Abstract
Type 1 diabetes (T1D) is an autoimmune disease caused by the destruction of insulin-producing cells. Due to the ability of apoptotic cells clearance to induce tolerance, we previously generated liposomes rich in phophatidylserine (PS) –a feature of apoptotic cells– loaded with insulin peptides to mimic apoptotic beta-cells. PS-liposomes arrested autoimmunity in experimental T1D through the induction of tolerance. The aim of this study was to investigate the potential of several peptides from different T1D autoantigens encapsulated in (PS)-liposomes for T1D prevention and to assess its safety. T1D autoantigens (Insulin, C-peptide, GAD65 and IA2) were encapsulated in PS-liposomes. Liposomes were administered to the 'gold-standard' model for the study of autoimmune T1D, the Non-Obese Diabetic mouse, that spontaneously develop the disease. Safety and toxicity of liposomes were also determined. Only PS-liposomes encapsulating insulin peptides decrease T1D incidence in the Non-Obese Diabetic mouse model. Disease prevention correlates with a decrease in the severity of the autoimmune islet destruction driven by leukocytes. PS-liposomes neither showed toxic effect nor secondary complications. Among the here referred autoantigens, insulin peptides are the best candidates to be encapsulated in liposomes, like an artificial apoptotic cell, for the arrest of autoimmunity in T1D in a safe manner.
Keywords:
Introduction
An essential goal to both prevent and cure type 1 diabetes (T1D) is to arrest the autoimmune reaction against β-cells. Insulin is a key autoantigen in T1D and the only β-cell-specific autoantigen, as well as a major target of the autoimmune attack mediated by T lymphocytes [Citation1]. Moreover, there is also a genetic association between polymorphisms of the insulin gene and the risk to develop T1D [Citation2]. However, in addition to insulin, other autoantigens such as the glutamic acid decarboxylase 65 (GAD65), islet-antigen 2 (IA2) and zinc transporter 8 (ZnT8) have been described in T1D. They are known to induce autoantibodies and T cell responses. Autoantibodies against insulin or GAD isoforms are the most common in the early phases of T1D and, occasionally, multiple auto-antibodies arise during advanced stages of the pathology [Citation3]. Although insulin is a primary autoantigen in T1D, other autoantigens are involved in the progression of the disease after epitope spreading [Citation4].
Furthermore, in order to arrest the autoimmune reaction against β-cells, immunological tolerance to T1D autoantigens must be restored. A process known to promote tolerance is efferocytosis, or phagocytosis of apoptotic cells. These dying cells exhibit surface phosphatidylserine (PS) to be distinguished from living cells and to allow for their recognition by phagocytes [Citation5,Citation6]. Following these principles, an antigen-specific cell immunotherapy using apoptotic β-cells was demonstrated efficacious in re-establishing specific immunological tolerance, which was mediated by dendritic cells (DCs) rendered tolerogenic after efferocytosis [Citation7]. Because autologous apoptotic β-cells for T1D treatment cannot be easily obtained, artificial cells (PS-liposomes) were generated to mimic tolerogenic apoptotic cell function [Citation8]. A previous work demonstrated the therapeutic potential of PS-liposomes encapsulating insulin peptides – as a main autoantigen in T1D – to prevent T1D in an experimental model of the disease, the Non-Obese Diabetic (NOD) mouse [Citation8]. The NOD mouse, developed in 1980 [Citation9] is considered the ‘gold-standard’ model for the study of T1D [Citation10]. These mice develop T1D spontaneously around 12 weeks of age, after the immune-mediated destruction of insulin-producing β cells, commonly called insulitis. NOD mice have been extensively utilised to study the ethiopathogenesis of the disease and to test new immunotherapies. This model mouse closely resembles human disease, albeit the obvious differences with human populations.
In order to better mimic the apoptotic β-cell, and to try to increase the efficacy of the nanotherapy, the present study aimed at encapsulating other peptides from several T1D autoantigens in PS-liposomes and to test its therapeutic effect in arresting autoimmunity. In addition, and in order to confirm the safety of the nanotherapy, the effects of a severe administration strategy were assessed in NOD mice.
