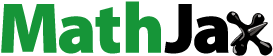
Abstract
Selective accumulation of photosensitizers (PSs) into cancerous cells is one of the most important factors affecting photodynamic therapy (PDT) efficacy. 5-Aminolevulinic acid (5-ALA) is precursor of a strong PS, protoporphyrin-IX (Pp-IX); but it has poor permeability in lipophilic membrane of the cells due to its hydrophilic property. Therefore, establishment of an improved delivery strategy could highly affect on treatment outcome. Moreover, folate receptors (FRs) are overexpressed on the surface of many tumor cells. In the present work, targeting ligand folic acid (FA) and 5-ALA conjugated bismuth oxide nanoparticles (FA-5ALA-Bi2O3 NPs) were synthesized; and used in PDT against human nasopharyngeal carcinoma cells (KB cell line). The KB cells incubated with the synthesized NPs for 2 h; then illuminated using a custom-made red light LED lamp at the light dose of 26 J/cm2. MTT and caspase-3 activity assays were performed to evaluate the efficacy of treatment. Results showed that FR targeting ligand enables selective endocytosis of FA-5-ALA-conjugated NPs into KB cells. Improved internalization of 5-ALA into cells decreased the cell viability to about 50%, 65%, and 85% in the groups receiving FA-5ALA-Bi2O3 NPs, 5ALA-Bi2O3 NPs, free 5-ALA and subsequent PDT, respectively. Therefore, FA-5ALA-Bi2O3 NPs can significantly increase the cell killing effect of PDT.
Introduction
Photodynamic therapy (PDT) is a noninvasive therapeutic modality that recently has drawn great attention for managing both non-oncological disorders and neoplastic diseases including cancer [Citation1,Citation2]. This treatment is performed using a light-sensitive agent, known as photosensitizer (PS), selective uptake of PSs by tumor cells, followed by illumination of the tumor region using a localized energy source with a suitable wavelength to activate PSs [Citation3]. The PS molecules then become excited and by transferring the absorbed energy to molecular oxygen, lead to the production of reactive oxygen species (ROS) [Citation4]. This process eventually causes cellular oxidation and subsequent death of the tumoral cells [Citation5].
PDT possesses great advantages such as facile application, selective targeting and besides, it is not a drug resistance treatment approach [Citation6]. However, it is barely been utilized due to the limited intracellular accumulation of PSs, low penetration depth of these substances and visible light into the target [Citation5]. Therefore, various studies have been performed to find more effective photosensitizing agents [Citation6,Citation7]. First-generation PSs induce cutaneous photo-toxicity; but second-generation PSs possess high quantum yield of singlet oxygen generation, deeper penetration into tissue and reduced skin photosensitivity [Citation8]. One such a molecule is 5-aminolevulinic acid (5-ALA) which is not a PS itself; but it accumulates in the cell mitochondrion [Citation9] and during the heme biosynthesis pathway, it turns into protoporphyrin-IX (Pp-IX) that actually is a strong PS [Citation5]. The Pp-IX can preferentially accumulate in metabolically active cancerous cellsCitation10. 5-ALA is a non-toxic material and also its excretion from biological systems is very fast; therefore, it has attracted significant attention to be used in PDT. However, its application in PDT because of its hydrophilic property, low specificity to cancer cells and thus poor cell permeability is hindered [Citation10,Citation11]. Therefore, using an appropriate delivery vehicle that might help 5-ALA selectively enter into cancer cells can increase the efficacy of 5-ALA mediated PDT. For this purpose, several studies have been carried out to make a suitable delivery system for transferring 5-ALA to tumor cells using metallic NPs. This is of great interest since these NPs can enhance singlet oxygen generation yield of PSs [Citation11,Citation12]. Moreover, NP-based drug delivery systems enable tumor selectivity and reduce toxicity to the normal tissues by means of the enhanced permeability and retention (EPR) effect [Citation11,Citation13,Citation14].
Bismuth NPs have recently drawn great attention for application in medical researches including radiotherapy [Citation15,Citation16]. It is related to the fact that bismuth is one of the least toxic metals with high atomic number (Z = 83) through which it can act as a radiosensitizer [Citation17]. In addition, bismuth compounds could be utilized in PDT because of their high photocatalytic performance [Citation1]. Moreover, bismuth does not accumulate in the body and therefore it is more biocompatible in biological systems [Citation16,Citation18].
High-affinity folate receptors (FR) are overexpressed on the surface of different tumor cells [Citation19,Citation20]. The presence of folic acid (FA) in NPs increases their ability to selectively target tumor tissues through detecting FR [Citation21,Citation22]. Thereby, receptor-mediated endocytosis takes place in which selective delivery of the photosensitizer to the cancerous cell could be acquired during PDT [Citation21].
In the present study, Bi2O3 NPs were successfully synthesized through solvothermal method and then conjugated to 5-ALA and FA using (3-aminopropyl)trimethoxysilane (APTMS). Moreover, the prepared NPs were utilized for selective delivery of 5-ALA to the tumor cells. To the best of our knowledge, no study has been conducted to investigate the potential of bismuth oxide NPs for acting as drug delivery agents in PDT. This study is designed to investigate whether or not folate and 5-ALA-conjugated Bi2O3 NPs can improve therapeutic efficiency of PDT on KB cancerous cells.
