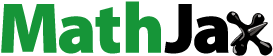
Abstract
Pristine- and strontium-doped Ag2O nanoparticles (NPs) were synthesized utilizing the symbolic co-precipitation method, in which sodium hydroxide was used as a precipitating agent. Various instrumentation methods were employed to get an inside view of the synthesized NPs. Powdered X-ray diffraction (PXRD) analysis revealed the existence of high crystallinity and small-sized NPs (an average diameter range of 35–48 nm). Scanning electron microscopy (SEM) and transmission electron microscopy (TEM) displayed spherical and circular disc-shaped morphology of the particles. Energy-dispersive X-ray spectroscopy (EDX) showed the purity of NPs. All the screened metal functionalized Ag2O NPs exhibited excellent cytotoxicity and antibacterial activities, moderate to good antioxidant and antifungal activities in comparison to Ag2O NPs. Furthermore, nanomaterials were evaluated for DNA interaction studies. The results illustrated that by increasing the concentration of dopant, i.e. strontium up to 5%, the binding affinity of the NPs effectively increased, hence causing the structural changes in DNA.
Introduction
Ever since the appearance of nanotechnology as a prevailing means of applied and basic sciences, it has captivated considerable concentration. Nanoparticles (NPs), due to their smaller dimensions and a greater surface area, have enlarged biological reactivity [Citation1]. With the advancement in the field of nanotechnology, nanomaterials with the exceptional properties have been fabricated. The preceding decade embraces the utilization of inorganic metallic NPs in biomedical applications attributable to their tremendous small size and distinctive physiochemical properties [Citation2]. The binding and cooperative ability of NPs with the biological materials alters the façade characteristics depending upon the present surroundings, hence escalating the apprehension on their probable outcomes on the human beings. Therefore, due to this biological binding, categorization of NPs as hazardous materials becomes complicated [Citation1]. Recently, the interaction of NPs with DNA acting as a hereditary material is gaining a lot of attention due to its significance in interpreting its recognition and in several other uses within gene therapy and drug transporting systems [Citation3]. Silver NPs are essentially used in medicinal and surgical fields owing to their recognized antimicrobial characteristics. In addition, these particles are used for the anticipation of biofilm development and the reduction of bacterial linkage to teeth surface implantation. Ag NPs and its ions acquire an inherent cytotoxic activity [Citation4]. Silver oxide NPs are efficient in opposition to drug-resisting bacteria and leishmania parasite. The bactericidal efficacy of silver oxide NPs, broadly used in antimicrobial applications, is ascribed to the electrical changes occurring as a result of interaction between NPs and bacterial membrane. These changes play a vital role in the increased and improved reactivity of surfaces of silver NPs [Citation5]. Panacek et al. [Citation6] stated the elevated antimicrobial and bactericidal action of silver oxide NPs on two different kinds of bacteria, i.e. Gram positive and Gram negative. Results depict that the bactericidal efficiency is NP size dependent [Citation6]. Shahverdi et al. [Citation7] examined the coalescing outcomes of silver oxide NPs and antibiotics on bacterial species like Staphylococcus aureus and E. coli. The incidence of silver oxide NPs enhanced the bactericidal efficiency of the antibiotics like Penicillin G, Vancomycin, etc. against the bacteria [Citation7]. Lara et al. [Citation8] inspected the effects of antibacterial activity of silver oxide NPs on the multi-drug defiance bacteria and concluded that these particles were incredibly effective against various antibiotic-resisting bacteria. The bacterial species Streptococcus pyogene that offers resistance against erythromycin were fully hindered, while the number of other drug-resisting bacterial species showed a steady decline at an increased concentration in divergent control groups [Citation8]. In the precedent years, researchers have explored several new applications, demonstrating the importance and handy nature of silver oxide, but there are no reports of surface modification of these NPs. Deeming this fact, the present work aspires to modify the surface of silver oxide by doping it with