Material and methods
Mice
Wild-type non-obese diabetic (NOD) mice were purchased from Charles River (Calco, Lecco, Italy) and kept under specific pathogen-free conditions at the Animal House facility of the Germans Trias i Pujol Research Institute. Mice were in a temperature- and humidity-controlled room with 12 h light/12h dark cycle, provided with standard chow diet (Teklad Global 14% Protein Rodent Diet, Envigo, Sant Feliu de Codines, Spain) and water ad libitum. To detect spontaneous development of T1D, NOD mice were monitored daily for urine glucose using Glucocard strips (Menarini, Barcelona, Spain), starting at 10 weeks of age and until 25 weeks of age. Mice with glycosuria were confirmed diabetic when the blood glucose level was >300 mg/dL. Mice were anesthetised with isofluorane inhalation to obtain serum and euthanized by cervical dislocation to harvest the pancreas at 25 weeks of age or at T1D onset. The study was approved by the Committee on the Ethics of Animal Experimentation of the Germans Trias i Pujol Research Institute and carried out in strict accordance with the recommendations in the Guide for the Care and Use of Laboratory Animals of the Generalitat de Catalunya, Catalan Government.
Peptides and liposomes
Peptides were selected because they are target epitopes in T1D [Citation4]. Peptides from Insulin, C-peptide, GAD65 and IA2 () were chosen (GL Biochem, Shanghai, China). Peptides were >95% pure and Trifluoroacetic acid was removed. Liposomes were composed of 1,2-dioleoyl-sn-glycero3-phospho-L-serine (sodium salt) (DOPS, Lipoid, Steinhausen, Switzerland) and 1,2-didodecanoyl-sn-glycero-3-phosphocholine (DLPC, Lipoid), and cholesterol (CH, Sigma Aldrich, MO, USA). Liposomes were prepared using the thin-film hydration method from a lipid mixture, to mimic apoptotic bodies as described, under sterile conditions and at a final concentration of 30 mM [Citation11]. Encapsulation efficiencies (EE) were calculated according to the equation EE (%) = [(peptide, total–peptide, out)/peptide, total] × 100, where peptide, total is the initial peptide concentration and peptide, out is the concentration of non-encapsulated peptide. To measure the peptide, out, liposome suspensions were centrifuged at 110.000 × G at 10 °C for 30 min. The concentration of non-encapsulated peptide was assessed in the supernatants by PIERCE BCA protein assay kit (Thermo Fisher Scientific Inc., IL, USA). For toxicity experiments, empty PS-liposomes were generated. The distribution of the particle size and the stability – given as ζ potential – were measured by dynamic light scattering using Malvern Zetasizer (Malvern Instruments, Malvern, UK) in undiluted samples. The morphology of liposomes was examined by cryogenic transmission electron microscopy with a JEOL-JEM 1400 device (Jeol Ltd., Tokyo, Japan).
Table 1. Sequences of peptides encapsulated in PS-liposomes.
T1D prevention and assessment of islet leukocytic infiltration
Normoglycemic 8-week-old NOD mice were treated with a single dose of liposomes. Briefly, 3.5 mg of the different peptide-filled PS-liposomes in 200 μL saline solution were injected i.p. Thus, PSAB-lipo group received 200 μL of a mix of PSA and PSB liposomes, PSGAD65-lipo group received 200 μL of a mix of PSG1 and PSG2 liposomes, PSIA2-lipo group received 200 μL of PSI1 and PSI2 liposomes and PSCpep-lipo group received 200 μL of PSC liposomes. A group containing 200 μL of a mix of all the different peptide-loaded PS-liposomes (Cock-lipo) was included, in which each liposome fraction was present in an equal amount. A group containing the mix of all the different peptides (200 μL) was also included (Cock-Pep), the amount of peptide given being in accordance to encapsulation efficiency inside the liposomes. Sham group received saline solution. Each group contained 5–9 animals. Mice were monitored daily for glycosuria (Glucocard strips, Menarini, Barcelona, Spain) until 25 weeks of age or detection of T1D. To determine the destructive islet leukocytic infiltration, insulitis score was assessed at 25 weeks in all non-diabetic mice. Pancreases were snap frozen in an isopentane/cold acetone bath. Non-overlapping cryosections (5 μm) were stained with haematoxylin and eosin (H/E). A blind analysis was performed by two independent observers, analysing a minimum of 40 islets per animal. Insulitis was scored as previously described: 0, no insulitis; 1, peri-insular; 2, mild insulitis (<25% of the infiltrated islet); 3, 25–75% of the islet infiltrated; 4, >75% islet infiltration [Citation12].
