Abstract
Wharton’s jelly-derived extracellular matrix (WJ-ECM) has attracted researcher’s attention for its biomedical applications. Previously, we fabricated a biomimetic spongy scaffold from decellularized WJ-ECM and, in this study, we sought to examine the osteogenic inductive potential of this scaffold and its underlying mechanism. To address this question, mesenchymal stem cells (MSCs) were isolated from WJ using a mechanical method and cultured on the scaffold, under dynamic condition, for over 21 days in the presence or absence of osteogenic medium. The status of signalling pathways involved in the osteogenic differentiation and the expression profile of integrins in the WJ-derived MSCs (WJ-MSCs) were examined. WJ-MSCs displayed differentiation capacities and expressed surface antigens, characteristics of MSCs. Histologically, WJ-MSCs seeded on the scaffold showed a proper cellular attachment, penetration and migration. They also exhibited a higher degree of alkaline phosphatase activity, calcium deposition and osteogenic gene expression, than those cultured in 2D condition. The expression of Wnt, BMP and TGF-β signalling target genes together with that of α2, αv and β1 integrins was increased in WJ-MSCs in both presence and absence of osteogenic induction medium. Taken together, our results demonstrate that WJ-derived scaffold induces osteogenic differentiation of WJ-MSCs, possibly through activating integrins and subsequently conventional intracellular signalling pathways.
Introduction
The development of tissue engineering has made it an attractive method with great repairing potential for tissue defects. Bone tissue engineering techniques based on autogenously cell/tissue transplantation would eliminate problems of donor compatibility, limitation of autograft implantation, pathogen transfer and immune response of allograft bone transplantation [Citation1]. The bone tissue engineering requires cellular components, scaffolds and growth factors. The scaffold provides the initial extracellular matrix (ECM) required to support the cells adhesion, proliferation and differentiation [Citation2]. Currently, the scientific challenges of bone tissue engineering are to develop suitable three-dimensional (3D) biodegradable and biocompatible scaffolds that easily are fabricated into a variety of shapes and sizes with desirable mechanical properties and interconnecting porosity [Citation3,Citation4].
Currently, Wharton’s jelly-derived extracellular matrix (WJ-ECM) has attracted researcher’s attention for its biomedical applications [Citation5–7]. Since, similar to bone, the major matrix protein in WJ-ECM is type I collagen [Citation8], it could have positive effects on bone tissue engineering. Moreover, WJ-ECM also contains significant amounts of hyaluronic acid, GAGs and various bioactive molecules like, acidic and basic fibroblast growth factors like (aFGF, bFGF), insulin growth factor-I (IGF-I), platelet-derived growth factor (PDGF), epidermal growth factor (EGF), transforming growth factor beta 1 (TGF-β1) and other peptide growth factors [Citation9] which could enhance osteogenic differentiation.
Recently, we fabricated a new biomimetic spongy scaffold from decellularized WJ-ECM using freeze-dried technique and demonstrated that these biocompatible scaffolds are able to improve cellular attachment, penetration, and proliferation and accelerate the wound healing process in full thickness wound healing model [Citation7].
The other important aspect of bone tissue engineering is choosing an ideal cell source with the high capacity of differentiating into osteoblasts. Mesenchymal stem cells (MSCs) have been demonstrated as an available source for tissue-engineering applications due to their multiple differentiation capabilities to diverse tissues (bone, cartilage, adipose tissue, muscle, etc.) [Citation10]. Among MSCs sources, WJ-MSCs that are perinatal stem cells and show an intermediate state between embryonic stem cells (ESCs) and adult MSCs, reflect ideal candidates for bone tissue engineering [Citation11]. Compared to other sources of MSCs, WJ-MSCs have some advantages, such as easy isolation and in vitro expansion, high proliferation rates and differentiation potential, minimal immune reactivity and immune modulatory effects [Citation12]. Therefore, the focus of the current study was to examine the osteogenic differentiation of WJ-derived mesenchymal stem cells (WJ-MSCs) on WJ-derived scaffolds under dynamic conditions.
The osteogenic differentiation of MSCs has been shown to take place in four steps including osteoprogenitor cells, pre-osteoblasts, osteoblasts and osteocytes that correspond to the processes of proliferation, maturation, matrix synthesis and matrix mineralization phases, respectively [Citation13]. Each step or phase was shown to depend on the activity of multiple signalling pathways, including Wnt, TGF-β and BMP signalling pathways [Citation14]. There are reports indicating that Wnt pathway promotes the progression of MSCs from pre-osteoblasts into more mature osteoblasts [Citation15]. BMP pathway was shown to promote osteogenic commitment and also induce terminal osteogenic differentiation in MSC [Citation16] and TGF-β signalling stimulates proliferation, early differentiation, and commitment of osteoprogenitors into the osteoblastic lineage [Citation14].