renowned bivalent cation strontium as it has been verified to be highly efficient. In the previous researches, various semiconductors in the dearth of noble metal co-catalysts have proven to be photo-catalytically active. Iwase et al. [Citation9] has investigated that by modifying the surface of sodium tantalum oxide with Sr, the rate of photo-catalytic activity of water splitting was greatly enhanced due to smaller dimensions of the Sr2+ ion. In the meantime, Yuexiang Li et al. [Citation10] has also stated that by doping the TiO2 surface with the alkaline earth metals, the hydrogen production photo-catalytically was significantly enlarged depending upon the size and the fabrication method. Salim et al. [Citation11] reported that with variation in the conditions of Sr-doped NiO synthesized ceramic, i.e. escalating the sintering temperature, the enlargement of the granular size and hence the density with the loss of porosity is observed. The enhanced values of dielectric constant are correlated with the lower dielectric loss [Citation11]. The group of K.P. Bansal indexed that the growing amount of strontium in chromite-based ceramic leads to the augmentation of granular size and the conductivity, but the addition of the dopant beyond the solubility reduces the bulk conductivity by increasing the thickness of the grain boundaries. The magnetic susceptibilities of the doped ceramic materials were found to be less than those of the undoped one in the considered temperature range. Furthermore, by increasing the amount of the dopant strontium, the magnetic susceptibility became impassive by the change in temperature [Citation12]. Therefore, keeping in accord of all the above evidences, it is concluded that the dopant strontium increases the activity, grain size, total conductivity and the dielectric constant. The aim of the present research is (1) to synthesize silver oxide NPs functionalized with varying concentrations of strontium; (2) characterization and comparative study of pristine and metal-functionalized NPs; (3) to investigate the effect of surface modification of silver oxide NPs with different amounts of strontium dopant on the size, shape and other properties; (4) to analyze the antimicrobial and therapeutic potentials of the synthesized NPs and (5) to study the role of NPs in biomedical applications and in DNA rupturing.
Experimental
Materials and methods
UV–Vis absorption spectra were recorded on Shimadzu 1800 spectrophotometer ranging from 200 to 800 nm. The silver oxide and strontium-doped NPs were characterized with CuKα radiation of 0.154 nm in the range of different diffraction angles of 20–80. The prepared samples were sonicated for 10 min after dispersing them in n-Hexane. Surface morphology studies were examined by utilizing JSM-6500F and Hitachi S-3000H scanning electron microscope (SEM). Furthermore, the energy-dispersive X-ray spectroscopy (EDX) spectra of all the catalysts were also obtained during their conventional SEM investigations. Additional structural information of as-synthesized NPs was inspected using JEM-1400 operating at 100 kV for transmission electron microscopy (TEM) imaging.
Synthesis of strontium-doped silver oxide NPs
Strontium-doped silver oxide NPs were synthesized using symbolic co-precipitation method in which sodium hydroxide was used as a precipitating agent. In the first step, aqueous solution of silver nitrate (0.01 M) was prepared under continuous stirring at 80 °C, followed by addition of SrCO3. Varying concentrations of SrCO3 that is 1 × 10−5 M (0.00147 g), 3 × 10−5 M (0.0044 g), 5 × 10−5 M (0.0073 g), 7 × 10−5 M (0.01 g) and 9 × 10−5 M (0.013 g) corresponding to 1, 3, 5, 7 and 9%, respectively were added in the reaction mixture. In the second step, aqueous solution of 1 M sodium hydroxide was added dropwise that turned the solution of reaction mixture from colorless to grayish-yellow colloidal suspension, indicating the formation of thermodynamically unstable silver hydroxide which then undergoes recombination process to yield Ag2O (pk = 2.8) through the following mechanism [Citation13]:
(1)
(1)
(2)
(2)
The solution mixture was then left as such for 2 h, maintaining the reaction conditions for completion of reaction. After filtration, the filtrate was decanted off, and brown-colored 1, 3, 5, 7 and 9% strontium-doped silver oxide NPs were obtained on the filter paper; afterwards, these particles were washed several times with distilled water and dried in the oven at 60 °C.
