Abstract
Nanofibrous scaffolds are considered as a new strategy for Type 1 diabetes mellitus therapy. We used a hybrid of poly-l-lactic acid (PLLA) and polycaprolactone (PCL) as three-dimensional (3D) culture models for differentiation of human induced pluripotent stem cells (hiPSCs) to beta islet-like cluster cell compared with routine culture (2D). Morphological changes of cells were checked by microscope. mRNA endodermal SOX-17 on day 7 and pancreatic gene markers Pdx1, glucagon and Glut2 were evaluated on day 23 by qPCR. As well as, insulin and C-peptide protein expression was evaluated by immunocytochemistry staining. In addition, insulin and C-peptide secretion in various glucose concentrations was evaluated by ELISA. Light and scanning electron microscopy (SEM) microscope showed changes in induced cells. In tandem, these modifications were more evident in 3 D culture. Pdx1, Glucagon and Glut2 markers in PLLA/PCL were significantly higher in 3 D culture. In addition, qualitative immunochemistry showed that insulin and C-peptide were expressed in 2 D and 3 D culture medium. Furthermore, evaluation of insulin and C-peptide clarified that secretion of these proteins in PLLA/PCL scaffold were statistically different in 2 D and 3 D strategies. These findings suggest that functional matured induction cells on PLLA/PCL scaffold can be used for islet beta cell therapy and regenerative medicine.
Introduction
Dysfunction of Beta-cells due to autoimmune reactions has profound devastating metabolic effects which consequently lead to Type I diabetes mellitus (T1DM). In this disease, the destruction of insulin-secreting beta cells occurs in the islets of Langerhans. Unfortunately diabetes is closely related to stroke and cardiovascular diseases [Citation1]. Its prevalence is expanding and is resulting in devastating morbidity and accelerated mortality in the world [Citation2–4]. Over the past decades, golden clinical standard suggested that islet cell transplantation for these patients as long-term treatment is promising in achieving insulin independence [Citation5,Citation6]. However, problems such as donor organ shortage, high risks of surgery and Immunologic transplant rejection have limited the successful development of this treatment. Thus, the novel alternative strategies such as gene transfer [Citation7] and usage of induced pluripotent stem cell, as well as three-dimensional (3D) culture of nanofibrous scaffolds should be developed to overcome these limitations [Citation8]. Nevertheless, the first method is expensive, difficult and time consuming. Therefore to circumvent these problems, many reports proposed that a proper choice for insulin-producing beta cells is identification of induce human iPSCs and nanofibrous scaffolds to produce beta cells. These processes according to cell–cell, cell–matrix and cell–scaffold contacts are important for the regulation of cell growth and differentiation, similar to natural Extra Cellular Matrix [Citation9]. Human iPS cells are specific cell types that are ideal for differentiation and could offer as an unlimited source for insulin producing cells which potentially will enhance the transplantation therapy in Type I diabetes mellitus patients [Citation10]. Furthermore, nanofibrous scaffolds which were used in regenerative medicine, have a topographic structure with wide surface, and has support structures of natural ECM microenvironment [Citation11]. This condition makes them more practical than two-dimensional culture [Citation12]. Recently, a number of studies have used various scaffolds such as PES[7], PLLA/PVA [Citation13] and PCL/PVA[Citation14] to obtain human iPSCs insulin-producing beta cells. However, some of the applied scaffolds such as PES have limitations, like being a non-biodegradable polymer it requested for repairing hard damaged tissues like bone and cartilage [Citation9,Citation15]. Moreover, PES has a low potential to attract cells to penetrate into the nanofibrous structure [Citation7]. Another used scaffold is PCL; this nanofibrous is highly hydrophobic thus resulting in poor cell attachment. Therefore, it needs to be treated with O2 or needs to be blended with other materials. The usage of pure PLLA by the researchers is limited because of its mechanical properties and poor biocompatibility [Citation16,Citation17]. Although polyvinyl alcohol (PVA) is a good candidate scaffold for pancreatic differentiation, its high price makes it difficult to use[Citation18]. Considering this condition, many reports indicated the feasible applications of PLLA/PCL blends [Citation19]. Recently, it has been supposed that nano-scale PLLA/PCL fibers are cost-effective and readily available. These materials are ideal polymers that could provide the most favourably spaced binding sites and geometrical signals, because they are appropriate nano-bio degradable, flexible and biocompatible polymers for soft tissues. As well as, their structure is similar to a natural supramolecular arrangement in the ECM and do not stimulate the immune system [Citation20]. Among different methods to construct nanofibrous, electrospinning has been widely used because of its simplicity, low cost and potential for large scale production of nanofibrous [Citation21,Citation22]. A number of studies have used electrospin nanofibrous as scaffolds for different types of cells like bone, skin, nerve, cartilage, myocardium, tendon and liver [Citation23–25]. Following this pattern, PCL/PLLA nanofibrous was used to investigate its capacity in supporting the successful differentiation of the hiPSCs to Insulin-Producing Islet-Like cells by evaluating expression of biochemical and molecular pancreatic beta-cell markers. The results showed that compared to 2 D culture hybrid 3 D scaffold can provide a good pancreatic differentiation condition for hiPS stem cell therapy and regenerative medicine.