In bone formation, the ECM plays an important role in osteoblast differentiation and function. Integrins are involved in cell-ECM interaction and transmit the signals to cytosol to activate kinases and signalling pathways including those involved in osteogenic differentiation [Citation17]. Human MSCs have been reported to express α1–6, αV and β1, 3 and 4 integrin [Citation18] which is different between MSCs of various sources [Citation19]. It has been demonstrated that several integrins have important role in MSCs osteogenic differentiation. The β1 integrin seems to be important for the differentiation of hMSCs into osteoblasts and mineralization of matrix [Citation20], α2 integrin is up-regulated during osteogenic differentiation [Citation21] and the expression of α2β1 integrin is increased during commitment of hMSCs towards osteoblast fate [Citation22]. Several studies have shown that MSCs have the ability to change the integrin expression profile in 3D matrices compared to 2D beds [Citation23], highlighting the importance of choosing appropriate ECM for the cell culture and differentiation.
Having mentioned the importance of cellular signalling response to the 3D scaffolds, there is no much information available. Therefore, in addition to examining osteogenic induction capacity of our newly fabricated biomimetic scaffold, we also investigated the signalling mechanism responsible for osteogenic differentiation of WJ-MSCs cultured on this scaffold.
Materials and methods
Fabrication of WJ-derived scaffold
WJ-ECM was obtained, decellularized and characterized as described [Citation7]. For more details, see supplementary data.
WJ-derived stem cells isolation and characterization
UCs were washed in sterile PBS to remove blood cells contamination, and then were cut into 1–2 cm pieces. Umbilical arteries and vein were removed and WJ explants transferred to a 50 ml tube containing PBS. See supplementary data for details for WJ-MSCs isolation and osteogenic, chondrogenic and adipogenic differentiation protocols.
WJ-MSCs surface markers expression
1 × 105 WJ-MSCs were incubated for 45 min with the following monoclonal antibodies including mouse anti-human CD105-PE, CD90-FITC, CD44-PE, CD73-PE, CD34/45-PE/FITC, CD133-PE and HLA DR-PE (all from Becton Dickinson, Franklin Lakes, NJ). After washing with PBS, cells were analysed in FACSCalibur system (BD Biosciences, San Jose, CA) by flowing software.
Cell seeding
A suspension of 2 × 105 WJ-MSCs in 100 µl FBS was seeded on the WJ-derived scaffold and incubated for 3 h at 37 °C to allow for cell attachment, then 1.5 ml of appropriate medium was added to each scaffold and left overnight in the incubator before being placed in dynamic condition. For more details, see supplementary data.
Cell attachment and migration
Cell migration into the scaffolds was detected by Hoechst 33344 (Sigma, St. Louis, MO) staining on 6 µm thick cross sections of scaffolds (see supplementary data for details).
Alkaline phosphatase (ALP) activity and calcium content assay
ALP activity and calcium content assay of each WJ-MSCs/scaffold construct were measured at day 7, 14 and 21 using Alkaline Phosphatase Assay kit (Pars Azmoon, Tehran, Iran) and Calcium Content Assay kit (Pars Azmoon, Tehran, Iran), respectively (see supplementary data for details).
Scanning electron microscopy
Matrix mineralization of WJ-MSCs/scaffold construct was observed by scanning electron microscope (SEM) analysis (Ais2300, Seron, Uiwang-si, Korea) (see supplementary data for details).
Mechanical properties of WJ-MSCs/scaffold construct
Mechanical (compressive) property of MSCs/scaffold constructs at day 7, 14 and 21 of culture in growth and osteogenic medium was determined using a universal load test machine (Santam, stm20, Korea). For compressive test, cylindrical-shaped specimens with approximately 4 mm in diameter and 8 mm in length were conducted at 2 mm/min crosshead speed.
Real-time quantitative PCR analysis (qPCR)
The total RNA of WJ-MSCs seeded on the scaffolds and 2D culture plates was extracted using RNeasy Mini Kit (Qiagen, Hilden, Germany) at day 7, 14 and 21 of culture in osteogenic and growth medium (see supplementary data for details) ().
Statistical analysis
One-way analysis of variance (ANOVA) was used for calculating statistically significant differences between groups and t test used for the individual groups. All experimental data were reported as mean ± standard deviation (SD) (n = 5) and p values of less than .05 were accepted as significant.
Results
A spongy scaffold was made from decellularized WJ-ECM using freeze-dried technique. The characterization of the scaffolds showed heteroporous 3D structure with high degree of interconnectivity (data not shown) [Citation7].
WJ-derived MSCs characterization
WJ-derived stem cells after five days culture in DMEM-LG showed typical MSCs characteristics and displayed spindle-shaped with fibroblastic morphology when adhered to culture plate. WJ-MSCs had ability to differentiate into adipogenic, chondrogenic and osteogenic lineages, furthermore expressed mesenchymal CD markers (CD105, CD44, CD90 and CD73) and lacked the expression of CD45, CD34, CD133 and HLA-DR ().
Figure 1. Characterization of WJ-MSCs. (A) Photomicrograph of MSCs with spindle-shaped morphology after five days of culture, (B) MSCs monolayer at approximately 90% confluence in P2. (C) MSCs colonies (black arrows) in P3. (D) Osteogenic differentiation of P3 MSCs confirmed by alizarin red staining at day 21. (E) Adipogenic differentiation of P3 MSCs confirmed by oil red staining at day 21. (F) Chondrogenic differentiation of P3 MSCs confirmed by Alcian blue at day 21. (G) Flow cytometry analysis of WJ-MSCs surface CD markers expression.