Brine shrimp lethality assay
Experiments were carried out in a 96-well plate against the brine shrimp larval specie (Artemia salina) following the already described protocol in the literature with minor modifications. The eggs of A. salina were incubated for a time period of 24–48 h in simulated sterilized se 6ba water (38 g/L supplemented along with 6 mg/L of dried yeast) in a particularly designed two compartment plastic trays, under irradiating light and hot environment (30–32 °C). Then, 10 fully grown phototropic nauplii with the aid of Pasteur pipette were harvested and shifted to each and every well of plate. Volumes of doped and pristine NPs consistent with each other restraining ≤1% DMSO in sea water at final concentrations of 500 and 200 µg/mL were then shifted to the wells containing both sea water and shrimp larvae. In every well, the absolute volume of 300 µL was reserved. Serial concentrations of doxorubicin and 1% DMSO were included in the positive- and negative-controlling wells. After the passage of 24 h time period, the number of shrimps alive was counted, giving an idea about the percentage of mortality, and finally by the utilization of table curve of 2 D v5.01 software median lethal concentration (LC50) was evaluated [Citation14].
Determination of total antioxidant capacity (TAC)
The determination of TAC of NPs was done by phospho-molybdenum method with little modification. To the 900 µL reagent solution consisting of 28 mM sodium phosphate, 4 mM ammonium molybdate and 0.6 M sulfuric acid, 100 µL from the stock solutions of each NP was added. By utilizing a micro-plate reader, the absorbance was determined at a wavelength of 695 nm after cooling the reaction mixtures at room temperature incubated for 90 min at an elevated temperature of 95 °C. Ascorbic acid as a positive control was used for the calibration curve, and the resulting TAC was expressed as ascorbic acid equivalent per mg dry weight (µg AAE/mg DW) [Citation15].
Reducing power assay
The investigation of reduction potential was carried out in accordance with the methods reported in literature. Precisely, 100 µL of different samples (4 mg/mL extract in DMSO) was mixed with 200 µL of phosphate buffer (0.2 M, pH 6.6) and 250 µL of 1% w/v potassium ferricyanide. The reaction mixtures were then made acidic with the addition of the 200 µL of 10% w/v trichloroacetic acid after the incubation at 50 °C for a time period of just 20 min. The centrifugation of the solution mixtures was done at 3000 rpm for 10 min. Then, the mixture of 0.1% w/v ferric chloride (50 µL) and upper floating liquid (150 µL) was prepared, and lastly absorbance was measured at a wavelength of 630 nm. Ascorbic acid was preserved as positive control, and results were articulated as µg AAE/mg DW [Citation16].
DPPH free radical-scavenging assay
The free radical-scavenging potential was examined using 2, 2-diphenyl-1-picrylhydrazyl reagent. For NPs, 10 µL solution (4 mg/mL) in 190 µL of DPPH (0.004% w/v in methanol) was prepared. Afterwards, the resultant mixture was kept under dark for 1 h of incubation; absorbance was measured at 515 nm. Positive and negative controls comprised ascorbic acid and DMSO, respectively. For the evaluation of IC50 values, firstly, the screening of the samples was done at the final concentration of 200 µg/mL. Finally, the ones showing good DPPH quenching ability were checked at lower concentrations (200, 66.6, 22.2, 7.41 µg/mL). The subsequent formula was used for the calculation of the percent inhibition assay [Citation16]
(3)
(3)
where Abs is the observed absorbance of DPPH solution along with the sample, whereas Abo represents the absorbance of negative control (containing the reagent and solvent only). The IC50 was calculated by using table curve software 2D Ver. 4.
Antibacterial activity
The bactericidal efficiency of the NPs was examined following the protocols mentioned previously against three Gram-negative strains (Bordetella bronchiseptica ATCC # 4617, Salmonella typhimurium ATCC # 14028 and Enterobacter aerogenes ATCC # 13048). In the nutrient broth, these negative strains were cultured followed by the incubation at 37 °C for 24 h. The turbidity was adjusted to 104 CFU/mL by using deionized water and then comparing it with the standard turbidity of McFarland 0.5 BaSO4. Petri plates having nutrient agar (20 mL) were then washed down by the 100 µL of refreshed inocculum. Afterwards, sterile filter paper discs (6 mm) infused with the test samples (5 µL of 20 mg/mL DMSO; 100 µg/disc) were positioned on the seeded nutrient agar plate. The media supplemented with Cefixime-USP (20 µg/disc) and DMSO-impregnated discs were used as the positive and negative controls, respectively. Clear inhibition growth zones were deliberated after incubation of 24 h [Citation16].