Evaluation of the toxicity of PS-liposomes
In order to determine the safety of PS-liposomes, a severe administration strategy was tested in NOD mice. Six normoglycemic mice (8–12-week-old, 3 males and 3 females) were daily injected i.p. with 200 µL of 3.5 mg 30 mM-lipid PS-liposomes during 14 consecutive days. Simultaneously, PBS administration was used as control (sham) in 6 mice (3 males and 3 females). Mice health and welfare was monitored (Supplementary Table S1) during 21 days in terms of weight, glucosuria, physical appearance (fur, presence of secretions or bruises) and behaviour (barbering, physical activity, aggressiveness, stereotyped activities and pain). Mice were euthanised at the end of the follow-up or if the total score reached a maximum value of 3. Serum from 4 mice of each group was analysed for biochemical parameters, such as total cholesterol (Total CH), high-density lipoprotein (HDL) CH, triglycerides, creatinine, urea and alanine transferase (ALT).
Statistical analysis
The statistical analysis was performed using the Prism 7.0 software (GraphPad software Inc., CA, USA). Mantel-Cox Log-Rank was used to T1D incidence analysis. Mann–Whitney test was used for comparisons of nonparametric and unpaired data. A p-value ≤ .05 was considered significant.
Results
PS-liposomes encapsulating insulin, but not C-peptide, GAD65 or IA2 peptides decrease T1D incidence
Liposomes were characterised in terms of diameter, polydispersity index (PdI), surface charge (ζ-potential) and efficiency of peptide encapsulation (). All liposomes had a final lipid concentration of 30 mM. NOD mice were treated with a single dose of liposomes before T1D onset (8 weeks old) (). At the end of the follow-up period, T1D incidence tended to be lower in both PSAB-lipo and Cock-Pep groups (33.3% and 42.8%, respectively) when compared with the sham group (66.6%) (). Hence, and as expected, a 50%-fold reduction in T1D incidence was achieved in the PSAB-lipo group. The groups treated with liposomes encapsulating IA2, GAD65 or C-peptide did not show any reduction in the incidence of the disease (80%, 65% and 83.3% respectively) when compared with sham-treated animals (). Moreover, the group treated with the mix of the peptide-filled liposomes did not show a decrease in the incidence either (80%) (). In order to confirm that mice at the end of follow-up did not develop T1D, urine glucose concentration (glycosuria) and serum C-peptide concentration –as indicators of β-cell function– were assessed. All mice showed negative glycosuria and detectable C-peptide concentration (range 900–3000 pg/mL). No statistical differences were found between groups (data not shown).
Figure 1. T1D incidence in NOD mice treated with PS-liposomes filled with different autoantigenic peptides. Cumulative incidence (percentage) of T1D in NOD mice treated with IA2 liposomes (PSIA2-lipo, blue, n = 5), GAD65 liposomes (PSGAD65-lipo, red, n = 6), C-peptide liposomes (PSCpep-lipo, yellow, n = 6), insulin liposomes (PSAB-lipo, grey, n = 6), a mixture of all liposomes (Cock-lipo, green, n = 5) and a mixture of all non-encapsulated peptides (Cock-Pep, black, n = 7). A sham group was also included (violet, n = 9). The arrow indicates the time-point of the single-dose administration (at 8 weeks). No significant differences in T1D incidence were found between groups (Mantel-Cox Log-Rank). PSIA2-lipo: IA2 liposomes; PSGAD65-lipo: GAD65 liposomes; Cock-lipo: a mixture of all liposomes; PSCpep-lipo: C-peptide liposomes; Cock-Pep: a mixture of all non-encapsulated peptides; PSAB-lipo: insulin liposomes.