Experimental
Chemicals and cell line
The main chemical materials used for the synthesis of the NPs were bismuth (III) nitrate pentahydrate (≥98%), di-tert-butyl dicarbonate (BOC; 99%), 5-aminolevinic acid hydrochloride ≥98% (5-ALA; molecular weight of 167.59 gr/mol), propylene glycol, ethanol, toluene (anhydrous, 99.8%), ethyl-3-(3-dimethylaminopropyl) carbodiimide (EDC, ≥97%), N-hydroxysuccinimide (NHS, ≥98%), folic acid (FA; C19H19N7O6), (3-aminopropyl) trimethoxysilane (APTMS, 97%), tetrahydrofuran (THF, ≥99.9%) and calcium chloride (CaCl2, ≥99.9%), all purchased from Sigma-Aldrich (Missouri; USA). Sodium bicarbonate (NaHCO3), citric acid (≥99.5%), dichloromethane (CH2Cl2, ≥99.9%) and diethylether (Et2O), all purchased from Merck (Germany). The materials used for the cell culture and MTT assay included Dulbecco’s modified Eagle’s medium (DMEM-F12), phosphate buffered solution (PBS), fetal bovine serum (FBS), trypan blue, trypsin–EDTA 0.25% all purchased from Bioidea company (Tehran, Iran). MTT powder (3-[4,5-dimethylthiazol-2-yl]-2,5-diphenyltetrazolium bromide) and Caspase-3 assay colorimetric kit were purchased from Sigma-Aldrich (Missouri; USA). The FR-positive human KB cell line (nasopharyngeal epidermal carcinoma) was gifted from Dr. Ali Shakeri-zadeh (Iran University of Medical Sciences, Iran).
Cells illumination device
A light-emitting diode array with power density of 44.8 ± 2.7 mW/cm2 was used as light source in the present study. The LED array was consisted of eight individual lamps dedicated for eight specified wells of a 96-well plate. The output light had peak emission wavelength of 630 nm and a band with the full width at half maximum (FWHW) of 20 nm. Illuminations were performed for 10 min to deliver the light dose of 26 J/cm2.
Synthesis and modification of Bi2O3 NPs
The Bi2O3 NPs were synthesized from bismuth nitrate using the methodology outlined in the article published by Luan et al. [Citation23]. First, bismuth (III) nitrate (1.94 g) was dissolved in propylene glycol (20 mL) under vigorous stirring overnight. The mixture was then placed in an oven at 150 °C for 3 h. The dried powder was dissolved in ethanol and then centrifuged at 5,000 rpm for 5 min. The supernatant was removed and the sediment was again washed with ethanol; then centrifuged. This process repeated three times. The resultant product was dried at 80 °C.
For functionalizing the synthesized NPs as described by Bogusz et al. [Citation24], 75 mg of the synthesized Bi2O3 NPs from the previous step were suspended in dry toluene (50 mL) and sonicated at 50 °C for 30 min; the mixture was purged with N2 gas to remove the dissolved oxygen, simultaneously the APTMS was added drop-wise (66/min). Thereafter, it was again sonicated at the same temperature for another half an hour and finally the mixture was stirred overnight. Afterwards, the mixture was centrifuged at 5,000 rpm for 5 min. The product obtained from centrifugation was washed with ethanol and water solution and dried at 60 °C for 5 h.
5 -ALA conjugation
According to the data reported by Shendage et al. [Citation25]; first, 50 mg 5-ALA, 109.5 mg BOC, 106 mg NaHCO3, 12.5 mL THF and 12.5 mL H2O were mixed together by stirring in the refrigerator for 17 h. The resulting product was extracted twice with 25 mL of Et2O. The aqueous solution was acidified to the pH below 5 (pH ) using citric acid. The product was then extracted twice and each time with 30 mL of dichloromethane and the aqueous phase was discarded. A few pieces of CaCl2 were added to the product to remove water in the final organic phase.
The protected 5-ALA (1 mg) was mixed with 5 mL of PBS with pH7.4. Then, EDC (0.88 mg) was dissolved in PBS (1 mL) and added to the previous solution and stirred for 20 min. In addition, NHS (0.53 mg) was dissolved in PBS (1 mL) and added to the previous solution and stirred for another 20 min. Thereafter, 10 mg of modified NPs were dispersed in 10 mL PBS and added to the previous solution, followed by 30 min stirring.
FA conjugation
The carboxyl group activation of the FA was necessary for conjugating FA to modified NPs. For this purpose, FA (1 mg) was dissolved in PBS (1 mL). Then, EDC (0.5 mg) was dissolved in 1 mL PBS and added to the above solution, then stirred at room temperature for 20 min. Afterwards, NHS (0.5 mg) was dissolved in 1 mL PBS and then added to the above solution followed by another 20 min stirring. The activated FA solution was then added to the 5ALA-conjugated NPs solution from previous step followed by 30 min stirring. The process of preparing FA-5ALA-conjugated Bi2O3 NPs is demonstrated in .