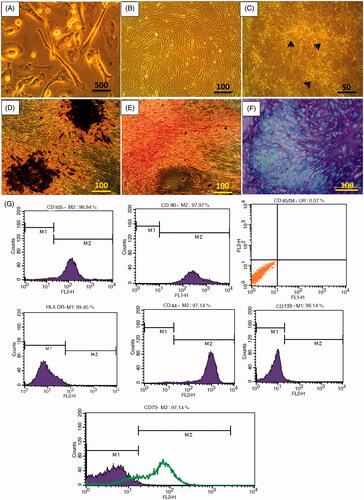
Cellular attachment and migration of WJ-MSCs on the scaffold
WJ-MSCs attachment and migration into the scaffold were detected by fluorescence microscopy on serial 6 µm thick cross sections. At day 3, the MSCs were mainly on the surface of the scaffold (. At day 7, the MSCs had remained mostly on the top of the scaffold, with only a few cells penetrating the scaffold (. The MSCs had largely migrated into the scaffold at day 14 ( and by day 21, the MSCs seeded on the scaffolds were distributed throughout the entire scaffold (.
Figure 2. WJ-MSCs migration within the scaffolds in growth medium. (X) The 3D spongy scaffolds fabricated from WJ-derived ECM (15 mm diameter, 3 mm thickness). (A–D) Histological sections of fluorescent photomicrographs stained with Hoechst 33344 at day 3 (A), 7 (B), 14 (C) and 21 (D). Note that the cells are distributed (arrows) on the surface and inside the scaffolds during three weeks of culture. Scale bars: 25 µm in A and B; 50 µm in C and D.
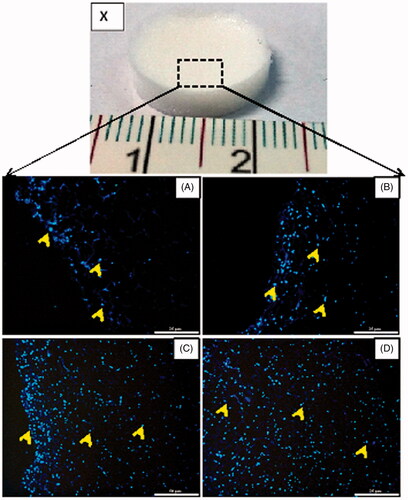
Differentiation and mineralization of WJ-MSCs
The alkaline phosphatase (ALP) activity is an indicator of cells commitment towards the osteoblastic lineage. WJ-MSCs were cultured in the presence of osteogenic medium in static or dynamic conditions for 21 days after which the ALP activity was examined and compared with TCP as control. As shown in , the ALP activity of the cells cultured on TCP and scaffold in dynamic condition achieved maximal levels in day 14 and 21 (there is no significant difference between day 14 and 21). However, the ALP activity on scaffold in static condition did not show significant changes during time course of osteogenic differentiation. Notably, at each time point in WJ-MSC differentiation, the ALP activity of the cells on the scaffold in dynamic condition was significantly higher compared to those in other groups (p < .05).
Figure 3. Alkaline phosphatase (ALP) activity of WJ-MSCs. ALP activity was assayed in all groups (TCP and scaffold in static and dynamic conditions) for over 21 days in the presence of osteogenic medium. Note that the ALP activity on the scaffold in dynamic condition was significantly higher than two other groups (*p < .05).
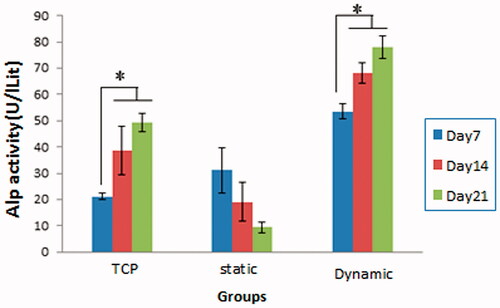
The calcium deposition, the indicator of fully matured osteoblastic cells, was also examined in WJ-MSCs cultured in osteogenic medium for 21 days. Confirming the above results, cells cultured on the scaffolds in dynamic condition had the highest calcium deposition on day 21 (. The calcium deposition in the scaffold in static condition had no significant difference from day 7 to day 21. These results together indicate that the scaffold in static condition was not able to induce osteogenic differentiation in WJ-MSCs, therefore, it was excluded from the rest of experiments.
Figure 4. Calcium deposition in WJ-MSCs/scaffold constructs during the 21 days culture period in the three groups (TCP, scaffold in static condition, and scaffold in dynamic condition). Note that the calcium deposition on the scaffold in dynamic condition was significantly higher compared with two other groups (*p < .05), while on the scaffold in static had no significant difference during the 21 days.
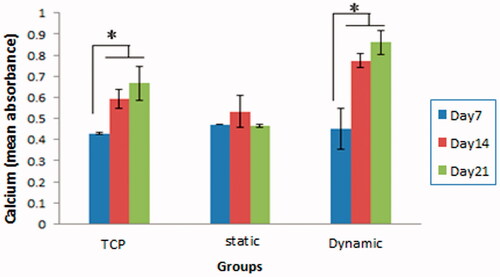
SEM analysis of matrix mineralization
The matrix mineralization of WJ-MSCs on the scaffolds in growth and osteogenic media was evaluated using SEM. At day 7 of culture, the cells showed attachment and spreading behaviour in both media (). However, at day 14 and 21, the MSCs filled the scaffolds pores with mineral depositions only in osteogenic medium (). At a higher magnification, large and rounded aggregations were visible on the scaffolds in osteogenic medium () as compared to the scaffolds in growth medium ().