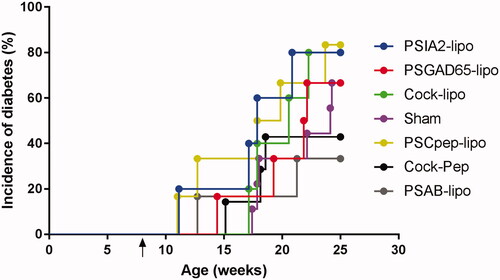
Table 2. Features of the liposomes used in the study.
Table 3. Amount of peptide encapsulated in a dose (200 µL) of PS-liposomes (3.5 mg lipid).
PS-liposomes encapsulating insulin peptides reduce the severity of the autoimmune islet destruction
Given the reduced incidence of T1D in both PSAB-lipo and Pep-Cock treated mice, islet leukocytic infiltration was determined at the end of the follow-up period showing a biological reduction of insulitis, although non-significant, in both treated groups (PSAB-lipo: 1.45 ± 0.57 and Cock-Pep: 1.32 ± 0.34) when compared to sham mice (1.85 ± 0.60) (. Furthermore, the analysis of the percentages of islets classified in each infiltration category revealed that in both treated groups more than 64% of the islets remained insulitis-free (type 0) or with benign insulitis (type 1); in the control group, 48% of the islets were scored with destructive insulitis (type 2, 3 and 4) ().
Figure 2. Islet leukocytic infiltrate (insulitis) following administration of nanotherapy. (A) Insulitis score from non-diabetic mice at the end of the follow-up period at 25 weeks, including the PSAB-lipo (n = 3), Cock-Pep (n = 3) and sham (n = 3) groups. Results are mean ± Standard Deviation (SD). No statistical differences were found between groups (Mann-Whitney test). (B) Percentage of islets in each of the infiltration categories: White = 0, no insulitis; Dotted = 1, peri-insular; Striped = 2, mild insulitis (<25% of the infiltrated islet); Squared = 3, 25–75% of the islet infiltrated; Black = 4, >75% islet infiltration. (C) Representative images of insulitis in islets from H/E-pancreatic cryostat sections (5 µm) from the three different groups. Scale bar represents 100 µm. PSAB-lipo: insulin PS-liposomes; Cock-Pep: mixture of all non-encapsulated peptides.
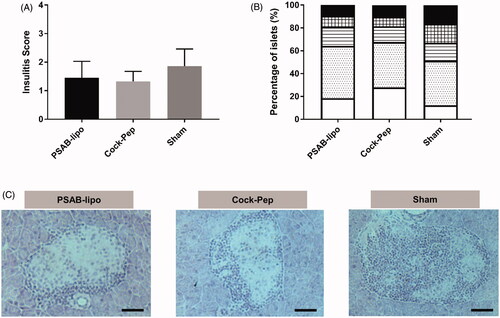
PS-liposomes treatment demonstrated optimal safety and tolerability in NOD mice
The observational study, taking into account the criteria used in welfare monitoring of mice used for toxicity and tolerability assays (Supplementary Table S1), demonstrated the safety of daily PS-liposomes administration in NOD mice. Only mild complications were observed both in daily PS-liposomes-treated and sham group () and no animal had to be euthanised due to severe complications of the treatment. All mice were negative for glucosuria during the 14 days of treatment and 7 days after withdrawal. The analysis of the serum showed no significant differences between PS-liposomes and sham groups at the end of the study (). Total CH, HDL CH, triglycerides and ALT concentrations remained unaltered and within reference values for NOD mice [Citation13]. Urea concentration in both the groups was found above reference values. Creatinine concentration was similar in both groups, but reference values were not available.