Figure 1. Schematic of nano-carrier preparation stages; fabrication of functionalized NPs (step 1), blocking the NH2 group of 5-ALA using BOC (step 2), 5-ALA@BOC activation using carbodiimides and conjugation of the resultant compound to functionalized NPs (step 3), FA activation and its conjugation to the product of step 3 (step 4).
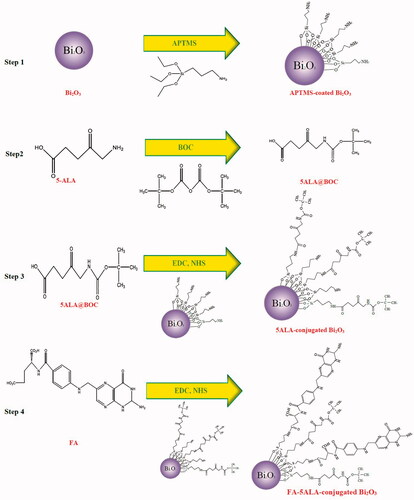
Cell culture
KB cells were grown monolayer using DMEM-F12 supplemented with 10% FBS in 25 cm2 flasks. The cell growth was carried out in a humidified atmosphere containing 5% CO2 at 37 °C. The culture medium was changed regularly to obtain a sufficient number of cells. When the cells reached to the confluency of more than 70%, they were washed with PBS and incubated with the trypsin–EDTA solution for 3 min at 37 °C to detach them from the dish. The cells were then re-suspended in the culture medium for seeding. Trypan blue staining was performed to determine the cell density before seeding and for doubling time measurement, at time points of 0, 24, 48 and 72 h after plating.
Incubation time measurement
KB cells were seeded in 12-well plates at the density of 5 × 104 cells/well. After 24 h, the medium was removed and replaced with serum-free DMEM-F12 containing 20 μ mL/ mL FA-5ALA-conjugated NPs, 5ALA-conjugated NPs and free 5-ALA, then incubated for 1, 2, 3, 4 and 6 h to determine the optimum incubation period before PDT execution. After twice washing un-internalized materials with PBS, the cells were lysed by triton X-100 (1%) solution. Then, they centrifuged at 12,000 rpm for 3 min and thereupon, the supernatant were collected gently. Thereafter, Pp-IX fluorescence intensity of the compounds were measured using a Synergy H1 Microplate Reader (BioTek) with excitation and emission wavelengths of 407 and 602 nm, respectively. Protein concentrations were also equalized for each time point.
Cytotoxicity of the conjugated NPs and free 5-ALA
For determining the darkness toxicity of each agent and finding out a non-toxic concentration of the compounds for KB cells, 5 × 104 cells/well were seeded in 96-well plates with medium supplemented with 10% FBS and incubated overnight. After 24 h, the cells were incubated for 2 h with 1, 10, 20, 50, 100 and 200 μg/ mL concentration of the FA-5ALA-conjugated NPs, 5ALA-conjugated NPs and free 5-ALA. It is important to mention that the concentration of the conjugated NPs was determined based on the existing 5-ALA concentration in the suspension of the compound. After 24 h, MTT assay was carried out using an ELISA ELx800 Microplate Reader (BioTek) at 490 nm. The cells were not illuminated during this study.
Examining the effects of PDT
Fresh KB cells were seeded in 96-well plates at the density of 5 × 104 cells/well. After 24 h, the medium was replaced with serum-free DMEM-F12 containing 20 μg/ mL FA-5ALA-conjugated NPs, 5ALA-conjugated NPs and free 5-ALA, then incubated for 2 h. Afterwards, un-internalized materials were removed by washing cells twice with PBS. Cells were then illuminated under the custom-made LED lamp (630 nm) with the light dose of 26 J/cm2. Irradiated cells were grown in 5% FBS-containing DMEM-F12 for 24 h, and then subjected to MTT and caspase-3 activity assays. Experimental groups are shown in .
Table 1. Photodynamic treatment conditions of the different groups.
MTT assay
Cell viability was evaluated by the MTT assay. After unloading the wells from cell-culture medium, DMEM-F12 supplemented with 10% FBS containing MTT solution (0.5 mg/ mL) were added to each well. The plates were kept in an incubator for 2 h. Then, the medium was replaced by 100dimethyl sulphoxide (DMSO). Optical absorption of each well was measured using an ELISA Reader. The cell survival percentage was measured in comparison to the control group.
Caspase-3 activity assay
Caspase-3 activity assay was performed using the colorimetric caspase kit. For this purpose, the cells were treated based on the groups mentioned in . After 24 h, they were washed with PBS, trypsinized and centrifuged at 1400 rpm for 7 min. Then, 30 μL of the cell lysis buffer was added to each group. Each group was placed in ice for 30 min and subsequently centrifuged at 14,000 rpm for 15 min. Finally, 10 μL of supernatant was combined with caspase-3 colorimetric substrate and 75 μL of buffer assay solution (1%). This mixture was incubated for 1, 2 and 24 h at 37 °C, the absorbance was measured at 405 nm using a microplate reader. Protein concentrations were also equalized for each condition.