Materials and methods
Scaffold preparation and surface treatment
Hybrid random nanofibrous PLLA/PCL scaffolds were built up via electrospinning in Stem Cell Technology Research Center. To the PCL dissolved in a concentration of 8% (w/w) and PLLA 4% (w/w) in chloroform a second solvent N, N-dimethylformamide (DMF) (8:2, v/v) was added, and the mixture was homogenized. After that, a rotating cylindrical drum was used as collector and was placed at a distance of 15 cm from the needle. A high voltage potential (20 kV) was also applied between the needle and the collector. The disposable syringe containing a polymer solution was forced through the needle and was collected as nanofibrous on the rotating cylinder. Oxygen treatment can increase the surface of hydrophilic polymeric nanofibres. The random nanofibrous membranes were separated from the collector surface and treatment with oxygen was performed using a plasma generator with low frequency, 44 KHz, with a cylindrical quartz reactor (Diener Electronics, Germany). Pure oxygen was introduced into the reaction chamber at 0.4 mbar pressures, and then the glow discharge was performed for 3 min. Plasma-treated sheets were punched with a device of 0.5 cm diameter to make a rim which is 0.5 cm in width to the desired size and was sterilized under ethanol 70% for 30 min, and were left overnight in the F12 medium supplemented with 10% calf serum.
Human induced pluripotent stem cells expansion
Human induced pluripotent stem cells (hiPSCs) [Citation26] were cultured and expanded on inactive mouse embryonic fibroblasts (MEFs) in DMEM F-12 enriched with 10% knockout serum replacement, 100 µM nonessential amino acids, 2 mM l-glutamine, 100 µM 2-mercaptoethanol and 4 ng/ml bFGF, (all from Invitrogen). The cells were incubated under standard conditions at 95% humidity and 5% CO2 at 37 °C.
Embryonic body formation and differentiation into insulin-producing cells
Hipscs colonies for Embryonic Body (EB) formation were detached with collagenase IV (Gibco, 15140–122) every 5–7 days. Aggregated cells were transferred into Petridis nonattachment plates containing iPSC medium without bFGF for EB formation and disposed to differentiation.
For induction, EBs were divided into two groups: One group was plated on PLLA/PCL plasma treated nanofiberous and other group was transferred to gelatin-coated tissue culture plate in four well plates for 3 days in EB medium supplemented with100 ng/ml activin A (Sigma–Aldrich, St. Louis, MO). Then, Activin A was removed and the EBs was incubated for 6 h in EB medium alone. Further treatment with 1 µM Retinoic Acid and FGF (Sigma–Aldrich) was carried out for additional 4 days. After that, for a week, the plates were treated with 20 ng/ml EGF (R&D Systems, Inc., Minneapolis, MN, USA) in DMEM/F12 which was supplemented with N2 and B27. Finally, the premature exocrine-like cells were treated for additional 7 days in 20 ng/ml bFGF, 100 ng/ml glucagon-like peptide-1 (GLP-1, 7–36 amide; Bachem AG, Torrance, CA, USA), 20 ng/ml BMP4 and 10 mM nicotinamide (NA, Sigma–Aldrich) [Citation27,Citation28]. On the following 2 days, 0.5 and 1% insulin-transferrin-selenium (ITS, Gibco, 41400045) was added to this medium, respectively on nanofiber PLLA/PCL and gelatin coated palates. In control group we added hiPSCs media for 23 days.