Figure 5. SEM micrographs of WJ-MSCs mineralization on the scaffolds in the presence of growth and osteogenic medium at day 7, 14 and 21. The MSCs are attached and spread on the scaffolds on both groups (A and E), however, only in osteogenic medium they filled the scaffold pores with mineral depositions (F, G). Note that aggregates (most probably calcium salts) are deposited on the scaffold only by MSCs culture in the presence of osteogenic medium (H). Scale bars: 500 µm in A–C and E–G; 3 µm in D and E.
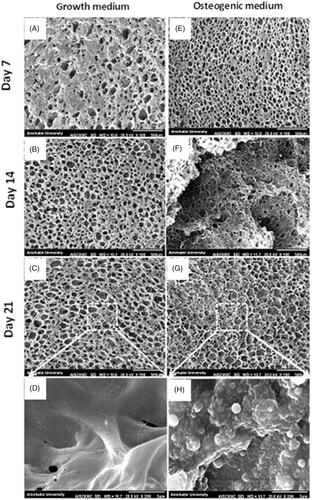
Mechanical properties of WJ-MSCs/scaffold constructs
Compressive modulus of WJ-MSCs/scaffold constructs in growth and osteogenic medium was measured at day 7, 14 and 21 of culture (). The highest compressive modulus was measured on the scaffold in osteogenic medium at day 21 (0.71 ± 0.04 MPa) that was 4.7 fold increase compared to that of the scaffold in growth medium (*p < .05). This result indicates that the mechanical properties of the scaffolds are improved by osteogenic differentiation of MSCs due to the matrix mineralization.
Expression of osteogenic specific genes
The relative expression for the bone associated gene Runx 2, ALP, osteocalcin (OCN) and osteopontin (OPN) was performed by qPCR analysis in WJ-MSCs cultured on the scaffolds in growth and osteogenic medium. When growth medium was used (), the expression of all osteogenic markers was increased in the cells cultured on the scaffolds up to 28 times more than TCP. In the case of differentiation medium (), as expected, this increased level of gene expression was up to 70 folds. These results clearly indicate that the scaffold significantly increase the osteogenic differentiation of WJ-MSCs even in the absence of osteogenic induction medium.
Figure 7. Expression of osteogenic specific genes in WJ-MSCs cultured on the scaffolds in growth (A) and osteogenic medium (B). The cells were cultured on the scaffold or TCP for over 21 days in osteogenic medium after which expression of the osteogenic specific marker was examined using real-time PCR. The mRNA relative expression of osteogenic genes in MSCs cultured in growth and osteogenic medium was clearly higher than that on TCP (*p < .05, **p < .01). OCN: osteocalcin; OPN: osteopontin; ALP: alkaline phosphatase.
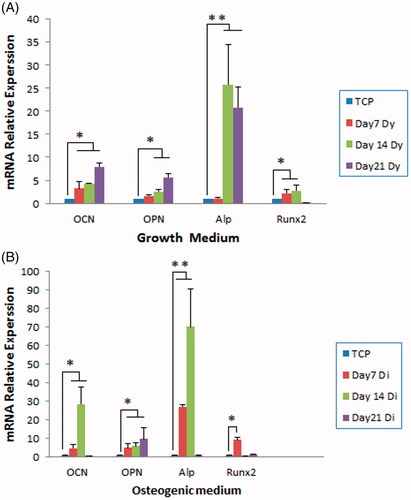
Expression of signalling pathways target genes in WJ-MSCs
To investigate the intracellular mechanism by which the scaffold enhances osteogenic differentiation of WJ-MSCs, the status of Wnt, BMP and TGF-β signalling pathways, known to involve in osteoblast differentiation, was evaluated in the presence of osteogenic induction medium. To address this question, the expression of target genes of these signalling pathways was examined in the cells on day 7, 14 and 21 of culture using qPCR analysis. As shown in , the expression of cyclin D1 and c-Myc (Wnt target genes) was significantly highest at day 14 compared to TCP (p < .05). The highest expression of Id1 (BMP target gene) and pai and smad 7 (TGF-β target genes) was observed at day 7 (p < .05). These results indicate differential activities of these signalling pathways during osteogenic differentiation of WJ-MSCs.
Figure 8. Expression of Wnt, BMP and TGF-β signalling target genes in WJ-MSCs. The cells were cultured on the scaffold or TCP for over 21 days in osteogenic medium after which expression of the target genes was examined using real-time PCR. The expression of C-myc and Cyclind1 (Wnt target genes) was clearly higher than TCP in the second week. The expression of Id1 (BMP target gene) and also Smad7 and Pai (TGF-β target genes) was the highest in the first week of osteogenic differentiation compared to that of TCP. Dy: dynamic condition (*p < .05, **p < .01).