Measuring mitochondrial membrane potential (MMP)
Mitochondria play an important role in the cells' apoptotic pathway [Citation26]. In the current study, rhodamine-123 (Rh123), as a fluorescent dye, was used for determining MMP. During cell apoptosis, the MMP is depolarized and causes the loss of Rh123 from the mitochondria followed by intracellular fluorescence intensity reduction. KB cells were plated (5 × 104 cells/well), incubated overnight, and then incubated with 20 μg/ mL concentration of the selected materials for 2 h. The medium was then replaced with DMEM-F12 containing 5% FBS and cells were immediately illuminated at 26 J/cm2 based on the groups mentioned in . After 24 h, cells were incubated with 2 μL Rh123 for 45 min, then washed with PBS and 75 μL triton X-100 (1%) was added to each well. The plates were then placed in refrigerator for 30 min in order to cells to be lysed. The fluorescence intensity was measured (excitation, emission wavelength of 488 and 520 nm, respectively) using a fluorescence microplate-reader. The fluorescence intensity corresponds to the value of MMP [Citation27].
Intracellular ROS detection
Intracellular ROS levels were determined using DCFH-DA which is a non-fluorescent lipophilic ester and easily passes through the cell membrane. When this molecule is oxidized to fluorochrome DCF, green fluorescence is emitted. The cellular fluorescence intensity reflects the ROS level [Citation28]. After seeding for 24 h, KB cells were washed with PBS, pretreated with selective compounds for 2 h and immediately illuminated at the light dose of 26 J/cm2. After 24 h, the cells were incubated with 2 μL DCFH-DA for 30 min, then lysed with triton X-100 (1%). The fluorescence was measured (excitation, emission wavelength of 488 and 528 nm, respectively) using a fluorescence microplate-reader and fluorescent microscopic images were taken by Cytation 5 cell imaging reader (BioTek).
Statistical analysis
All the data was analyzed using one-way ANOVA followed by Tukey’s test as the post hoc analysis using Graph Pad Prism software (San Diego, CA, USA). The data are represented as mean ± standard deviation (SD) of the three independent experiments and compared with control group. Differences between the mean ± SD of samples were considered significant at *p<.05, **p<.01 and ***p<.001.
Results
NPs' characterization
Morphology and size distribution of the NPs using TEM
Transmission electron microscopy (TEM) was used for the study of morphology and size distribution of the synthesized NPs (Philips model CM30; operated at 150 kV). shows the TEM image of the Bi2O3 NPs synthesized at the solvothermal temperature of 150 °C. The TEM micrographs shows an hexagonal or nearly hexagonal morphology for these particles with a little agglomeration, and the particle sizes are in the range of 5−50 nm. The analysis of the TEM images carried out on 200 NPs indicated an average diameter of 19.2 ± 6.5 nm for NPs ().
FT-IR spectroscopic characterization of synthesized NPs
shows the Fourier Transform Infrared spectrum (FT-IR) of different synthesis stages of nanocarrier recorded by IR Prestige-21 spectrometer (Shimadzu, Japan). As demonstrated in , the Bi2O3, APTMS-coated Bi2O3, 5ALA-conjugated Bi2O3 and FA-5ALA-conjugated Bi2O3 NPs were investigated. Regarding the FTIR of Bi2O3 NPs, the peak at 1381.03 cm−1 is the characteristic one of NO3– group and the broad band at about 516 cm−1 is attributed to the metal oxygen vibration (Bi[sbond]O) that represents the successful synthesis of Bi2O3 NPs [Citation29]. The aminated Bi2O3 NPs with APTMS showed the broad peak at 1597.06 and 3379.29 cm−1 which are attributed to the vibration of amine groups on the surface of modified NPs. The spectrum of 5ALA-conjugated Bi2O3 showed the decrease in the intensity of the –NH2 vibration which proved the conjugation of some of the amine groups with BOC-decorated 5-ALA. In addition, the vibration bands at 2858.51 and 2924.09 cm−1 belong to the CH2 and [sbond]CH3 groups of BOC and 5-ALA. The shoulder around 1700 cm−1 belongs to the C[dbond]O group of folate in FA-5ALA-conjugated Bi2O3 NPs [Citation30]. Moreover, the band at 1400–1600 cm−1 and peak at 1083.99 cm−1 is apportioned to bending vibration mode of aromatic C=C of FA in FA-5ALA-conjugated Bi2O3 NPs [Citation31]. , shows the comparison of FA-5ALA-conjugated Bi2O3 NPs with free 5-ALA and folate. As can be seen, the main peak of 5-ALA (the peak at 2854.65–2924.09 cm−1) and folate (the peak at 1693.50 cm−1) remains almost constant.
Surface charge
Zeta potential indicates the surface charge, which determines particle stability in dispersion. The zeta potential of conjugated-NPs were measured using Zetasizer NANO ZS (Malvern). The results are shown in . The zeta potential of the synthesized 5ALA-conjugated NPs and FA-5ALA-conjugated NPs determined to be 11.4 and –5.53 mV, respectively.
Cell growth rates
Morphology of the KB cells is shown in . Cellular density was determined by trypan blue staining at time points of 0, 24, 48 and 72 h after plating. The growth curve of the cells was obtained in semi-logarithmic scale () by counting the number of living cells in each time. Doubling time of the cells was calculated using the online software of calculating the doubling time at www.doubling-time.com. According to the calculations, the doubling time of the KB cell was about 16.37 h which is in agreement with data presented by Nassiri et al. [Citation32].