RNA extraction and real-time PCR of beta cell markers
Total RNA was extracted at days 0, 7 and 23, after starting process from different cells cultured in two and three dimentional culture, as well as control group by using TRIzol Reagent (Gibco, 15596018)? For reverse transcription reaction approximately 2 μg of total RNA was used with the revert aid first strand cDNA synthesis Kit (Fermentas, k1621) and oligo dt primers according to the manufacturer’s instructions. The cDNA was amplified using Takara SYBR Premix ExTaq Master Mix (RR820A). Gene expression levels were quantified by the ABI Light Cycler (ABI step one) and analyzed by rest 2009. Target gene expression values were normalized to the signal obtained for housekeeping gene Beta-Actin mRNA (ActB) and calibrated to the iPSCs. Primers and product lengths are listed in .
Table 1. Primer sequences and conditions used for qPCR.
Immunofluorescence staining
Differentiated sample cells were rinsed twice with PBS and were fixed with 4% paraformaldehyde (Sigma, 30525–89-4) for 20 min at 4 °C and 5 min at room temperature (RT). After washing, the cells were permeabilized with 3% Triton X-100 in PBS. Then were incubated overnight, at 4 °C, with primary-mouse antibodies against human insulin (1:100; ab7760, abcam, Cambridge, MA) and mouse anti-human C-peptide (1:100; ab115199). At the end of the incubation time, the cells were rinsed three times with PBS-Tween 20 (0.1%) and incubated with the FITC labelled goat anti-mouse IgG (1:50; #AF8032, Razi Biotech, Iran) and Texas red-labeled goat anti-mouse IgG (1:100; #ab5884) as appropriate for 45 min at 37 °C in the dark. Subsequently, these subjected cells were washed with PBS-Tween 0.1%, 3 × 5 min. The nuclei were counterstained with 4´,6-diamidino-2-phenylindole [(DAPI) (1 μg/ml)] (Sigma, 28718–90-3). Cell imaging was performed with fluorescence microscope (Nikon; Japan).
Scanning electron microscopy (SEM)
The surface of PLLA/PCL polymer that hiPS Cell-derived Insulin-Producing Islet-Like cells is on it, was washed with PBS and fixed in 2.5% glutaraldehyde for 20 min and was finally dehydrated in a series of graded concentrations of ethanol. Afterward, the cells were dried overnight. Dehydrated constructs was sputter-coated with gold using a sputter coating unit (MED010; Baltec). Top views were imaged at an accelerating voltage of 20 Kv using a Hitachi Model-4500 SEM.
Insulin and C-peptide release analysis
After induction of hiPS, PLLA/PCL and gelatin coated plates along with controls groups were washed three times with PBS and pre-incubated in Krebs–Ringer bicarbonate (KRB) buffer by ELISA (#10–1132-01, #10–1141-01, Mecodia, Uppsala, Sweden) according to the manufacturer’s protocol without the use of glucose at 37 °C for 120 min. After this time, induced cells were incubated for 90 min in KRB buffer and different glucose concentrations (2.5 mM and 27.5 mM) at 37 °C. In order to evaluate the C-peptide content, induced cells were treated with lysis buffer and total protein was extracted, afterward Human C-peptide ELISA Kit ((#10–1132-01, #10–1141-01, Mercodia, Uppsala, Sweden) was used for C-peptide assay. The content of cell total protein) was detected by a BCATM Protein Assay Kit (PIERCE). This assay was done for three times.