Antifungal activity
For the assessment of the antifungal activity of the NPs, reported literature method was followed in which three fungal strains (Aspergillus fumigatus FCBP # 66, Fusarium solani FCBP # 0291, N. niger FCBP # 0300; purchased from Fungal Culture Bank of Pakistan, Lahore, Pakistan) were cultured and kept on the Sabouraud dextrose agar (SDA). The spores were gathered in 0.02% Tween 20 solution where the turbidity adjustment was done in accordance to the McFarland 0.5 turbidity standard, before the sensitivity examination. On the plates possessing SDA (25 mL), each harvested spore (100 µL) was swabbed, followed by the placement of discs of filter paper loaded with 5 µL of test sample (20 mg/mL DMSO; 100 µg/disc), besides the standard antifungal terbinafine (50 µg/disc) and DMSO on seeded SDA plates. At the end, a clear inhibition zone growth around the discs was measured with the help of Vernier caliper after the incubation of the plates at 28 °C for 24–48 h [Citation16].
DNA interaction studies spectrophotometrically
The salmon DNA solution was prepared by dissolving commercially available suitable amount of salmon sperm DNA in the Tris (hydroxyl methyl) amino methane-HCl buffer (25 mM) solution (pH = 7.5) under continuous agitation for 12 h at 4 °C. The interaction of DNA with silver oxide NPs was monitored using UV–Vis spectra obtained from the Shimadzu Spectrophotometer UV-1800 (Kyoto, Japan). Changes in the absorption spectra were recorded by maintaining the concentration of DNA (200 µM) constant with the addition of varying concentrations of NPs in the UV–Vis absorption range of 200–800 nm. All the tests were conducted in the quartz cells of 1 cm path length thermostated for sustaining the temperature at 310 K [Citation1].
Result and discussion
Characterization of NPs
The opted co-precipitation method resulted in the production of silver oxide and various concentrations of strontium-doped silver oxide NPs varying in size, shape and properties. Different physical techniques such as SEM, TEM, powder X-ray diffraction (PXRD) and EDX were used for the characterization of the fabricated NPs. The XRD pattern obtained was compared with reported data from JCPDS card no. 00–001-1041 and indexed as a pure cubic crystal system of Ag2O with Pm–3m space group and a primordial cubic unit cell with a, b, c = 4.72 Å. The incidence of various strong diffraction peaks at (111), (200), (220) and (311) planes specifies the favored growth of atoms alongside these planes of primordial cubic system. The absence of any other reflection in the XRD pattern other than silver oxide divulges the sample’s high-phase purity. Nano-dimensions of the particles are allied to the slightly broad reflections in the pattern. XRD analysis confirms the crystallinity of synthesized NPs (). The average diameter of Sr-doped Ag2O NPs was calculated using Scherrer’s equation. D = k λ/β Cos θ where D represents the average crystallite size perpendicular to the reflecting planes, K is Debye Scherrer’s coefficient (0.94), λ is wavelength of X-rays (1.5406 A°), β represents FWHM, i.e, full width at half maximum in radians, and θ is the diffraction angle. The average crystallite sizes of different synthesized NPs with the varying dopant concentrations are listed in .
Table 1. Average crystallite sizes of catalysts.
Ag2O NPs exist in different morphologies such as cubic, octapods and hexapods depending upon the reaction conditions used for their fabrication. Scanning electron micrograph technique was utilized to get the details about the surface topography of the catalyst. Results obtained revealed that strontium-doped NPs were structurally spherical and cubical, evenly distributed on the surface, indicating that the morphology is uniform and well defined in comparison to undoped Ag2O (). The sample’s purity was further confirmed through EDX which gives information about the elemental composition of prepared NPs, analysis proving the absence of any other elemental peak as an impurity except the expected one, containing a higher concentration of silver, oxygen and strontium. Silver Ag Lα appears at 2.984 KeV and Sr Lα appears at 1.806 KeV, while O Kα appears at 0.525 KeV (). TEM images of NPs divulge the existence of a broad diversity in their size and shape. Ag2O NPs have a relatively large diameter in comparison to Sr-doped Ag2O NPs, though spherical and circular disc-shaped NPs are found abundantly. However, a gradual decrease in the size of doped NPs is apparent with an increase in the weight ratio of strontium ().