Pp-IX formation
The cellular uptake of FA-5ALA-conjugated NPs, 5ALA-conjugated NPs and free 5-ALA at pre-determined time points (1, 2, 3, 4 and 6 h incubation) was assessed in KB cells. Cellular uptake was evaluated utilizing the inherent fluorescence of Pp-IX (excitation 407 nm; emission 602 nm). As shown in , strong fluorescence was observed with FA-5ALA-conjugated NPs after 2 h of incubation, which was stronger than that of the free 5-ALA and 5ALA-conjugated NPs (p<.001).
Figure 6. (a) Recorded Pp-IX fluorescence signal after various incubation periods of cells with 20 concentration of free 5-ALA, 5ALA-conjugated and FA-5ALA-conjugated NPs. The signal of control group was subtracted from other groups. (b) Cell survival percentage after 2 h incubation with different concentrations of the mentioned materials.
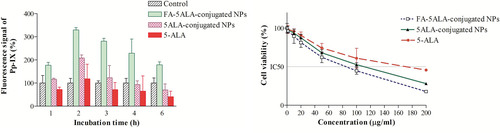
Cytotoxicity of the conjugated NPs and free 5-ALA
In order to find a nontoxic concentration of the materials, cells were seeded in 96-well plates. After 24 h, they were incubated with 1, 10, 20, 50, 100 and 200 μg/ mL concentration of each substance in serum-free medium for 2 h. Thereafter, cells were washed twice with PBS, then incubated with medium containing 5% FBS for 24 h. MTT assays were performed to measure the cells' viabilities. The result is shown in . Due to non-toxic effect of compounds at lower concentrations, 20 μg/ mL concentration was chosen for the rest of the study.
Material-based treatment evaluation using MTT assay
KB cells were incubated with materials as described in for 2 h. Immediately after that, PDT was executed in optical dose of 26 J/cm2. As shown in , the efficacy of PDT on the cells increased in the presence of free 5-ALA, 5ALA-conjugated NPs and FA-5ALA-conjugated NPs. The significant difference was observed between the control group and the groups receiving 5ALA-conjugated NPs (group 4) and FA-5ALA-conjugated NPs (group 5) (p<.001). However, no significant difference was noted in cell viability between illumination alone and control groups (p>.05).
Figure 7. (a) Cell survival percentage after PDT in the absence of PS and in the presence of free 5-ALA, 5ALA-conjugated and FA-5ALA-conjugated NPs. (b) Effects of the material-based treatment on caspase-3 activity. (c) Effects of selected material and subsequent PDT on MMP in KB cells (*p<.05, **p<.01, ***p<.001).
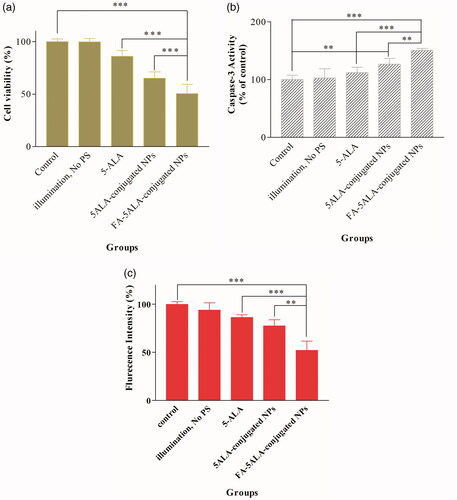
Effect s of the material-based treatment on caspase-3 activity
The activity of caspase-3 enzyme was evaluated to verify effects of the material-based treatment on cellular death in our experiments. Results showed that treatment with 20 μg/ mL concentration of selected materials increased caspase-3 activity in KB cell line (). Both 5ALA-conjugated and FA-5ALA-conjugated NPs were obviously able to increase caspase-3 activity to ∼126% and ∼151%, respectively. There is no significant difference between the group receiving illumination without PS and the control group (p>.05).
MMP analysis
The ability of free 5-ALA, 5ALA-conjugated NPs and FA-5ALA-conjugated NPs to change the MMP and induction of apoptosis was determined using Rh123. Treatment approach was performed as described in and 24 h after that, results showed the significantly decreased fluorescence signal (p<.001) when KB cells were exposed to 20 μg/ mL concentration of FA-5ALA-conjugated NPs and subsequent PDT ().
Effects of the material-based treatment on ROS generation
In order to determine oxidative stress induced by PDT, DCFH-DA assay was performed. Images which were taken using Cytation 5 Cell Imaging Reader showed a noticeable ROS generation in cells treated with 5ALA-conjugated and FA-5ALA-conjugated NPs after 2 h of incubation and subsequent PDT (). It must be noted that illumination without PS had no effect on intracellular ROS levels in KB cell line (). As shown in , the significant differences (p<.001) in level of ROS generation were obtained among the groups using fluorescence microplate-reader which were in accordance with the images. ROS levels in cells exposed to 5ALA-conjugated and FA-5ALA-conjugated NPs had increased up to 232% and 405.3%, respectively.