Statistical method
T-test was used to compare mRNA expression of OCT-4 and SOX-17 between hiPSCs and endoderm on day 7. Also, T-test was used for analysis of relative gene expression between 2 D and 3 D for pdx1, Glut2 and Glucagon. Moreover, insulin production and c-peptide secretion between 2 D and 3 D in concentrations of 5.5, 15 and 25 was tested by T-test. All studies were performed in triplicate and significance levels was taken as *p < .05 or **p < .01
Results
hiPSCs culture and embryoid body formation
As illustrated in hiPSCs formed flat colonies with sharp edges that exhibited large nuclei and limited cytoplasm (). After passaging and transferring in suspension culture, the hiPSCs clones formed round structures known as embryoid bodies (EBs) ().
PLLA/PCL hybrid scaffold characterization
Randomally, a homogeneous hybrid matrix and uniform PLLA/PCL scaffolds were synthesized by electrospinning and were treated by plasma generator. So they were characterized morphologically by SEM micrographs (). Surface hydrophilicity of nanofibrous was strongly increased after plasma treatment and the cells occupied the pores and were penetrated well onto the surfaces of the hybrid scaffolds.
Morphological changes of hiPCs after seeding on gelatin coated palates (2D) or scaffolds palates (3D)
For beta-like islet inductions, embryoid bodies were seeded in four wells of the tissue culture dishes for over 23 days. As seen in under SEM micrographs, viability, the rate of hiPSCs proliferation and differentiation was recorded during the period of culture. hiPCs on the day 7 after starting the process upon exposure to Act A, RA and FGF began to acquire a round shape and assembled together ( and ). At the second week, the assembled cells became aggregates and new islet-like clusters began to form ( and ). Finally at the end of the differentiation process, the matured cells formed larger masses and bunch formed in both groups that were cultured in PLLA/PCL nanofaiber scaffolds and differentiated in two dimensional culture ( and ).
Pancreatic gene expression
Definitive endoderm markers sox-17 and pluripotent transcription factor Oct-4 were identified by quantitative real-time PCR in the starting day and first week of islet-like cells differentiation. We found that mRNA expression of Oct-4 was significantly (p < .05) down-regulated in endodermal cells. Furthermore, endoderm marker sox-17 had a significantly higher expression on day 7 on PLLA/PCL scaffolds in comparison to the starting day (p < .01). No significant difference was observed in control groups. These results demonstrated that derived hiPSCs could be efficiently differentiated into endoderm in vitro (). As well as, our results determined that pancreatic specific gene markers pdx1, endocrine hormone gene pattern of glucagon and pancreatic polypeptide Glut 2 expressed at 23 days after induction. A significant up regulation in the expression of pancreatic mRNA has been seen in mature islet like cells that differentiated in PLLA/PCL in comparision to other group which differentiated in gelatin palate (p < .01). (). Overall, these results indicated that synthetic PLLA/PCL scaffolds can enhance and improve differentiation of hiPSCs to beta-like cells (These detailed results are shown in supplementary material).
Immunofluorescence
Immunostaining analysis was conducted to test if insulin and C-peptide that are endocrine markers were secreted on the 23th day of differentiation. As confirmed in , in both groups of 2 D and 3 D, protein marker insulin and C-peptide began to appear and positive for insulin and C-peptide secretion in all the induced cells. Briefly, we don’t see any pancreatic secretion marker in the control group (data not shown).
Figure 5. Immunocytochemistry staining showed (A) insulin and C-peptide expression in end stage derived pancreatic cells on hybrid scaffold. (B) Insulin and C-peptide protein, the main markers of beta- like pancreatic cells in matured cells in 2D group. Nuclei were stained with DAPI. Data are presented as mean ± SEM. (× 100 magnifications).
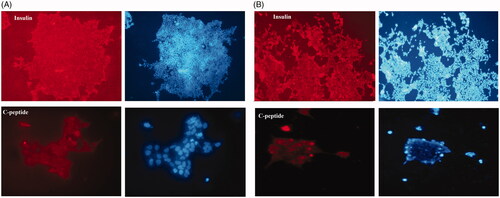
Glucose-induced insulin and C-peptide secretion quantity
We found that insulin secretion increased in the high glucose medium of 15 and 25 mM compared to the low glucose concentration (5.5 mM) and higher level in islet-like cells that differentiated in 3 D culture (p < .01) (). Furthermore, C-peptide release by the differentiated insulin-producing cells was increased by high glucose treatment (p < .01) in a manner comparable to low glucose (p > .05) (). Thus, these results showed that maturated cells in 3 D group could produce significant amount of insulin and C-peptide when compared to the gelatinated group. Details of results are shown in the Supplementary material.