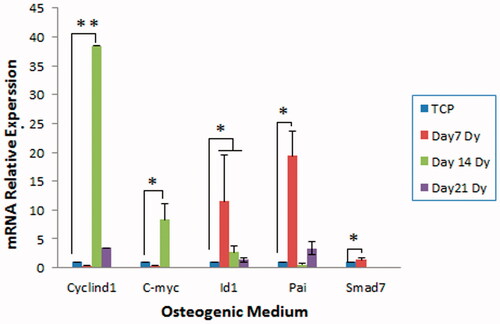
To investigate the effects of the scaffold alone on the activities of these signalling pathways, the expression of their target genes was examined in WJ-MSCs after seven days of culture in the absence of osteogenic medium. The effects of the scaffold alone on osteogenic differentiation of WJ-MSCs showed that the expression of all target genes (except of pai) increased significantly compared to that of TCP (p<.05) (. These results indicate the positive role of the scaffold in activating of the signalling pathways involved in osteogenic differentiation without the inducing factors.
Figure 9. Expression of Wnt, BMP and TGF-β signalling target genes in WJ-MSCs cultured on scaffold in the absence of osteogenic induction medium. The cells were cultured on the scaffold or TCP for seven days in growth medium after which expression of the target genes were examined using real-time PCR. The expression of all target genes (except of Pai) was clearly higher than that in TCP. D: dynamic condition (*p < .05, **p < .01).
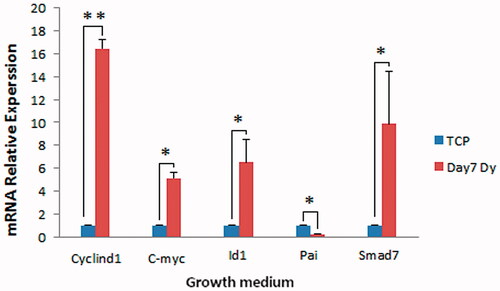
Expression of integrins in WJ-MSCs
Integrins have been shown to mediate cell adhesion and attachment and also to regulate multiple intracellular signalling pathways [Citation24]. Therefore, the expression of several integrin genes including a2, av, a5, a6 and β1 involved in osteogenic differentiation [Citation25,Citation26], was examined in WJ-MSCs after seven days of culture on the scaffold with or without osteogenic medium. In the presence of osteogenic medium and dynamic condition, the expression of all integrins, except the integrin α6, showed a significant increase compared to that of TCP () (p < .05). In the absence of osteogenic medium (but the presence of growth medium), significant increase in the expression of α2, αv and β1 integrins was still observed compared to that of TCP () (p<.05). These results indicate that, regardless of the presence or absence of osteogenic induction medium, the scaffold induces expression of the integrins involved in osteogenic differentiation in WJ-MSCs.
Figure 10. Expression of integrins in the WJ-MSCs cultured on the scaffold in the growth (A) or osteogenic medium (B) under dynamic conditions (Dy). The cells were cultured on the scaffold or TCP for seven days after which expression of integrins was examined using real-time PCR. The expression of α2, αv and β1 integrins was clearly higher than that in TCP in both osteogenic and growth media after seven days of culture. Dy: dynamic condition (*p < .05, **p < .01).
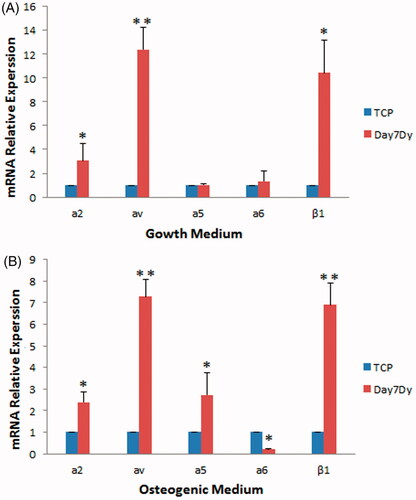
Discussion
The tissue-derived acellular ECM as a natural material has attracted researcher’s attention because of its valuable source of growth factors and bioactive molecules that provide ideal substrate for tissue engineering and regenerative medicine [Citation27–29]. Many researchers are seeking to use human tissue-derived ECM as a scaffold for clinical purposes to overcome the risk of immunological reactions and pathogen transmission of animal tissue-derived materials [Citation30].
Previously, we introduced the WJ-ECM as an ideal source of natural material for fabrication of biocompatible spongy scaffold and used it as an acceptable skin graft for wound healing [Citation7]. Since, similar to bone, the major matrix protein in WJ-ECM is type I collagen [Citation8], it could provide ideal substrate for bone tissue engineering. Here, we examine the ability of our new biomimetic spongy scaffold to induce osteogenic differentiation of WJ-MSCs and investigate the signalling pathways involving this differentiation.
In the present study, WJ-MSCs were used to evaluate the cellular response to the scaffold in osteogenic medium and dynamic conditions. Like other researchers [Citation31–34], MSCs were isolated from the WJ using a mechanical method to avoid decreased cellular viability, degradation of cellular surface receptors, and changed cellular function. Here, we describe, for the first time, a simplified mechanical method to obtain a sufficient number of MSCs from all umbilical cord samples, without enzymatic treatment. Moreover, these MSCs exhibited functional properties and expression of surface antigen markers similar to MSCs. Our results further revealed that the WJ-MSCs cultured on WJ-scaffold exhibited a high degree of attachment and migration. The SEM images demonstrated that all pores of scaffold on day 21, was filled by the mineral depositions. Analysis of the ALP activity and matrix mineralization showed that WJ-MSCs cultured on the scaffold were more differentiated in comparison to TCP. Moreover, our results indicated that the increased osteogenic differentiation of WJ-MSCs was observed when these cells were cultured in the dynamic conditions and not in static condition. This increased osteogenic differentiation in response to dynamic conditions is consistent with the other reports [Citation35,Citation36], and might be as a result of the convective transport of nutrients to the surface of the scaffold, in contrast to the purely diffusional transport in static culture, increasing concentration of oxygen throughout the scaffold [Citation37].