Figure 8. Formation of ROS after different treatment conditions of KB cells. (a) Control; no PS, no PDT. (b) No PS, just PDT. (c) 5-ALA and subsequent PDT. (d) 5ALA-conjugated NPs and subsequent PDT. (e) FA-5ALA-conjugated NPs and subsequent PDT. (f) DCF fluorescence detected by Synergy H1 Microplate Reader.
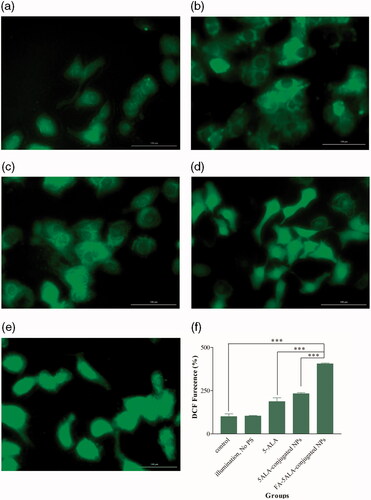
Discussion
In order to overcome the main limitations of the PDT including low penetration of light into tissues, poor accumulation of the PSs into tumor [Citation33] and improving the efficacy of the photodynamic treatment for cancer management, targeted delivery of PSs to tumor cells is considered as a developing approach in precision medicine [Citation7]. Recent studies have focused on the receptors which are overexpressed on the surface of cancerous cells through which targeted delivery of the drugs to the tumor would be expected [Citation21]. FA receptors are overexpressed on the surface of different cancerous cells including nasopharyngeal carcinoma cells (KB cell line) [Citation34]. Therefore, utilizing folate as a targeting ligand became a promising strategy since it is non-immunogenic, specific for many types of cancer cells, and could possibly conjugate with a number of nanotechnology platforms [Citation35]. In the present study, FA-conjugated Bi2O3 NPs have been utilized for the selective and effective delivery of 5-ALA to the tumor cells. This might facilitate the penetration of 5-ALA directly into the cells where the Pp-IX is generated and can act as a strong PS for PDT purposes [Citation36]. Bi2O3 NPs were synthesized with the average diameter of 19.2 ± 6.5 nm, then covered with APTMS in form of a shell, to which 5-ALA was covalently bound via amidation. After that the compound was conjugated with FA. The conjugated NPs easily internalized by the cells due to their small sizes. Coating with APTMS, also facilitated their dispersibility and stability [Citation24]. Eventually, the cell toxicity of the free 5-ALA, 5-ALA-conjugated and FA-5ALA-conjugated NPs was evaluated alone or in combination with PDT execution.
The FA-5ALA-conjugated-Bi2O3 NPs were found to be toxic for KB cells in the applied concentrations, however, at the concentration of 20 μg/mL, which was applied in our experiments, showed 20% toxicity (). Our results also demonstrated that the uptake of FA-5ALA-conjugated NPs by the cells was much higher than that of other groups and caused more toxicity than others. Both the MTT and caspase-3 activity studies confirmed that the FA-5ALA-conjugated NPs has high level of cytotoxicity when the NP concentration is 20 μg/ mL and PDT is executed at the light dose of 26 J/cm2. As shown in , maximum cellular death (∼50%) was obtained in the presence of FA-5ALA-conjugated NPs and subsequent PDT while the viability of KB cells using 5ALA-conjugated NPs and free 5-ALA followed by PDT is ∼65% and 85%, respectively. The reason behind this may be because of two claims; one of them could be the fact that the Bi2O3 NPs can increase the cellular death by disrupting on mitochondrial and lysosomal functions and induce apoptosis [Citation37]. Together with PDT, the Bi2O3 NPs could decrease the cells’ viability in KB cell line. Another reason could be active targeting effect of FA that is based on the specific identification of folate receptors on the surface of KB cells [Citation38].
Since the cytotoxic effects of ALA-PDT depend on targeting of mitochondria [Citation39], in this study we aimed to specifically evaluate mitochondrion-affecting parameters including MMP and ROS generation [Citation40]. A change in trans-membrane potential of the mitochondrion is one of the earliest changes occurs during apoptosis. Rh123 is a cationic dye which accumulates in mitochondrial membrane. Loss of MMP leads to loss of Rh123 fluorescence. Therefore, Rh123 can be used to detect MMP dissipation and thus, it has wide scope in research on apoptosis. In the present study, the ability of free 5-ALA, 5ALA-conjugated and FA-5ALA-conjugated NPs for apoptosis induction was compared with the control group using Rh123. Based on our findings, FA-5ALA-conjugated NPs can effectively induce apoptotic cell death compared to free 5-ALA and 5ALA-conjugated NPs.
Another important factor affecting the apoptotic process is the generation of ROS, so that the excess of ROS generation enhances the permeability of mitochondrial membrane, thus disturbs the respiratory chain to trigger the apoptotic pathway [Citation41]. In this study, the treatment with 20 μg/ mL of FA-5ALA-conjugated NPs and subsequent PDT showed a greater potential to induce a strong green fluorescence and considerable increase in the level of ROS generation, indicating that with the mediation of NPs, a large amount of ROSs was produced.
