as iontophoresis provides synergistic activity in enhancing drug penetration through deep skin layers. When electroporation is applied alone, it enhances the permeation of the drugs as compared with iontophoresis as it creates new pathways through the stratum corneum, which increases the permeation and delivery of the anti-cancer drug across the skin. This technique has a good potential for transdermal antineoplastic drug delivery; however, the side effects and patient discomfort associated needs to be studied [Citation48].
Chemical penetration techniques
This technique has been widely used in transdermal formulations to enhance drug delivery of drugs by 1) reversibly disrupting the epidermis, 2) enhancing drug penetration across the skin and 3) altering the solubility in such a way that it will penetrate more in the skin. The disruption of lipid bilayer takes place by the technique explained in . It was found that Cinnamomum camphora (cinnamon) enhance the in vitro skin transcutaneous flux of 5-FU to considerable extent. Authors accredited the effect of permeation enhancing of molecule to change in structure of skin. Further studies confirmed that camphor changed the properly arranged molecules by altering the polarity of intercellular lipids. The chemical penetration enhancers usually increase permeation and retention into the skin. The most commonly used chemical enhancers for chemotherapy through skin are dimethyl sulfoxide (DMSO) and monoolein. The monoolein is less toxic and biodegradable compared with DMSO, which increases its use as a penetration enhancer. The commonly used chemical penetration enhancers and their characteristics are explained in . Recently researchers have developed a transdermal patch consisting of lipid-based transferosomes for painless delivery of drug [Citation49].
Newer technologies used for cancer treatment
LBL technique
LBL engineering holds a great potential to deliver nanocarriers used in topical treatment of various diseases due to its low cost and effectiveness. Nanogels show similar properties as that of tissues and to the extracellular matrix of the skin. However, they have the limits of pouring out the contents and also get aggregated. Hence to avoid these problems, nanogels are coated with many layers of polymers to attain the desired results. One such nanogel of folic acid was prepared using carboxymethyl cellulose and casein nanogels, and casein alone using LBL technique for treatment of skin cancer. This system can be used for the treatment of melanoma or other types of skin cancers in which curcumin reaches at the site of action providing effective treatment as compared with other formulations [Citation50].
Dendrimers
Dendrimers are spherical, hyper-branched 3 D (3-dimensional) macromolecules with nanoscale and have distinctive physical and chemical properties. The nano-size, lipophilic nature and globular shape facilitate their penetration through cell walls effortlessly, and thus suitable for topical drug delivery systems. ()
The patent by Hong proved that surface-engineered dendrimers can be used for topical delivery of cytotoxic agents in breast cancer treatment. The method was found to be effective and safe for the delivery of cytotoxic drugs. The cationic PAMAM dendrimers were prepared which showed almost 30% skin penetration by conjugating them with endoxifen due to strong dendrimer–phospholipid interactions and facilitated the delivery of endoxifen at much higher rate as compared with other dendrimeric formulations [Citation51].
Gel
Topical delivery to the breast can provide site-specific drug delivery with reduced systemic side effects. An in-situ clinical trial of 4-hydroxytamoxifen gel in women with ductal carcinoma showed that the analogous antiproliferative effect to breast skin; however, effects on parameters of endocrine and coagulation were reduced. These results support further evaluation of topical therapy for breast cancer prevention and treatment [Citation52].
Microneedle
Microneedles have created a new frontier in transdermal delivery of drugs as this tiny technique penetrates through skin up to 80–200 µm, avoiding the disruption of dermal layer. () This makes the delivery of drug to the patients painless, and thus show high patient compliance. Large molecules, such as anti-neoplastic, can be easily administered avoiding the first-pass metabolism. The administration using microneedles is easy as compared with hypo needle as it takes very less time to heal and there is no oedema or redness associated after injection. This helps in achieving the site-specific delivery of the drugs especially in case of anti-tumour agents where active agent can be delivered directly to the tumour area. Recently, researchers have developed nanoneedles of PVA, PLGA, etc. Nanoneedles can be used in developing nanoelectronics such as biosensors [Citation53]. The biodegradable microneedles of anti-PD-1 immunotherapy were prepared using hyaluronic acid and dextran nanoparticles, which encapsulated the anti-PD-1 and glucose oxidase enzyme. This enzyme converted the blood glucose into gluconic acid, thus creating acidic environment that promoted the dissociation of nanoparticles present inside and facilitated the release of anti-PD-1. The prepared system was found to induce the immune response. This combination with other immunomodulating agents can provide improved anti-tumour activity [Citation54].