To investigate the role of the scaffold alone, on the osteogenic differentiation of WJ-MSCs and its underlying mechanisms, these cells were cultured on the scaffolds in the dynamic conditions and in the presence or absence of osteogenic inductive medium. In the presence of the medium, the expression of both early (Runx2, highest at day 7 and ALP, highest at day 14) and late specific osteogenic gene markers (OCN highest at day 14 and OPN, highest at day 21) was significantly increased in the cells cultured on the scaffold compared to that of TCP. The status of osteogenic signalling pathways (by examining the expression of their target genes) showed that while highest activity of TGFβ and BMP signalling was observed at day 7, Wnt/β-catenin signalling displayed highest activity at day 14. This activity pattern of Wnt, TGFβ and BMP signalling pathways during osteogenic differentiation has already been reported [Citation38–43]. The expression of integrins, as the signal transducer from the ECM to the intracellular signalling pathways, was also examined in the WJ-MSCs. Our results demonstrated that the expression of α2, αv, α5 and β1 integrins was significantly increased in the cells cultured on the scaffold compared to that of TCP. These results indicate that, in the presence of osteogenic medium, differentiation of WJ-MSCs cultured on the scaffold is improved compared to that of TCP, most probably through conventional signalling mechanisms. Consistent with this conclusion, it was reported that the interaction of cell surface integrins with collagenous compounds of scaffolds activated signalling pathways inducing osteogenic specific genes expression [Citation44,Citation45].
In the absence of osteogenic inductive medium, interestingly, the expression of all osteogenic gene markers was increased, in all time point, in WJ-MSCs cultured on the scaffold compared to that of TCP. This result indicates that our fabricated scaffold induces osteogenic differentiation of WJ-MSCs even in the absence of osteogenic induction medium. The roles of collagenous scaffolds to induce osteogenic differentiation in the absence of inducing factor was also reported by Byrne et al. who examined the expression of osteogenic genes in MSCs cultured on a cow collagen-glycosaminoglycan-derived scaffold, and indicated that collagen-based scaffolds are capable to induce bone gene expression in the absence of bone induction factors [Citation46]. As we expected, the expression of the integrins (α2, αv and β1) was also increased in the MSCs cultured on the WJ-scaffold in the absence of osteogenic inductive medium. Generally, it is well accepted that 3D scaffolds increase expression or activation (dimerization) of integrins [Citation47,Citation48]. More specifically, it has been reported that the binding of MSCs through their α2β1 integrins to collagen type I based substrates is sufficient to induce bone differentiation, even in the absence of bone induction factors [Citation44,Citation49]. Therefore, our results are consistent with the other reports [Citation20,Citation21] that collagen based matrix increases the differentiation of osteoblasts by interacting with integrins.
The signalling mechanism by which the 3D scaffolds affect stem cell differentiation is not well understood. It is well known that Wnt, BMP and TGFβ signalling pathways are major player in osteogenic differentiation in vitro and in vivo [Citation14]. Interestingly, we observed a remarkable increase in the activity of these signalling pathways (in a different pattern, summarized in ) in the MSCs, in the absence of osteogenic inductive medium, indicating that our fabricated scaffold improves the osteogenic differentiation of WJ-MSCs through the conventional signalling mechanisms.
Figure 11. Schematic image of signalling pathways that regulates the key stages of bone differentiation. The BMP pathway plays a role in all stages of MSCs differentiation into osteocytes. TGF-β plays an inductive role in the proliferation of osteoprogenitors and their early differentiation into immature osteoblasts; however, it has also an inhibitory role in the further differentiation of immature osteoblasts through inhibiting bone matrix mineralization. Wnt signalling pathway induces immature osteoblasts into mature osteoblasts.
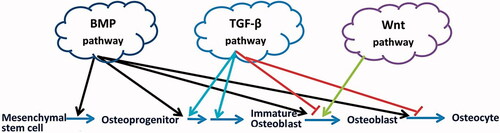
Conclusions
In the present study, we described for the first time a simplified mechanical method to obtain a sufficient number of MSCs from the WJ samples with minimum time consuming. These MSCs were then cultured on the WJ-derived scaffold to examine whether the scaffold enhances their osteogenic differentiation. Our results showed that WJ-derived spongy scaffolds increased the osteoblastic differentiation of the MSCs compared to TCP, in the presence or absence of bone induction factors, confirming the positive effect of the scaffold on bone differentiation possibly through providing more surface area and adhesion sites for the cells. The increased expression of integrins, and Wnt, TGF-β and BMP signalling target genes even in the absence of osteogenic induction medium suggest that activation of integrins and subsequently these signalling pathways, by the scaffold, could be sufficient to induce osteogenic differentiation WJ-MSCs. Further experiments are needed to clarify more precisely the role of integrins on osteogenic differentiation of MSCs and to investigate how scaffold-activated integrins induce signalling pathways involved in osteogenic differentiation.