Figure 2. SEM images of (a) Ag2O, (b) 1% Sr/Ag2O, (c) 3% Sr/Ag2O, (d) 5% Sr/Ag2O, (e) 7% Sr/Ag2O and (f) 9% Sr/Ag2O.
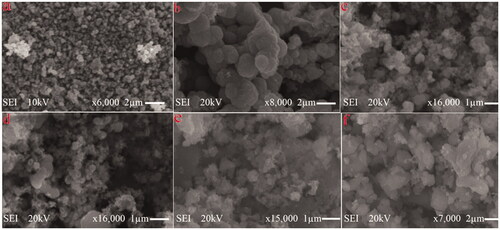
Brine shrimp lethality assay
The toxicity of various materials is examined by carrying out brine shrimp lethality studies that gives a proficient preface approach for the determination of several activities including antimicrobial, antitumor, etc. Many compounds that are biologically active show enhanced lethality against the A. salina shrimps at higher concentrations, hence making it a simple and suitable mediator for initial testing of diverse pharmacological activities [Citation17]. In this study, all the synthesized NPs had LC50 values less than 1000 µg/ml, suggesting that this cytotoxicity of the catalysts is accountable for the toxicology. In general, higher the mortality rate, lesser will be the lethal concentration value and then higher will be the cytotoxicity of the catalyst. The cytotoxicity of NPs is connected with anticancer activity as they can be an alternate resource of anticancer drug [Citation18]. Amongst all the prepared NPs, 3% Sr/Ag2O proved to be the most cytotoxic in nature with the highest percentage of mortality up to 93.6% against standard doxorubicin ().
DPPH free radical-scavenging assay
The assessment of the decolorization of DPPH solution helps in the evaluation of the free radical-extracting activity that includes the conversion of the purple tint of DPPH into a stabilized yellow-colored organic molecule particularly diphenyl picryl hydrazine upon reception of an electron or a hydrogen radical released from the donating agent that is antioxidant [Citation14]. Substances that are proficient to undergo such a type of reaction are regarded as antioxidants and hence radical-scavenging compounds. The highest free radical-scavenging potential is observed in the case of 5 and 9% Sr- doped Ag2O NPs as they displayed the utmost percent inhibition in comparison to ascorbic acid standard ().
Total antioxidant Capacity
The TAC of the catalysts is examined against standard AAE. In this technique, molybdenum is reduced from +6 to Mo (+5) with the help of antioxidant agents, leading to the development of a green-colored complex of phosphate/Mo (V). The obligatory natural process in biological systems is oxidation that includes the production of the hydroxyl and peroxyl radicals that have a pronounced high reactivity and are quenched by the antioxidants [Citation14]. The antioxidant capacity is found maximum in the case of 9% Sr/Ag2O which is in agreement with the DPPH results, hence establishing a positive correlation ().
Total reducing power
In general, the reducing properties are attributed to the existence of the reductones that are connected to the action of antioxidants by donating the hydrogen atom, breaking the chain of free radicals, therefore developing a direct correlation between the antioxidant capacity and the total reducing power [Citation14]. In this study, all the samples illustrated a good reducing property, the highest shown by 7% Sr/Ag2O followed by 9% Sr/Ag2O, having a slight difference between them and the least shown by 3% Sr/Ag2O ().