Macroflux
In the past few years, antisense oligodeoxynucleotides have come up as a potential therapy for the treatment of a variety of diseases, especially cancer due to its effectiveness and lower toxicity. They specifically target genes and transcripts, which are involved in development of disease. However, they are prone to degradation in gastrointestinal tract and also poorly absorbed through bilayers. The parenteral route, which is commonly used for gene therapy, however is not compliant to patients. Hence, as a patient-friendly alternative, this transdermal delivery of oligodeoxynucleotides using macroflux technique can be explored [Citation55].
Needle-free jet injection
This new technique is employed for naked nucleic acid delivery for the treatment of cancer, as systemic delivery is limited due to the non-specific delivery due to destabilized blood circulation. In jet-injection technique, high air pressure is used that allows the site-specific targeted delivery of the drug. () Moreover, this technique is reliable and easy-to-use as it can be used over large area of skin without causing any irreversible or prolonged damage to the skin. ‘Chromosome 7 open reading frame 24’, is up-regulated in various forms of cancer and is generally linked with proliferation stage. The study conducted by Hama et al., the anti-tumour effect of anti-chromosome 7 open reading frame 24 small interfering RNA administered by needle-free jet injection for treatment of lung cancer in mice. This technique could successfully deliver anti-chromosome 7 open reading frame 24 siRNA to tumour, which inhibited the tumour growth by reducing ‘Chromosome 7 open reading frame 24’ levels [Citation56].
Conclusion
Conventional cancer treatment is a keystone to combat cancer, but several limitations are linked to the delivery of antineoplastic agents and their pharmacokinetics like resistance to anti-cancer drugs, toxicity issues and lack of selectivity. The random damage of healthy cells, the toxicity associated with chemotherapy and acquired resistance to them causes the need to look for effective therapies which will provide site-specific action. Newer pharmacotherapeutic approaches for transporting of antineoplastics through skin facilitate cancer treatments with reduced side effects and better pharmacokinetic profile as well. This review discusses the old and novice techniques which can be used for improving the transdermal delivery of cytotoxic agents in effective manner. Studies proved that these techniques hold a great potential and can thus create a new frontier to treat various forms of cancer. However, it is imperative not only to reach therapeutic window of these antineoplastic drugs but also to expand the horizons in combination of various techniques which can smoothen the progress of the transdermal delivery of the anti-cancer drugs.
References
- Croce CM. Oncogenes and cancer. N Engl J Med. 2008;358:502–511.
- Hanahan D, Weinberg RA. Hallmarks of cancer: the next generation. Cell 2011;144:646–674.
- Müller-Hermelink N, Braumüller H, Pichler B. TNFR1 signaling and IFN-gamma signaling determine whether T cells induce tumor dormancy or promote multistage carcinogenesis . Cancer Cell. 2008;13:507–518.
- Hanahan D, Coussens LM. Accessories to the crime: functions of cells recruited to the tumor microenvironment. Cancer Cell. 2012;21:309–322.
- Sibbesen NA, Kopp KL, Litvinov IV. Jak3: STAT3, and STAT5 inhibit expression of miR-22, a novel tumor suppressor microRNA, in cutaneous T-Cell lymphoma. Oncotarget 2015;6:20555–20569.
- Nicolaidou V, Koufaris C. MicroRNA responses to environmental liver carcinogens: biological and clinical significance. Clin Chim Acta. 2015;445:25–33.
- Yin WY, Lee MC, Lai NS. BK virus as a potential oncovirus for bladder cancer in a renal transplant patient. J Formos Med Assoc. 2015;114:373–374.
- Pluchino LA, Liu AKY, Wang HCR. Reactive oxygen species-mediated breast cell carcinogenesis enhanced by multiple carcinogens and intervened by dietary ergosterol and mimosine. Free Radic Biol Med. 2015;80:12–26.
- Labala S, Jose A, Venuganti VVK. Transcutaneous iontophoretic delivery of STAT3 siRNA using layer-by-layer chitosan coated gold nanoparticles to treat melanoma. Colloids Surf Biointerfaces. 2016;146:188–197.
- Walker S, Brew B. Kaposi sarcoma in a fingolimod-treated patient with multiple sclerosis. J Clin Neurosci. 2016;31:217–218.
- Shankar DB, Cheng JC, Kinjo K. The role of CREB as a proto-oncogene in hematopoiesis and in acute myeloid leukemia. Cancer Cell. 2005;7:351–362.[10.1016/j.ccr.2005.02.018]
- Kim H, Lee C, Yang JS. Structural modifications at the 6-position of thieno[2,3-d]pyrimidines and their effects on potency at FLT3 for treatment of acute myeloid leukemia. Eur J Med Chem. 2016;120:74–85.
- Beil CM, Keberle M. Oral and oropharyngeal tumors. Eur J Radiol. 2008;66:448–459.