Figure 3. Effect of daily administration of PS-liposomes on blood biochemical parameters of NOD mice at the end of the study (Day 21). In vivo toxicity assay by measuring the levels of (A) Total CH, mg/dL, (B) HDL CH, mg/dL), (C) triglycerides (mg/dL), (D) ALT, U/L, (E) urea, mg/dL and (F) creatinine, mg/dL in serum of PBS-treated mice (n = 4, squares) and PS-liposomes-treated mice (n = 4, circles), at the end of the study (Day 21). Continuous lines represent the mean of each group. The grey area depicts normal ranges of parameters and the median (discontinuous line) of the different analytes for NOD mice. Significant differences were not found between groups (Mann-Whitney test). CH: cholesterol; ALT: Alanine transferase.
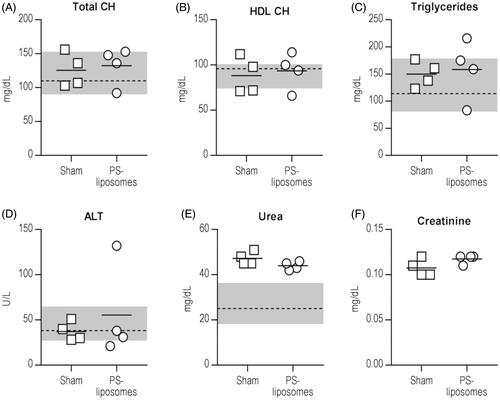
Table 4. NOD mice daily treated with PS-liposomes (14 days) with disturbances.
Discussion
This study shows that the administration of PS-liposomes encapsulating insulin peptides is the best strategy to reduce the incidence of T1D, spontaneously developed in NOD mice, when compared to other encapsulated autoantigen peptides (GAD65, IA2 and C-peptide). Liposomes are vesicles composed of lipid bilayers that can be used as a vehicle for drug delivery. Considering that the physicochemical features of the liposomes are homogeneous, and that empty liposomes have no effect on T1D and other autoimmune diseases [Citation8,Citation14], the differences here reported can be due to the biological features of the encapsulated autoantigens. The role of the different autoantigens in the stages of autoimmunity in T1D is also relevant. On the one hand, insulin is the most expressed specific autoantigen in the target β-cells on which the autoimmune reaction is focussed and, on the other hand, single positivity for autoantibodies against insulin but not for the rest of autoantigens is associated with the greatest risk to develop T1D [Citation15]. Moreover, it has been described that insulin is an early autoantigen in T1D whereas the other autoantigens seem to be the consequence of epitope spreading, after the activation of autoimmunity [Citation15]. IA2 expression is not exclusive of pancreatic β-cells in humans nor rodents, being found expressed also in many neuroendocrine cells [Citation16–18]. This lack of specificity would explain the unsuccessful therapeutic use by its single encapsulation in PS-liposomes. GAD65 expression is also present in other tissues due to its role in the biosynthesis of gamma-aminobutyric acid (GABA) like GABAergic cells [Citation19]. Furthermore, it has been recently reported that GAD65 has no capacity to prevent T1D in wild type NOD mice [Citation20]. Finally, the role of C-peptide as autoantigen in T1D remained controversial despite recent work hints at its action as an autoantigen [Citation21–23]. This work validates the use of PS-liposomes encapsulating insulin peptides to prevent the development of autoimmune T1D and tends to reduce the insulitis in the experimental NOD model [Citation8].
A different encapsulation efficiency has been observed for the different peptides and this fact must be taken into account to both understand the results in this study and to design future nanotherapies. The lowest percentage of encapsulation corresponds to liposomes encapsulating C-peptide and, as mentioned above, the role of this molecule as autoantigen remains unclear. Both facts may be the reason of the lack of effect in T1D prevention. Moreover, the mixture of liposomes (Cock-lipo) contains a 7-fold reduction of the total insulin peptide amount carried in the PSAB-lipo, resulting in a loss of therapeutic action.