Antimicrobial assays
Antibacterial activity
The evaluation of the antibacterial potential of the NPs was done against three bacterial stains such as E. aerogens, B. bronchiseptaca and S. typhimurim. All the doped and undoped catalysts showed significant activity against these strains. Amongst all the bacterial strains tested, the highest susceptibility with maximum inhibition was found in the case of B. bronchiseptaca (). Amongst all the catalysts, 9% Sr-doped Ag2O showed maximum inhibition growth zone of 20 mm followed by 5% Sr/Ag2O exhibiting the growth zone of 18 mm. Considering the case of S. typhimurim highest inhibition zone of 19 mm shown by 3% Sr/Ag2O followed by 5% and 9% () Strontium doped silver oxide NPs with 15 mm and 12 mm of inhibitory growth, respectively. The enhanced bactericidal activity of the doped NPs is attributed to the strontium content present on the surface. Ag2O penetrating the bacteria interacts powerfully with the molecules restraining sulphur and phosphorus groups such as DNA. Upon exposure to silver oxide NPs, DNA loses its replication capability due to the induction of apoptosis. The inactivity of bacterial enzymes occurs as silver oxide enters the bacteria where it forms atomic silver Ag° and the clusters of Ag+ and releases hydrogen peroxide and other free radicals which cause the cell death [Citation19]. It is expected that with the doping of strontium, this process is facilitated, hence causing more cell death. The other reason behind the increased efficiency of doped silver oxide NPs may be due to the defects in Ag2O lattice caused by the strontium doping, upsetting the O/Ag stoichiometric ratio along with the increase in the electrostatic forces and binding strength of NPs with bacteria, hence generating stronger adsorption [Citation20].
Table 2. Efficiencies against various bacterial strains.
Antifungal activity
The antifungal potential of the catalysts was evaluated using three filamentous strains of fungi. Different antifungal inhibitory zones of growth are enlisted in . The data illustrate that 3% Sr/Ag2O shows significant growth against A. fumigatus and 5% strontium-doped silver oxide against N. niger that is believed to be ubiquitous fungi that affects corn by producing potential mycotoxins on the eatables (). The key factor determining the sample’s antifungal activity is lipid solubility. The permeability of the cell is controlled by the lipid membrane that permits lipid-soluble substances in the cell. The polarity of the metal ion is decreased by chelation, owing to the ligand orbital overlap and partial sharing of metal ion’s positive charge with donor groups. An increase in lipophilicity causes easy penetration of the complexes in the lipid membrane, hence blocking the binding sites of metals on the enzymes of the microorganism [Citation21].
Table 3. Efficiencies against various strains of fungi.
DNA interaction studies
The interaction of NPs with DNA was studied through UV–Vis spectroscopy in order to comprehend the binding mode of DNA. By keeping the concentration of DNA constant, different concentrations of NPs were added successively, maintaining the temperature at 37°C. Upon addition of silver oxide NPs up to approximately 25 µM, there was no significant increase in the absorption peak of DNA; however, a slight blue shift in the peak was observed, depicting that Ag2O NPs have a weak-binding affinity with DNA (). The value of “K” binding constant was calculated by plotting the double reciprocal linear plots according to the subsequent Equation (1)
(4)
(4)
where Ao represents the absorbance peak of DNA at 264 nm without the addition of NPs and
is the absorbance documented at 264 nm for DNA with the successive addition of NPs. The ratio of intercept to the slope gave the binding constant from the linear plot between 1/(A−Ao) and 1/[NPs].
Figure 9. DNA–Ag2O NPs interaction: (a) plot of absorbance versus wavelength (nm); (b) comparative change in absorbance of DNA with addition of NPs; and (c) linear plot for estimation of binding constant.