Figure 6. Insulin and C-peptide production in response to glucose in end stage of differentiation. (A) Insulin production and (B) C-peptide release pattern in the studied cultures and control group in response to different concentrations of glucose (C) Insulin normalized with total cellular protein D) C-peptide normalized with total cellular protein. *Significance was defined as p < .05 and values were presented as means ± SD.
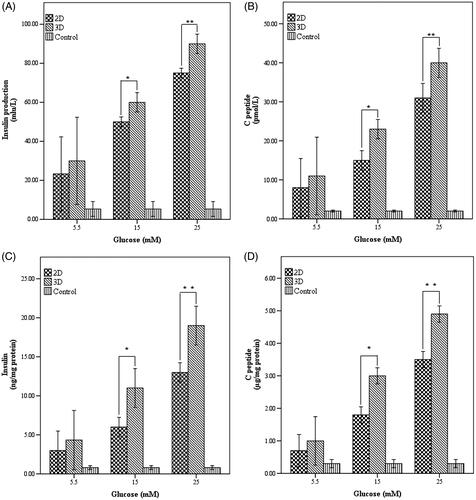
Discussion
The connection between cells and their extracellular microenvironment matrix is vital for cell-cell interaction, proliferation and differentiation. Nanofibrous scaffolds are being used increasingly in tissue engineering and have built broad three dimensional structures that mimics the structures of the natural microenvironment’s extra cellular matrix (ECM) [Citation29,Citation30]. Aloysius and Nair, 2014 reported that using 3 D culture for various cell types’ differentiation is much better than two-dimensional (2D) culture for the cells differentiation [Citation31]. Up to recently, Enderami, Abazari and Nassiri Mansour reported that beta-like cells can be differentiated from hiPSCs on PLLA/PVA, PCL/PVA and PES scaffolds. But our result showed that pancreatic markers are markedly expressed well in PLLA/PCL hybrid scaffold than they reported. Poly (ε-caprolactone) (PCL) is a bioresorbable, biocompatible and inexpensive nanofiber, having native tissue like properties that are generally used for tissue regeneration, as a reported, because of low bioactivity, use of pure PCL in tissue engineering is limited [Citation7,Citation15,Citation16] . Zhang et al., reported that in order to enhance adhesion, proliferation and encourage of cell to growth into the PCL scaffolds, this nanofibres need effective hybridization with other bioactive molecules compared to single PCL [Citation32]. PLLA, the l-form of poly-lactic acid (PLLA), is FDA approved polyester that has been studied extensively as a biodegradable material and has long term stability [Citation17,Citation29]. More importantly, l-lactic acid is the final product of PLLA scaffolds degradation which is harmless for the body [Citation33]. Moreover the fibrous porous structure produced by blending is one of the several efforts used to enhance Polymer hydrophilicity [Citation34] that introduced them as a good candidate for tissue engineering applications, stem cell proliferation and differentiation. Moreover, hybrid scaffold is energy-cost and labor saving without having the disadvantages of single polymer [Citation35] Many studies show that embryonic cells and hiPSCs can differentiate into beta like-cells by cytokine growth factors, microRNA and on gelatin-coated plates or nanofibrous scaffolds, but so far, sufficient studies on differentiation of hiPSCs to pancreatic islet cells on the surface of nano-scaffold hybrids have not been performed. Therefore, in this work hiPSCs were used as sources of stem cells to pancreatic differentiation on these hybrids. In our study we: (1) made polymers that support long-term attachment, proliferation and differentiation of hiPSCs (2) successfully generated the insulin-producing cells from hiPSCs on hybrid scaffold. Similar to Enderami et al [Citation13] our result showed that hiPSCs can tightly stick to scaffolds and penetrate to this hybrid. Also higher expression of pluripotent stem cell marker OCT-4 confirmed the intact characterization of these cells on hybrid scaffold in compared to gelatin coated cells was shown. We examined the ability of hiPSCs to differentiate into endoderm lineages. Similar to our previous reports [Citation26,Citation36] as a showed in , endodermic marker sox 17 was successfully expressed on day 7, also this marker was increased in scaffold hybrid accompanied to Abazari research. In days 7, 14 and 23 after this process, we evaluated the potential of hiPSCs to generate