- Adegboyega PA, Rodriguez S, McLarty J. Stromal expression of actin is a marker of aggressiveness in basal cell carcinoma. Hum Pathol. 2010;41:1128–1137.
- Lucena SR, Salazar N, Gracia-Cazana T. Combined treatments with photodynamic therapy for non-Melanoma skin cancer. Int J Mol Sci. 2015;16:25912–25933.
- Hodi FS, Corless CL, Giobbie-Hurder A. Imatinib for melanomas harboring mutationally activated or amplified KIT arising on mucosal: acral, and chronically sun-damaged skin. Jco. 2013;31:3182–3190.
- Li C, Tang C, Hu Z. Synthesis and structure–activity relationships of novel hybrid ferrocenyl compounds based on a bicyclic core skeleton for breast cancer therapy. Bioorg Med Chem. 2016;24:3062–3074.
- Simões MCF, Sousa JJS, Pais AACC. Skin cancer and new treatment perspectives: a review. Cancer Lett. 2015;357:8–42.
- Hasby EA, Mashad NE, Eltatawy R. C-Kit, CD34 & a-SMA immunohistochemical features in classic kaposi sarcoma and kaposiform hemangioendothelioma. J Microsc Ultrastruct. 2017;5:49–57.
- Crowell HL, MacLean AL, Stumpf MPH. Feedback mechanisms control coexistence in a stem cell model of acute myeloid leukaemia. J Theor Biol. 2016;401:43–53.
- Taveira SF, Lopez RFV. Topical administration of anticancer drugs for skin cancer treatment. In Skin Cancers-Risk Factors, Prevention and Therapy. IntechOpen, Croatia: Skin Cancers Caterina La Porta; 2011.
- McGrath JA, Eady RAJ, Pope FM. Anatomy and organization of human skin. Rook’s Textbook of Dermatology. United States: Blackwell Science Ltd; 2004. p.1–15.
- VA, Neel AJ. Sober. Other Skin Cancers. In: Donald W. Kufe, Raphael E. Pollock, Ralph R, et al., editors. Cancer Medicine Volume 7.Colombia:DC Decker; 2006. p.1663–1674.
- Schäfer-Korting MA, Wolfgang MB, Korting H. Lipid nanoparticles for improved topical application of drugs for skin diseases. Adv Drug Deliv Rev. 2007;59:427–443.
- Williams AC, Barry BW. Penetration enhancers. Adv Drug Deliv Rev. 2004;56:603–618.
- Schmid M-H, Korting HC. Therapeutic progress with topical liposome drugs for skin disease. Adv Drug Deliv Rev. 1996;18:335–342.
- Rancan F, Papakostas D, Hadam S. Investigation of polylactic acid (PLA) nanoparticles as drug delivery systems for local dermatotherapy. Pharm Res. 2009;26:2027–2036.
- Gratieri T, Gelfuso GM, Lopez RFV. Current efforts and the potential of nanomedicine in treating fungal keratitis. Expert Rev Ophthalmol. 2010;5:365–384.
- Teixeira Z, Zanchetta B, Melo BA, et al. Retinyl palmitateflexible polymeric nanocapsules: characterization and permeation studies. Colloids Surf B: Biointerfaces. 2010;81:374–380.
- Marquele-Oliveira F, Santana DCA, Taveira SF. Development of nitrosyl ruthenium complexloaded lipid carriers for topical administration: improvement in skin stability and in nitric oxide release by visible light irradiation. J Pharm Biomed Anal. 2010;53:843–851.
- Donnelly RF, McCarron P, Al-Kassas R, et al. Influence of formulation factors on PpIX production and photodynamic action of novel ALA-loaded microparticles. Biopharm Drug Dispos. 2009;30:55–70.
- McCarron PA, Hall M. Incorporation of novel 1-alkylcarbonyloxymethyl prodrugs of 5-fluorouracil into poly (lactide-co-glycolide) nanoparticles. Int J Pharm. 2008;348:115–124.
- Garg NK, Tyagi RK, Singh B. Nanostructured lipid carrier mediates effective delivery of methotrexate to induce apoptosis of rheumatoid arthritis via NF-kB and FOXO1. Int. J. Pharm 2016;499:301–320.
- Bhatnagar P, Pant AB, Shukla Y. Bromelain nanoparticles protect against 7,12-dimethylbenz[a]anthracene induced skin carcinogenesis in mouse model. Eur J Pharm Biopharm. 2015;91:35–46.
- Pierre MBR, Ricci E, Tedesco AC. Oleic acid as optimizer of the skin delivery of 5-aminolevulinic acid in photodynamic therapy. Pharm Res 2006;23:360–366.