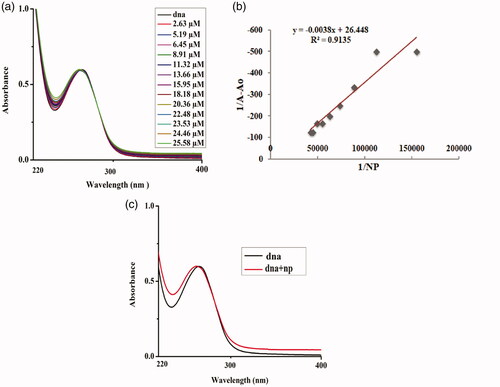
The addition of varying concentrations of 1% Sr-doped Ag2O NPs up to 50 µM depicted a hyperchromic effect which is the absorbance of the DNA at 267 nm increased along with the blue shift of 5 nm in the peak, indicating the binding mode to be electrostatic in nature (). When 5% Sr/Ag2O was added successively keeping the concentration of DNA constant at 265 nm, blue shift of about 7 nm was recorded along with an increase in the absorption peak, illustrating the strong binding interaction of NPs via electrostatic interactions to the DNA (). The binding of DNA with metallic nanoparticles is of major significance for the researchers of integrative discipline of nanoscience due to its potential persuasion on the fabrication, imitation and structural cohesion of DNA and RNA [Citation1]. The change in the structural confirmation of the DNA is governed by the charge present on the NPs. The positively charged NPs bind with the anionic phosphate backbone of the DNA molecule via a permutation of electrostatic interaction, groove binding and third being the intercalative mode. NPs are highly positively charged, and binding to the DNA causes it to enfold and twist while such a trend is not observed when the surface of NPs is weakly charged [Citation22].
Figure 10. DNA–1%Sr/Ag2O NPs interaction. (a) Plot of absorbance versus wavelength (nm). (b) Linear plot for estimation of binding constant.
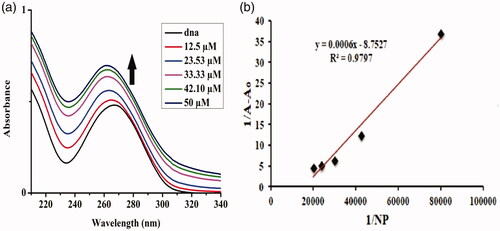
Figure 11. DNA–5%Sr/Ag2O NPs interaction. (a) Plot of absorbance versus wavelength (nm). (b) Comparative change in absorbance of DNA with addition of NPs. (c) Linear plot for estimation of binding constant.
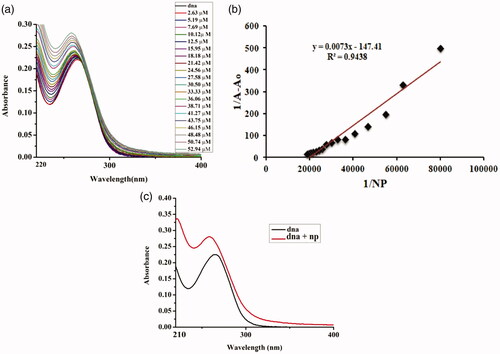
DNA binding parameters
The binding constant along with Gibb’s free energy is enlisted in . The negative value of ΔG shows that the reaction is spontaneous in nature. And through the binding constant values, it can be inferred that by increasing the concentration of the strontium dopant in the NPs, the binding affinity of NPs increases successively ().
Table 4. DNA binding parameters.
Conclusion
In summary, this study reveals that Ag2O NPs with different strontium-dopant concentrations have been successfully synthesized. After inclusive characterization of pristine- and strontium-doped Ag2O NPs, we have depicted that NPs showed improved and enhanced activities in the presence of a strontium dopant. Therapeutic potential studies revealed that all the screened catalysts are biologically active, showing significant antifungal and antibacterial results in the dark with excellent cytotoxicity, hence demonstrating the potential medicinal importance of NPs. Furthermore, DNA binding studies carried out spectrophotometrically illustrate that by increasing the concentration of strontium up to 5% in the nanomaterials, DNA binding affinity increases efficiently, hence totally breaking its confirmation.
Disclosure statement
No potential conflict of interest was reported by the authors.
Additional information
Funding
References
- Roy S, Sadhukhan R, Ghosh U, et al. Interaction studies between biosynthesized silver nanoparticle with calf thymus DNA and cytotoxicity of silver nanoparticles. Spectrochim Acta A Mol Biomol Spectrosc. 2015;141:176–184.
- Sasidharan A, Riviere JE, Monteiro-Riviere NA. Gold and silver nanoparticle interactions with human proteins: impact and implications in biocorona formation. J Mater Chem B. 2015;3:2075–2082.
- Wahab R, Kim Y-S, Hwang I, et al. A non-aqueous synthesis, characterization of zinc oxide nanoparticles and their interaction with DNA. Synth Met. 2009;159:2443–2452.