morphologically pancreatic islet-like structures by light and SEM microscope. To agree with Abazari et al. [Citation14] literatures, as illustrated in and in both differentiated groups the cells proceeded from endoderm to final stage, gradually changed to assemble and granulate the shape. Furthermore, in final stage, our research team tested the expression of gene markers Pdx1, Glut-2 and Glucagon hormone. Our findings parallel to Lahmy et al. [Citation37] revealed mRNA of pancreatic factors made a strong change in differentiated cells and can induced its differentiation to a pancreatic lineage. As well as in line with enderami and mansuor in 3 D culture, these markers were significantly more expressed than cells which were induced in two dimentional. Similar to our finding, Mahmoodinia et al. [Citation38] performed differentiation of human iPSCs on IPCs on hybrid nanofibrous scaffold and they showed successful expression of protein marker C-peptide in IPS Cell-derived Insulin-Producing Islet-Like cells. Additionally, similar to Lahmy, in this work we demonstrated that endocrine marker of insulin was secreted from induced cells. Briefly, our findings were similar to Enderami [Citation13], who showed that insulin secretion and C-peptide releasing in 3 D medium is higher than 2 D culture in 25 Mm glucose. As well as, our result is opposite to that of Enderami and Abazari (authors) who revealed that pancreatic marker Glut-2 has higher level in 3 D compared to 2 D it can be due to significant expression of Glut-2 gene between the two groups. With regards to this contradiction in result, we found that cell-scaffold interaction in PLLA/PCL for proximity adjacent physiological conditions to beta-like cells is better than PCL/PVA and PLLA/PVA scaffolds and altered due to the cellular signalling and extracellular matrix and in confirmation of our study several reports [Citation39,Citation40] have described the role of ECM in islet differentiation. Our study has limitations, although we analysed the potency of IPS Cell-derived Insulin-Producing Islet-Like cells on PLLA/PCL scaffolds, the high cost and time didn’t allow our team to check the differentiation potency of PLLA and PCL separately. Also more research is needed to obtain a good biodegradable, efficient and desirable methods for in vivo transplantation.
Conclusions
We introduced a new approach for the differentiation of hiPSCs to insulin-producing cells by PLLA/PCL scaffold that is highly flexible for the first time. These finding suggest that functional matured induction cells on PLLA/PCL scaffold can be used as a successful method for soft tissue engineering of islet beta cell therapy and regenerative medicine.
SUPPLEMENTED.docx
Download MS Word (17.6 KB)Acknowledgements
Also, authors would like to thank the Stem Cell Technology Research Center for the use of their laboratory facilities.
Disclosure statement
No potential conflict of interest was reported by the authors.
Additional information
Funding
References
- Mazidi M, Speakman JR. Association of fast-food and full-service restaurant densities with mortality from cardiovascular disease and stroke, and the prevalence of diabetes mellitus. J Am Heart Assoc. 2018;7:e007651.
- Jafarian A, Taghikhani M, Abroun S, et al. Generation of high-yield insulin producing cells from human bone marrow mesenchymal stem cells. Mol Biol Rep. 2014;41:4783–4794.
- Wang A, Sander M. Generating cells of the gastrointestinal system: current approaches and applications for the differentiation of human pluripotent stem cells. J Mol Med. 2012;90:763–771.
- Amer LD, Mahoney MJ, Bryant SJ. Tissue engineering approaches to cell-based type 1 diabetes therapy. Tissue Eng Part B Rev. 2014;20:455–467.
- Kim D, Gu Y, Ishii M, et al. In vivo functioning and transplantable mature pancreatic islet-like cell clusters differentiated from embryonic stem cell. Pancreas. 2003;27:e34–e41.