Conclusions
Aiming at the selective intracellular release of 5-ALA in the PDT of cancer, Bi2O3 NPs have been successfully fabricated by a simple and cost-effective hydrolysis solvothermal method and then conjugated to 5-ALA and FA. According to the cytotoxicity results on KB cell line with and without PDT, the efficacy of FA-5ALA-conjugated NPs for the intracellular uptake of 5-ALA was proved as a result of the FA-receptor mediated endocytosis.
Acknowledgements
This work was performed in fulfilment of the requirements for the Master of Science degree of Fatemeh Akbarzadeh, in the School of Medicine, Kermanshah University of Medical Sciences, Kermanshah, Iran. We also wish to thank Dr. Sahar Jamshidi-Navid for her collaboration in the work and her valuable helps.
Disclosure statement
No potential conflict of interest was reported by the authors.
Additional information
Funding
References
- Xu Y, Shi Z, Zhang L, et al. Layered bismuth oxyhalide nanomaterials for highly efficient tumor photodynamic therapy. Nanoscale. 2016;8(25):12715–12722.
- Wen X, Li Y, Hamblin MR. Photodynamic therapy in dermatology beyond non-melanoma cancer: an update. Photodiagn Photodyn Ther. 2017;19:140–152.
- Mohammadi Z, Sazgarnia A, Rajabi O, et al An in vitro study on the photosensitivity of 5-aminolevulinic acid conjugated gold nanoparticles. Photodiagn Photodyn Ther. 2013;10(4):382–388.
- Makhadmeh GN, Abdul Aziz A, Abdul Razak K. The efficacy of methylene blue encapsulated in silica nanoparticles compared to naked methylene blue for photodynamic applications. Artif Cells Nanomed Biotechnol. 2016;44(3):1018–1022.
- Tahmasebi H, Khoshgard K, Sazgarnia A, et al Enhancing the efficiency of 5-aminolevulinic acid-mediated photodynamic therapy using 5-fluorouracil on human melanoma cells. Photodiagn Photodyn Ther. 2016;13:297–302.
- Xu J, Gao J, Wei Q. Combination of photodynamic therapy with radiotherapy for cancer treatment. J Nanomater. 2016;2016(5):1–7.
- Wei Y, Wei Z, Luo P, et al pH-sensitive metal–phenolic network capsules for targeted photodynamic therapy against cancer cells. Artif Cells Nanomed Biotechnol. 2017:1–10. doi:10.1080/21691401.2017.1377724
- Ghoodarzi R, Changizi V, Montazerabadi A, et al. Assessing of integration of ionizing radiation with Radachlorin-PDT on MCF-7 breast cancer cell treatment. Lasers Med Sci. 2016;31(2):213–219.
- Ma M, Huang Y, Chen H, et al. Bi2S3-embedded mesoporous silica nanoparticles for efficient drug delivery and interstitial radiotherapy sensitization. Biomaterials. 2015;37:447–455.
- Koo E, Austin E, Mamalis A, et al Efficacy of ultra short sub‐30 minute incubation of 5‐aminolevulinic acid photodynamic therapy in vitro. Lasers Surg Med. 2017;49(6):592–598.
- Xu H, Liu C, Mei J, et al. Effects of light irradiation upon photodynamic therapy based on 5-aminolevulinic acid–gold nanoparticle conjugates in K562 cells via singlet oxygen generation. Int J Nanomed. 2012;7:5029.
- Zhang Z, Wang S, Xu H, et al Role of 5-aminolevulinic acid-conjugated gold nanoparticles for photodynamic therapy of cancer. J Biomed Opt. 2015;20(5):051043.
- Chatterjee DK, Fong LS, Zhang Y. Nanoparticles in photodynamic therapy: an emerging paradigm. Adv Drug Deliv Rev. 2008;60(15):1627–1637.
- Norouzi H, Khoshgard K, Akbarzadeh F. In vitro outlook of gold nanoparticles in photo-thermal therapy: a literature review. Lasers med sci. 2018:1–10.
- Hossain M, Su M. Nanoparticle Location and material-dependent dose enhancement in X-ray radiation therapy. J Phys Chem C. 2012;116(43):23047–23052.
- Alqathami M, Blencowe A, Geso M, et al Quantitative 3D determination of radiosensitization by bismuth-based nanoparticles. J Biomed Nanotechnol. 2016;12(3):464–471.
- Su X-Y, Liu P-D, Wu H, Gu N. Enhancement of radiosensitization by metal-based nanoparticles in cancer radiation therapy. Cancer Biol Med. 2014;11(2):86–91.
- Stewart C, Konstantinov K, McKinnon S, et al. First proof of bismuth oxide nanoparticles as efficient radiosensitisers on highly radioresistant cancer cells. Phys Med. 2016;32(11):1444–1452.
- Khoshgard K, Hashemi B, Arbabi A, et al. Radiosensitization effect of folate-conjugated gold nanoparticles on HeLa cancer cells under orthovoltage superficial radiotherapy techniques. Phys Med Biol. 2014;59(9):2249.
- Butzbach K, Rasse‐Suriani FA, Gonzalez MM, et al. Albumin–folate conjugates for drug‐targeting in photodynamic therapy. Photochem Photobiol. 2016;92(4):611–619.