Apart from the nanotherapy described here, a mixture of the selected autoantigens (Cock-Pep) was found to reduce the incidence of the disease when compared with the sham group but with a minor effect than the observed with PS-liposomes encapsulating insulin. The synergistic effect of PS in the liposomes and the encapsulated peptide has been described in previous work showing a tolerogenic effect mediated by DCs [Citation8]. Many therapies have been tested both for prevention and treatment of T1D in the NOD mice based on peptides [Citation24]. The here reported effect of combined peptides fits well with previous data showing a preventive effect of T1D after peptide administration of insulin, GAD65 and IA2 in NOD mice and in other autoimmune models [Citation25,Citation26].
This works shows that the choice of autoantigen and derived peptides is key in the design of vesicles for T1D immunotherapies. Despite that, questions still remain about the therapeutic contribution of each peptide and about the molecular mechanisms involved in their immune-recognition. Of note, the timing of administration of nanotherapies to arrest spontaneous autoimmunity in T1D plays an essential role in the success of the immunotherapy. Moreover, the best peptide option for encapsulation in nanovesicles will probably depend not only on the checkpoint of intervention but also on the immunophenotype (specific autoantibodies and autoreactive T cells) of each patient.
A vital issue for the successful implementation of the PS-liposomes immunotherapy in the clinics is its potential toxicity and tolerability. The daily injections of PS-liposomes during two weeks, revealed a well-tolerated and safe profile in NOD mice. The minor complications found among both groups are probably due to the stress of the injections the mice received daily. Also, the loss of 10% of body weight reported in mice in both the groups suggests that these mice were on the road to developing diabetes. The analysis of the serum confirmed the safety of the treatment.
We are fully aware that they are preliminary results and that a low number of mice have been analysed. Further studies are required to validate the tendencies observed in a larger group of mice and over a longer period of time, and to determine the effect of the therapy in several tissues by histological analyses. However, the effect of only one dose of PS-liposomes encapsulating autoantigens is clear and resulted in T1D prevention without side-effects, providing a simple and innovative system to recover self-tolerance.
Supp_Table_1_1_.docx
Download MS Word (13.7 KB)Acknowledgements
We acknowledge Ms. Deborah Cullell-Young for English grammar assistance. Special thanks to Ms. Laia Gomez-Muñoz for aid in collecting microscope images.
Disclosure statement
MVP, MCS and DM hold patents that relate to liposomes and are co-founder of Ahead Therapeutics S.L. The other authors report no conflicts of interest in this work.
Additional information
Funding
References
- Michels AW, Landry LG, McDaniel KA, et al. Islet-Derived CD4 T cells targeting proinsulin in human autoimmune diabetes. Diabetes. 2017;66(3):722–734.
- Noble JA. Immunogenetics of type 1 diabetes: a comprehensive review. J Autoimmun. 2015;64:101–112.
- Steck AK, Vehik K, Bonifacio E, et al. Predictors of progression from the appearance of islet autoantibodies to early childhood diabetes: The Environmental Determinants of Diabetes in the Young (TEDDY). Dia Care. 2015;38(5):808–813.
- Lampasona V, Liberati D. Islet autoantibodies. Curr Diab Rep. 2016;16(6):53.
- Steinman RM, Turley S, Mellman I, et al. The induction of tolerance by dendritic cells that have captured apoptotic cells. J Exp Med. 2000;191(3):411–416.
- Hochreiter-Hufford A, Ravichandran KS. Clearing the dead: apoptotic cell sensing, recognition, engulfment, and digestion. Cold Spring Harb Perspect Biol. 2013;5(1):a008748–a008748.
- Marin-Gallen S, Clemente-Casares X, Planas R, et al. Dendritic cells pulsed with antigen-specific apoptotic bodies prevent experimental type 1 diabetes. Clin Exp Immunol. 2009;160(2):207–214.