- Rutberg F, Dubina M, Kolikov V, et al. Effect of silver oxide nanoparticles on tumor growth in vivo. Dokl Biochem Biophys. 2008;421:191–193.
- Raffi M, Hussain F, Bhatti T, et al. Antibacterial characterization of silver nanoparticles against E. Coli ATCC-15224. J Mater Sci Technol. 2008; 24:192–196.
- Panacek A, Kvítek L, Prucek R, et al. Silver colloid nanoparticles: synthesis, characterization, and their antibacterial activity. J Phys Chem B. 2006;110:16248–16253.
- Shahverdi AR, Fakhimi A, Shahverdi HR, et al. Synthesis and effect of silver nanoparticles on the antibacterial activity of different antibiotics against Staphylococcus aureus and Escherichia coli. Nanomed Nanotechnol Biol Med. 2007;3:168–171.
- Lara HH, Ayala-Núñez NV, Turrent L. d C I, et al. Mode of antiviral action of silver nanoparticles against HIV-1. J Nanobiotechnol. 2010;8:1–621.
- Iwase A, Kato H, Okutomi H, et al. Formation of surface nano-step structures and improvement of photocatalytic activities of NaTaO 3 by doping of alkaline earth metal ions. Chem Lett. 2004;33:1260–1261.
- Li Y, Peng S, Jiang F, et al. Effect of doping TiO2 with alkaline-earth metal ions on its photocatalytic activity. J Serb Chem Soc. 2007;72:393–402.
- Mohd Salim NN, Mohamed JJ, Ahmad ZA. Effect of sintering temperatures on Sr-doped NiO ceramics. Mater Sci Forum. 2016;840:61–65. [Zurich, Switzerland: Trans Tech Publications Inc.]
- Bansal K, Kumari S, Das B, et al. On some transport properties of strontium-doped lanthanum chromite ceramics. J Mater Sci. 1983;18:2095–2100.
- Sullivan KT, Wu C, Piekiel NW, et al. Synthesis and reactivity of nano-Ag2O as an oxidizer for energetic systems yielding antimicrobial products. Combust Flame. 2013;160:438–446.
- Fatima H, Khan K, Zia M, et al. Extraction optimization of medicinally important metabolites from Datura innoxia Mill.: an in vitro biological and phytochemical investigation. BMC Complement Altern Med. 2015;15:376.
- Ali A, Ambreen S, Javed R, et al. ZnO nanostructure fabrication in different solvents transforms physio-chemical, biological and photodegradable properties. Mater Sci Eng C Mater Biol Appl. 2017;74:137–145.
- Tabassum S, Ahmed M, Mirza B, et al. Appraisal of phytochemical and in vitro biological attributes of an unexplored folklore: Rhus Punjabensis Stewart. BMC Complement Altern Med. 2017;17:146.
- Ali A, Ambreen S, Maqbool Q, et al. Zinc impregnated cellulose nanocomposites: synthesis, characterization and applications. J Phys Chem Solids. 2016;98:174–182.
- Ghareeb MA, Hussein A, Hassan M, et al. Antioxidant and cytotoxic activities of Tectona grandis linn. leaves. Int J Phytopharm. 2014; 5:143–157.
- Allahverdiyev AM, Abamor ES, Bagirova M, et al. Antimicrobial effects of TiO(2) and Ag(2)O nanoparticles against drug-resistant bacteria and leishmania parasites. Future Microbiol. 2011;6:933–940.
- Guo B-L, Han P, Guo L-C, et al. The antibacterial activity of Ta-doped ZnO nanoparticles. Nanoscale Res Lett. 2015;10(1):1047.
- Sadeek SA, El-Shwiniy WH, Zordok WA, et al. Synthesis, spectroscopic, thermal and biological activity investigation of new Y (ΙΙΙ) and Pd (ΙΙ) norfloxacin complexes. J Argent Chem Soc. 2009; 97:128–148.
- Railsback JG, Singh A, Pearce RC, et al. Weakly charged cationic nanoparticles induce DNA bending and strand separation. Adv Mater Weinheim. 2012;24:4261–4265.