- Zhang H, Li J, Hu Y, et al. Folic acid-targeted iron oxide nanoparticles as contrast agents for magnetic resonance imaging of human ovarian cancer. J Ovarian Res. 2016;9(1):19.
- Ghaznavi H, Hosseini-Nami S, Kamrava SK, et al. Folic acid conjugated PEG coated gold–iron oxide core–shell nanocomplex as a potential agent for targeted photothermal therapy of cancer. Artif Cells Nanomed Biotechnol. 2017:1–11. Available from: 10.1080/21691401.2017.1384384
- Luan X, Jiang J, Yang Q, et al Facile synthesis of bismuth oxide nanoparticles by a hydrolysis solvothermal route and their visible light photocatalytic activity. Environ Eng Manage. 2015;14(3):703–707.
- Bogusz K, Tehei M, Stewart C, et al. Synthesis of potential theranostic system consisting of methotrexate-immobilized (3-aminopropyl) trimethoxysilane coated α-Bi2O3 nanoparticles for cancer treatment. RSC Adv. 2014;4(46):24412–24419.
- Shendage DM, Fröhlich R, Haufe G. Highly efficient stereoconservative amidation and deamidation of α-amino acids. Org Lett. 2004;6(21):3675–3678.
- Shoji KF, Debure L. Fluorometric methods for detection of mitochondrial membrane depolarization induced by CD95 activation. CD95. Berlin: Springer; 2017. p. 49–62.
- Shang H, Han D, Ma M, et al Enhancement of the photokilling effect of TiO2 in photodynamic therapy by conjugating with reduced graphene oxide and its mechanism exploration. J Photochem Photobiol B. 2017;177:112–123.
- Wang H, Yu X, Su C, Shi Y, et al. Chitosan nanoparticles triggered the induction of ROS-mediated cytoprotective autophagy in cancer cells. Artif Cells Nanomed Biotechnol. 2018:1–9. Available from: https://doi.org/10.1080/21691401.2017.1423494
- Mallahi M, Shokuhfar A, Vaezi M, et al. Synthesis and characterization of bismuth oxide nanoparticles via sol–gel method. AJER. 2014;3:162–165.
- Chen J, Peng Q, Jodl H-J. Infrared spectral comparison of 5-aminolevulinic acid and its hexyl ester. Spectrochim Acta A Mol Biomol Spectrosc. 2003;59(11):2571–2576.
- Azandaryani AH, Kashanian S, Derakhshandeh K. Folate Conjugated hybrid nanocarrier for targeted letrozole delivery in breast cancer treatment. Pharm Res. 2017;34(12):2798–2808.
- Nassiri MR, Hanks CT, Cameron MJ, et al Application of flow cytometry to determine the cytotoxicity of urethane dimethacrylate in human cells. J Biomed Mater Res A. 1994;28(2):153–158.
- Mohammadi Z, Sazgarnia A, Rajabi O, et al Comparative study of X-ray treatment and photodynamic therapy by using 5-aminolevulinic acid conjugated gold nanoparticles in a melanoma cell line. Artif Cells Nanomed Biotechnol. 2017;45(3):467–473.
- Beik J, Jafariyan M, Montazerabadi A, et al. The benefits of folic acid-modified gold nanoparticles in CT-based molecular imaging: radiation dose reduction and image contrast enhancement. Artif Cells Nanomed Biotechnol. 2017:1–9. Available from: https://doi.org/10.1080/21691401.2017.1408019
- Zeinizade E, Tabei M, Shakeri-Zadeh A, et al. Selective apoptosis induction in cancer cells using folate-conjugated gold nanoparticles and controlling the laser irradiation conditions. Artif Cells Nanomed Biotechnol. 2018:1–13. Available from: https://doi.org/10.1080/21691401.2018.1443116
- Takahashi J, Misawa M, Iwahashi H. Combined treatment with X-ray irradiation and 5-aminolevulinic acid elicits better transcriptomic response of cell cycle-related factors than X-ray irradiation alone. Int J Radiat Biol. 2016;92(12):774–789.
- Abudayyak M, Öztaş E, Arici M, et al Investigation of the toxicity of bismuth oxide nanoparticles in various cell lines. Chemosphere. 2017;169:117–123.
- Mirrahimi M, Hosseini V, Kamrava SK, et al. Selective heat generation in cancer cells using a combination of 808 nm laser irradiation and the folate-conjugated Fe2O3@ Au nanocomplex. Artif Cells Nanomed Biotechnol. 2017:1–13. Available from: https://doi.org/10.1080/21691401.2017.1420072
- Yanase S, Nomura J, Matsumura Y, et al. Enhancement of the effect of 5-aminolevulinic acid-based photodynamic therapy by simultaneous hyperthermia. Int J Oncol. 2005;27(1):193–201.
- Naserzadeh P, Ansari Esfeh F, Kaviani M, et al. Single-walled carbon nanotube, multi-walled carbon nanotube and Fe2O3 nanoparticles induced mitochondria mediated apoptosis in melanoma cells. Cutan Ocul Toxicol. 2018;37(2):157–166.
- Sriram MI, Kalishwaralal K, Barathmanikanth S, et al. Size-based cytotoxicity of silver nanoparticles in bovine retinal endothelial cells. Nanosci Methods. 2012;1(1):56–77.