Disclosure statement
No potential conflict of interest was reported by the author(s).
Table 1. Primers used for real-time PCR analysis.
Additional information
Funding
References
- Liu X, Ma PX. Polymeric scaffolds for bone tissue engineering. Ann Biomed Eng. 2004;32:477–486.
- Porter JR, Ruckh TT, Popat KC. Bone tissue engineering: a review in bone biomimetics and drug delivery strategies. Biotechnol Prog. 2009;25:1539–1560.
- Xu C, Su P, Chen X, et al. Biocompatibility and osteogenesis of biomimetic bioglass–collagen–phosphatidylserine composite scaffolds for bone tissue engineering. Biomaterials. 2011;32:1051–1058.
- Le Pape F, Richard G, Porchet E, et al. Adhesion, proliferation and osteogenic differentiation of human MSCs cultured under perfusion with a marine oxygen carrier on an allogenic bone substitute. Artif Cells Nanomed Biotechnol. 2018;46:95–107.
- Stocco E, Barbon S, Radossi P, et al. Autologous chondrocytes as a novel source for neo-chondrogenesis in haemophiliacs. Cell Tissue Res. 2016;366:51–61.
- Herrero-Mendez A, Palomares T, Castro B, et al. HR007: a family of biomaterials based on glycosaminoglycans for tissue repair. J Tissue Eng Regen Med. 2015;11:989–1001.
- Beiki B, Zeynali B, Seyedjafari E. Fabrication of a three dimensional spongy scaffold using human Wharton's jelly derived extra cellular matrix for wound healing. Mater Sci Eng C Mater Biol Appl. 2017;78:627–638.
- Nanaev AK, Kohnen G, Milovanov AP, et al. Stromal differentiation and architecture of the human umbilical cord. Placenta. 1997;18:53–64.
- Sobolewski K, Małkowski A, Bańkowski E, et al. Wharton's jelly as a reservoir of peptide growth factors. Placenta. 2005;26:747–752.
- Mauney JR, Volloch V, Kaplan DL. Role of adult mesenchymal stem cells in bone tissue engineering applications: current status and future prospects. Tissue Eng. 2005;11:787–802.
- Kim DW, Staples M, Shinozuka K, et al. Wharton's jelly-derived mesenchymal stem cells: phenotypic characterization and optimizing their therapeutic potential for clinical applications. IJMS. 2013;14:11692–11712.
- La Rocca G, Lo Iacono M, Corsello T, et al. Human Wharton's jelly mesenchymal stem cells maintain the expression of key immunomodulatory molecules when subjected to osteogenic, adipogenic and chondrogenic differentiation in vitro: new perspectives for cellular therapy. CSCR. 2013;8:100–113.
- Neve A, Corrado A, Cantatore FP. Osteoblast physiology in normal and pathological conditions. Cell Tissue Res. 2011;343:289–302.
- Chen G, Deng C, Li YP. TGF-β and BMP signaling in osteoblast differentiation and bone formation. Int J Biol Sci. 2012;8:272–288.
- Case N, Rubin J. Beta-catenin—a supporting role in the skeleton. J Cell Biochem. 2010;110:545–553.
- Hu W, Ye Y, Wang J, et al. Bone morphogenetic proteins induce rabbit bone marrow-derived mesenchyme stem cells to differentiate into osteoblasts via BMP signals pathway. Artif Cells Nanomed Biotechnol. 2013;41:249–254.
- Saidak Z, Le Henaff C, Azzi S, et al. Wnt/beta-catenin signaling mediates osteoblast differentiation triggered by peptide-induced alpha5beta1 integrin priming in mesenchymal skeletal cells. J Biol Chem. 2015;290:6903–6912.
- Majumdar MK, Keane-Moore M, Buyaner D, et al. Characterization and functionality of cell surface molecules on human mesenchymal stem cells. J Biomed Sci. 2003;10:228–241.
- Brooke G, Tong H, Levesque JP, et al. Molecular trafficking mechanisms of multipotent mesenchymal stem cells derived from human bone marrow and placenta. Stem Cells Dev. 2008;17:929–940.
- Gronthos S, Simmons PJ, Graves SE, et al. Integrin-mediated interactions between human bone marrow stromal precursor cells and the extracellular matrix. Bone. 2001;28:174–181.
- Foster LJ, Zeemann PA, Li C, et al. Differential expression profiling of membrane proteins by quantitative proteomics in a human mesenchymal stem cell line undergoing osteoblast differentiation. Stem Cells. 2005;23:1367–1377.
- Walsh S, Jordan GR, Jefferiss C, et al. High concentrations of dexamethasone suppress the proliferation but not the differentiation or further maturation of human osteoblast precursors in vitro: relevance to glucocorticoid-induced osteoporosis. Rheumatology (Oxford). 2001;40:74–83.
- Martino MM, Mochizuki M, Rothenfluh DA, et al. Controlling integrin specificity and stem cell differentiation in 2D and 3D environments through regulation of fibronectin domain stability. Biomaterials. 2009;30:1089–1097.
- Walling BL, Kim M. LFA-1 in T cell migration and differentiation. Front Immunol. 2018;9:952.