- Inoue K, Miyamoto M. Islet transplantation. J Hepatobiliary Pancreatic Surg. 2000;7:163–177.
- Mansour RN, Barati G, Soleimani M, et al. Generation of high-yield insulin producing cells from human-induced pluripotent stem cells on polyethersulfone nanofibrous scaffold. Artif Cells Nanomed Biotechnol. 2018;2:1–7.
- Wang W, Gu Y, Tabata Y, et al. Reversal of diabetes in mice by xenotransplantation of a bioartificial pancreas in a prevascularized subcutaneous site. Transplantation. 2002;73:122–129.
- Hashemi SM, Soudi S, Shabani I, et al. The promotion of stemness and pluripotency following feeder-free culture of embryonic stem cells on collagen-grafted 3-dimensional nanofibrous scaffold. Biomaterials. 2011;32:7363–7374.
- Jacobson EF, Tzanakakis ES. Human pluripotent stem cell differentiation to functional pancreatic cells for diabetes therapies: Innovations, challenges and future directions. J Biol Eng. 2017;11:21.
- Mashhadikhan M, Soleimani M, Parivar K, et al. ADSCs on PLLA/PCL Hybrid nanoscaffold and gelatin modification: cytocompatibility and mechanical properties. Avicenna J Med Biotechnol. 2015;7:32–38.
- Fazili A, Gholami S, Minaie Zangi B, et al. In vivo differentiation of mesenchymal stem cells into insulin producing cells on electrospun poly-l-lactide acid scaffolds coated with matricaria chamomilla L. oil. Cell Journal 2016;18:310–321.
- Enderami SE, Kehtari M, Abazari MF, et al. Generation of insulin-producing cells from human induced pluripotent stem cells on PLLA/PVA nanofiber scaffold. Artif Cells Nanomed Biotechnol. 2018;2:1–8.
- Abazari MF, Soleimanifar F, Nouri Aleagha M, et al. PCL/PVA nanofibrous scaffold improve insulin-producing cells generation from human induced pluripotent stem cells. Gene 2018;5:50–57. doi: 10.1016/j.gene.2018.05.115.
- Shabani I, Haddadi-Asl V, Soleimani M, et al. Enhanced infiltration and biomineralization of stem cells on collagen-grafted three-dimensional nanofibers. Tissue Eng A. 2011;17:1209–1218.
- Bakhshandeh B, Soleimani M, Ghaemi N, et al. Effective combination of aligned nanocomposite nanofibers and human unrestricted somatic stem cells for bone tissue engineering. Acta Pharmacol Sin. 2011;32:626–636.
- Wright-Charlesworth DD, King JA, Miller DM, et al. In vitro flexural properties of hydroxyapatite and self-reinforced poly(L-lactic acid). J Biomed Mater Res. 2006; Sep 178A: 541–549.
- Gaaz TS, Sulong AB, Akhtar MN, et al. Properties and applications of polyvinyl alcohol, halloysite nanotubes and their nanocomposites. Molecules (Basel, Switzerland). 2015;20:22833–22847.
- Chen L, Bai Y, Liao G, et al. Electrospun poly(L-lactide)/poly(epsilon-caprolactone) blend nanofibrous scaffold: characterization and biocompatibility with human adipose-derived stem cells. PloS One. 2013;8:e71265.
- Basford CL, Prentice KJ, Hardy AB, et al. The functional and molecular characterisation of human embryonic stem cell-derived insulin-positive cells compared with adult pancreatic beta cells. Diabetologia. 2012;55:358–371.
- Carolan PJ, Melton DA. New findings in pancreatic and intestinal endocrine development to advance regenerative medicine. Curr Opin Endocrinol Diabetes Obes. 2013;20:1–7.
- Shim JH, Kim SE, Woo DH, et al. Directed differentiation of human embryonic stem cells towards a pancreatic cell fate. Diabetologia. 2007;50:1228–1238.
- Moradi SL, Golchin A, Hajishafieeha Z, et al. Bone tissue engineering: adult stem cells in combination with electrospun nanofibrous scaffolds. J Cell Physiol. 2018;10:6509–6522.