- Pujol-Autonell I, Serracant-Prat A, Cano-Sarabia M, et al. Use of Autoantigen-Loaded Phosphatidylserine-Liposomes to arrest autoimmunity in Type 1 diabetes. Plos One. 2015; 10(6): e0127057.
- Makino S, Kunimoto K, Muraoka Y, et al. Breeding of a non-obese, diabetic strain of mice. Jikken Dobutsu. 1980;29(1):1–13.
- Chen Y-G, Mathews CE, Driver JP. The role of NOD mice in type 1 diabetes research: lessons from the past and recommendations for the future. Front Endocrinol. 2018;9:51.
- Harel-Adar T, Mordechai T, Ben Amsalem Y, et al. Modulation of cardiac macrophages by phosphatidylserine-presenting liposomes improves infarct repair. Proc Natl Acad Sci USA. 2011;108(5):1827–1832.
- Alba A, Puertas MC, Carrillo J, et al. IFN beta accelerates autoimmune type 1 diabetes in nonobese diabetic mice and breaks the tolerance to beta cells in nondiabetes-prone mice. J Immunol. 2004;173(11):6667–6675.
- Zúñiga JM, Orellana Muriana JM, Tur Marí JA, Ciencia y tecnología del animal de laboratorio. Universidad de Alcalá. 2013;1:151–182.
- Pujol-Autonell I, Mansilla M-J, Rodriguez-Fernandez S, et al. Liposome-based immunotherapy against autoimmune diseases: therapeutic effect on multiple sclerosis. Nanomedicine. 2017;12(11):1231–1242.
- Nakayama M. Insulin as a key autoantigen in the development of type 1 diabetes. Diabetes Metab Res Rev. 2011;27(8):773–777.
- Giannopoulou EZ, Winkler C, Chmiel R, et al. Islet autoantibody phenotypes and incidence in children at increased risk for type 1 diabetes. Diabetologia. 2015;58(10):2317–2323.
- Solimena M, Dirkx R, Hermel JM, et al. ICA 512, an autoantigen of type I diabetes, is an intrinsic membrane protein of neurosecretory granules. Embo J. 1996;15(9):2102–2114.
- Takeyama N, Ano Y, Wu G, et al. Localization of insulinoma associated protein 2, IA-2 in mouse neuroendocrine tissues using two novel monoclonal antibodies. Life Sci. 2009;84(19–20):678–687.
- Mally MI, Cirulli V, Otonkoski T, et al. Ontogeny and tissue distribution of human GAD expression. Diabetes. 1996;45(4):496–501.
- Funda DP, Goliáš J, Hudcovic T, et al. Antigen loading (e.g., Glutamic Acid Decarboxylase 65) of Tolerogenic DCs (tolDCs) reduces their capacity to prevent diabetes in the Non-Obese Diabetes (NOD)-severe combined immunodeficiency model of adoptive cotransfer of diabetes as well as in NOD mice. Front Immunol. 2018;9:290.
- Mallone R, Brezar V, Boitard C. T cell recognition of autoantigens in human type 1 diabetes: clinical perspectives. Clin Dev Immunol. 2011;2011:1.
- Rudy G, Stone N, Harrison LC, et al. Similar peptides from two beta cell autoantigens, proinsulin and glutamic acid decarboxylase, stimulate T cells of individuals at risk for insulin-dependent diabetes. Mol Med. 1995;1(6):625–633.
- So M, Elso CM, Tresoldi E, et al. Proinsulin C-peptide is an autoantigen in people with type 1 diabetes. Proc Natl Acad Sci USA. 2018;115(42):10732–10737.
- Peakman M, Dayan CM. Antigen-specificc immunotherapy for autoimmune disease: fighting fire with fire? Immunology. 2001;9:290.
- Fierabracci A. Peptide immunotherapies in Type 1 diabetes: lessons from animal models. Curr Med Chem. 2011;18(4):577–586.
- Shen L, Lu S, Huang D, et al. A rationally designed peptide IA-2-P2 against type 1 diabetes in streptozotocin-induced diabetic mice. Diabetes Vasc Dis Res. 2017;14(3):184–190.