- Becerra-Bayona SM, Guiza-Arguello VR, Russell B, et al. Influence of collagen-based integrin alpha1 and alpha2 mediated signaling on human mesenchymal stem cell osteogenesis in three dimensional contexts. J Biomed Mater Res A. 2018.
- Wang H, Li J, Zhang X, et al. Priming integrin alpha 5 promotes the osteogenic differentiation of human periodontal ligament stem cells due to cytoskeleton and cell cycle changes. J Proteomics. 2018;179:122–130.
- Swinehart IT, Badylak SF. Extracellular matrix bioscaffolds in tissue remodeling and morphogenesis. Dev Dyn. 2016;245:351–360.
- Wolf K, Friedl P. Extracellular matrix determinants of proteolytic and non-proteolytic cell migration. Trends Cell Biol. 2011;21:736–744.
- Taylor-Weiner H, Schwarzbauer JE, Engler AJ. Defined extracellular matrix components are necessary for definitive endoderm induction. Stem Cells. 2013;31:2084–2094.
- Yang C, Hillas PJ, Báez JA, et al. The application of recombinant human collagen in tissue engineering. BioDrugs. 2004;18:103–119.
- De Bruyn C, Najar M, Raicevic G, et al. A rapid, simple, and reproducible method for the isolation of mesenchymal stromal cells from Wharton's jelly without enzymatic treatment. Stem Cells Dev. 2011;20:547–557.
- La Rocca G, Anzalone R, Corrao S, et al. Isolation and characterization of Oct-4+/HLA-G + mesenchymal stem cells from human umbilical cord matrix: differentiation potential and detection of new markers. Histochem Cell Biol. 2009;131:267–282.
- Wu LF, Wang NN, Liu YS, et al. Differentiation of Wharton's jelly primitive stromal cells into insulin-producing cells in comparison with bone marrow mesenchymal stem cells. Tissue Eng A. 2009;15:2865–2873.
- Petsa A, Gargani S, Felesakis A, et al. Effectiveness of protocol for the isolation of Wharton's Jelly stem cells in large-scale applications. In Vitro Cell Dev Biol Anim. 2009;45:573–576.
- Meinel L, Karageorgiou V, Fajardo R, et al. Bone tissue engineering using human mesenchymal stem cells: effects of scaffold material and medium flow. Ann Biomed Eng. 2004;32:112–122.
- Wang TW, Wu HC, Wang HY, et al. Regulation of adult human mesenchymal stem cells into osteogenic and chondrogenic lineages by different bioreactor systems. J Biomed Mater Res A. 2009;88:935–946.
- Sikavitsas VI, Bancroft GN, Mikos AG. Formation of three-dimensional cell/polymer constructs for bone tissue engineering in a spinner flask and a rotating wall vessel bioreactor. J Biomed Mater Res. 2002;62:136–148.
- Komori T. Regulation of bone development and maintenance by Runx2. Front Biosci. 2008;13:898–903.
- Tang Y, Wu X, Lei W, et al. TGF-beta1-induced migration of bone mesenchymal stem cells couples bone resorption with formation. Nat Med. 2009;15:757–765.
- Yan X, Liao H, Cheng M, et al. Smad7 protein interacts with receptor-regulated Smads (R-Smads) to inhibit transforming growth factor-β (TGF-β)/Smad signaling. J Biol Chem. 2016;291:382–392.
- Javed A, Afzal F, Bae JS, et al. Specific residues of RUNX2 are obligatory for formation of BMP2-induced RUNX2-SMAD complex to promote osteoblast differentiation. Cells Tissues Org. 2009;189:133–137.
- Phimphilai M, Zhao Z, Boules H, et al. BMP signaling is required for RUNX2-dependent induction of the osteoblast phenotype. J Bone Miner Res. 2006;21:637–646.
- Tachi K, Takami M, Sato H, et al. Enhancement of bone morphogenetic protein-2-induced ectopic bone formation by transforming growth factor-β1. Tissue Eng A. 2011;17:597–606.
- Mizuno M, Kuboki Y. Osteoblast-related gene expression of bone marrow cells during the osteoblastic differentiation induced by type I collagen. J Biochem. 2001;129:133–138.
- Li N, Zhang M, Drummen GP, et al. Sika deer antler collagen type I-accelerated osteogenesis in bone marrow mesenchymal stem cells via the Smad pathway. Evid Based Complement Alternat Med. 2016;2016:1.
- Byrne EM, Farrell E, McMahon LA, et al. Gene expression by marrow stromal cells in a porous collagen-glycosaminoglycan scaffold is affected by pore size and mechanical stimulation. J Mater Sci: Mater Med. 2008;19:3455–3463.
- Heckmann L, Fiedler J, Mattes T, et al. Mesenchymal progenitor cells communicate via alpha and beta integrins with a three-dimensional collagen type I matrix. Cells Tissues Org. 2006;182:143–154.
- Jakubikova J, Cholujova D, Hideshima T, et al. A novel 3D mesenchymal stem cell model of the multiple myeloma bone marrow niche: biologic and clinical applications. Oncotarget. 2016;7:77326–77341.
- Salasznyk RM, Williams WA, Boskey A, et al. Adhesion to vitronectin and collagen I promotes osteogenic differentiation of human mesenchymal stem cells. J Biomed Biotechnol. 2004;2004:24–34.