- Rezaie Z, Ardeshirylajimi A, Ashkezari MD. Improved anticancer properties of stem cells derived exosomes by prolonged release from PCL nanofibrous structure. Gene. 2018;665:105–110.
- Mahdavi FS, Salehi A, Seyedjafari E, et al. Bioactive glass ceramic nanoparticles-coated poly(l-lactic acid) scaffold improved osteogenic differentiation of adipose stem cells in equine. Tissue & Cell. 2017;49:565–572.
- Mobarra N, Soleimani M, Kouhkan F, et al. Efficient Differentiation of Human Induced Pluripotent Stem Cell (hiPSC) Derived Hepatocyte-Like Cells on hMSCs Feeder. Int J Hematol Oncol Stem Cell Res. 2014;8:20–29.
- Fishman B, Segev H, Kopper O, et al. Targeting pancreatic progenitor cells in human embryonic stem cell differentiation for the identification of novel cell surface markers. Stem cell. Stem Cell Rev and Rep. 2012;8:792–802.
- Zhang D, Jiang W, Liu M, et al. Highly efficient differentiation of human ES cells and iPS cells into mature pancreatic insulin-producing cells. Cell Res. 2009;19:429–438.
- Liu Y, Cui H, Zhuang X, et al. Electrospinning of aniline pentamer-graft-gelatin/PLLA nanofibers for bone tissue engineering. Acta Biomaterialia. 2014;10:5074–5080.
- Ge Z, Yang F, Goh JC, et al. Biomaterials and scaffolds for ligament tissue engineering. J Biomed Mater Res. 2006;77A:639–652. Jun 1 doi:10.1002/jbm.a.30578.
- Aloysious N, Nair PD. Enhanced survival and function of islet-like clusters differentiated from adipose stem cells on a three-dimensional natural polymeric scaffold: an in vitro study. Tissue Eng A. 2014; May20:1508–1522.
- Zhang Y, Ouyang H, Lim CT, et al. Electrospinning of gelatin fibers and gelatin/PCL composite fibrous scaffolds. J Biomed Mater Res. 2005;72B:156–165.
- Qi H, Ye Z, Ren H, et al. Bioactivity assessment of PLLA/PCL/HAP electrospun nanofibrous scaffolds for bone tissue engineering. Life Sci. 2016;148:139–144
- Birhanu G, Akbari Javar H, Seyedjafari E, et al. An improved surface for enhanced stem cell proliferation and osteogenic differentiation using electrospun composite PLLA/P123 scaffold. Artif Cell Nanomed Biotechnol. 2018;46:1274–1281.
- Shakhssalim N, Soleimani M, Dehghan MM, et al. Bladder smooth muscle cells on electrospun poly(epsilon-caprolactone)/poly(l-lactic acid) scaffold promote bladder regeneration in a canine model. Mater Sci Eng C Mater Biol Appl. 2017;75:877–884.
- Jaafarpour Z, Soleimani M, Hosseinkhani S, et al. Differentiation of definitive endoderm from human induced pluripotent stem cells on hmscs feeder in a defined medium. Avicenna J Med Biotechnol. 2016;8:2–8.
- Lahmy R, Soleimani M, Sanati MH, et al. MiRNA-375 promotes beta pancreatic differentiation in human induced pluripotent stem (hiPS) cells. Mol Biol Rep. 2014;41:2055–2066.
- Mahmoodinia Maymand M, Soleimanpour-Lichaei HR, Ardeshirylajimi A, et al. Improvement of hepatogenic differentiation of iPS cells on an aligned polyethersulfone compared to random nanofibers. Artif Cell Nanomed Biotechnol. 2018;46:853–860.
- Stendahl JC, Kaufman DB, Stupp SI. Extracellular matrix in pancreatic islets: relevance to scaffold design and transplantation. Cell Transplant. 2009;18:1–12.
- Bosco D, Meda P, Halban PA, et al. Importance of cell-matrix interactions in rat islet beta-cell secretion in vitro: role of alpha6beta1 integrin. Diabetes 2000;49:233–243.