- Paolino D, Cosco D, Muzzalupo R. Innovative bola-surfactant niosomes as topical delivery systems of 5-fluorouracil for the treatment of skin cancer. Int J Pharm. 2008;353:233–242. 2008),
- Liu CH, Chang FY. Development and characterization of eucalyptol microemulsions for topic delivery of curcumin. Chem Pharm Bull. 2011;59:172–178.
- Collaud S, Peng Q, Gurny R, et al. Thermosetting gel for the delivery of 5-aminolevulinic acid esters to the cervix. J Pharm Sci. 2008;97:2680–2690.
- Kong BJ, Kim A, Park SN. Properties and in vitro drug release of hyaluronic acid-hydroxyethyl cellulose hydrogels for transdermal delivery of isoliquiritigenin. Carbohydr Polym. 2016;147:473–481.
- Zheng X, Wang X, Gou M. A novel transdermal honokiol formulation based on Pluronic F127 copolymer. Drug Deliv. 2010;17:138–144.
- Benson HA. Transdermal drug delivery: penetration enhancement techniques. Cdd. 2005;2:23–33..
- Momparler RL. Optimization of cytarabine (ARA-C) therapy for acute myeloid leukemia. Exp Hematol Oncol. 2013;2:20.
- Ruan R, Chen M, Sun S. Topical and targeted delivery of siRNAs to melanoma cells using a fusion peptide carrier. Sci Rep. 2016;6:29159.
- Gratieri T, Gelfuso GM, Lopez RFV. Basic principles and applications of iontophoresis for cutaneous penetration of drugs. Quím Nova. 2008;31:1490–1498.
- Gelfuso GM, Gelfuso Gratieri T, Souza JG, Thomazine. The influence of positive or negative charges in the passive and iontophoretic skin penetration of porphyrins used in photodynamic therapy. Eur J Pharm Biopharm. 2011;77:249–256.
- Charoo NA, Rahman Z, Repka MA. Electroporation: An avenue for transdermal drug delivery. Curr Drug Deliv. 2010;7:125–136.
- Murthy SN, Murthy Zhao Y–L, Sen A, Hui. Cyclodextrin enhanced transdermal delivery of piroxicam and carboxyfluorescein by electroporation. J Control Release. 2004;99:393–402.
- K Moser Kriwet K, Naik A, Kalia. Passive skin penetration enhancement and its quantification in vitro. Eur J Pharm Biopharm. 2001;52:103–112.
- Shende PK, Bakal RL, Gaud RS. Modulation of serratiopeptidase transdermal patch by lipid-based transfersomes. J Adhes Sci Technol. 2015;29:2622–2633.
- Priya P, Raj RM, Vasanthakumar V. Curcumin-loaded layer-by-layer folic acid and casein coated carboxymethyl cellulose/casein nanogels for treatment of skin cancer. Arab J Chem. 2017;22. Available from: https://doi.org/10.1016/j.arabjc.2017.07.010
- Yang J, Hu J, He B. Transdermal delivery of therapeutic agents using dendrimers (US20140018435A1): a patent evaluation. Expert Opin Ther Pat.2015;325:1209–1214.
- Rouanet P, Linares-Cruz G, Dravet F. Neoadjuvant percutaneous 4-hydroxytamoxifen decreases breast tumoral cell proliferation: a prospective controlled randomized study comparing three doses of 4-hydroxytamoxifen gel to oral tamoxifen. JCO. 2005;123:2980–2987.
- Shende P, Sardesai M, Gaud RS. Micro to nanoneedles: a trend of modernized transepidermal drug delivery system. Artif Cells Nanomed Biotechnol. 2018;46:19–25.
- Wang C, Ye Y, Hochu GM, Sadeghifar H, et al. Enhanced cancer immunotherapy by microneedle patch-assisted delivery of anti-PD1 antibody. Nano Lett. 2016;2416:2334–2340.
- Lin W, Cormier M, Samiee A. Transdermal delivery of antisense oligonucleotides with microprojection patch (Macroflux®) technology. Pharm res. 2001;18:1789–1793.
- Hama S, Arata M, Nakamura I. Prevention of tumor growth by needle-free jet injection of anti-C7orf24 siRNA. Cancer Gene Ther. 2012;19:553
- Singh BN, Singh RB, Singh J. Effects of ionization and penetration enhancers on the transdermal delivery of 5-fluorouracil through excised human stratum corneum. Int J Pharm. 2005;298:98.
- Herai H, Gratieri T, Thomazine JA, Bentley, et al. Doxorubicin skin penetration from monoolein-containing propylene glycol formulations. Int J Pharm. 2007;329:88–93.
- Manconi M, Sinico C, Caddeo C, Vila AO, et al. Penetration enhancer containing vesicles as carriers for dermal delivery of tretinoin. Int J Pharm. 2011;412:37–46.