Abstract
In this study, we have designed a magnetic targeting pro-coagulant protein (MTPCP) for the embolic therapy of solid tumours. The MTPCP consists of a magnetic carrier and a pro-coagulant protein. The pro-coagulant protein used in this study is the fusion protein tTF-EG3287 which is not pro-coagulant when free in the blood circulation, but presents strong pro-coagulant ability once bound to the Neuropilin-1(NRP-1) that is highly expressed on tumour-associated vascular endothelial cells. And the magnetic carrier is O-Carboxymethyl chitosan-coated iron oxide nanoparticles (OCMC/Fe3O4). In vitro, we assessed the NRP-1 targeting ability of the MTPCP using confocal microscopy and flow cytometry, and evaluated the potential pro-coagulant activity of the MTPCP using the Spectozyme FXa assay. In vivo, the magnetic targeting ability of the MTPCP was detected using a living imaging system. At last, we assessed the anticancer activity of the MTPCP on HepG2 tumour bearing BALB/c nude mice models including subcutaneous transplantation and orthotopic transplantation. HepG2 tumour bearing mice models revealed that after intravenous administration of the MTPCP, thrombosis specifically occurs on tumour-associated blood vessels, and resulting in tumour growth retardation. No apparent side effects, such as thrombosis in other organs or other treatment-related toxicity, were observed during the treatment. Our data showed that the MTPCP may be a promising embolic agent for the embolic therapy of solid tumours.
Introduction
Embolic therapy is a promising treatment option for advanced cancer. Embolic agents cause a fast and selective occlusion of the tumour blood vessels, and lead to subsequent tumour cell demise because of ischaemia. Vascular occlusion could be accomplished using different embolic agents such as gelatine sponge, starch microspheres, polyvinyl alcohol beads, or non-biodegradable polymer like polyvinyl alcohol (PVA) [Citation1,Citation2]. However, clinical practice, there are some non-negligible disadvantages of conventional embolic agents to consider, including the possible toxicity of the materials, undesirable by-products of degradation, patient discomfort from the delivery device, and the high cost and complex operation of controlled-release systems. Pro-coagulant protein is a potential embolic agent, which is not an embolic material itself, but could block the blood supply by inducing thrombosis in the tumour-supplying blood vessels [Citation3].
It is a well-known fact that the tissue factor (TF) is the activator of the blood coagulation cascades. TF is a transmembrane protein and consists of three domains: an extracellular domain, a transmembrane domain and a cytosolic domain [Citation4,Citation5]. Huang et al. [Citation6] constructed a recombinant form of TF, truncated tissue factor (tTF), which only contains the extracellular domain of TF. The pro-coagulant activity of tTF is about five orders of magnitude less than that of native transmembrane TF, but it would recovery pro-coagulant activity once bound to a cell surface by a targeting ligand [Citation7–11]. Which means the thrombosis (blood clot) induced by tTF is exactly and strictly on the vessel that tTF targeted and bound. Therefore, tTF is an ideal and safe embolic agent for embolic therapy of tumour. Given the advantages of tTF as an embolic agent for embolic therapy of tumour, we designed a magnetic targeting pro-coagulant protein (tTF-EG3287@OCMC/Fe3O4), which consist of the fusion protein tTF-EG3287 and the drug delivery system O-Carboxymethyl chitins coated Fe3O4 nanoparticles (OCMC/Fe3O4).
This novel fusion protein tTF-EG3287 consisting of tTF and a short peptide EG3287 that targeting Neuropilin-1(NRP-1) was designed and constructed by our laboratory [Citation12]. NRP-1 is a co-receptor of a tyrosine kinase receptor for both vascular endothelial growth factor (VEGF) and Semaphorin-3A (SEMA3A) [Citation13]. As a co-receptor to VEGF, NRP-1 has been confirmed high expression not only on tumour tissue but also on tumour vessels [Citation14–17]. Therefore, NRP-1 is a potential and desirable target for tumour vascular targeted therapy. A short peptide EG3287 targeting NRP-1 was synthesised in 2005 by Jia et al. [Citation18]. In this study, the fusion protein, tTF-EG3287, was prepared using genetic engineering techniques. O-carboxymethyl chitins coated Fe3O4 nanoparticles (OCMC/Fe3O4) as a fitting drug delivery system have been reported previously [Citation19–21]. Its high drug delivery effect and low toxicity have been confirmed [Citation22].
In this study, we successfully constructed the magnetic targeting pro-coagulant protein (MTPCP). The mechanism of the MTPCP was shown in . The MTPCP was injected into the blood circulation through the tail vein. After intravenous administration, we placed a magnet exactly on tumour area. OCMC/Fe3O4 nanoparticles as a carrier, transports the fusion protein tTF-EG3287 towards to the tumour vessels. Consequently, the pro-coagulant protein tTF-EG3287 could be quickly enriched in the tumour vessels, and then bound to NRP-1 of tumour vascular endothelial cells by targeting peptide EG3287. The membrane-bound tTF then recovers its pro-coagulant activity and triggers the blood coagulation cascade, resulting in thrombosis and consequently blood-supply blockage, tumour vessel infarction, tumour cell necrosis and tumour growth inhibition.
Materials and methods
Materials and reagents
Ferric chloride (FeCl3·6H2O), Ferrous sulphate (FeSO4·7H2O) and Carbodiimide (EDC) were purchased from Shanghai Sangon Biotech Company (China). N-Hydroxysulfosuccinimide sodium salt (sulfo-NHS) and O-Carboxymethyl chitosan (OCMC) were purchased from Aladdin Chemicals (China). Dulbecco’s modified Eagle’s medium (DMEM) and foetal bovine serum were purchased from Invitrogen-Life Technologies (USA). Endothelial Cell Growth Medium (ECM) was purchased from Sciencell Research Laboratories (USA). Hoechest and RBITC fluorescent dyes were purchased from Shanghai Sangon Biotech Company (China). Nickel-nitrilotriacetic acid (Ni-NTA) agarose was purchased from Qiagen (USA). Ultrasound Bacteriolysis Buffer, Inclusion body Purgation buffer, Inclusion body solubilisation buffer were purchased from Huiyan Biotech Company (China).
Cell lines and cell culture
Human liver carcinoma cell line (HepG2) and Human umbilical vein endothelial cell line (HUVEC) were frozen by the Medical College of Xiamen University. HepG2 were cultured with DMEM medium supplemented with 10% foetal bovine serum and 1% penicillin/streptomycin. HUVEC were cultured endothelial cell medium which consists of 5% foetal bovine serum, 1% endothelial cell growth supplement and 1% penicillin/streptomycin. Both the cell lines were cultivated in cell incubator (Thermo Scientific) with a humidified atmosphere of 37 °C and 5% CO2.
Cloning, expression, purification and identification of the fusion protein tTF-EG3287
The expression vector pET-22b (+) encoding the fusion proteins tTF-EG3287 has been successfully constructed in our laboratory. The DNA sequence of tTF-EG3287 is shown in Supplementary Figure S1. The process of cloning, expression, purification of the fusion protein tTF-EG3287 refer to the methods reported earlier [Citation23,Citation24]. Briefly, we first transformed the expression plasmid into E. coli (BL21, DE3). And then bacteria were cultured in LB medium supplemented with 0.1 mg/mL of antibiotic ampicillin in an orbital shaker at 350 rpm at 37 °C until optical density of 0.6–0.8 at 600 nm. At this point, IPTG (isopropyl-thio-galactoside) was added to a final concentration of 0.5 mM and the culture was continued for another 6 h. After induced by IPTG, E. coli samples were collected and disrupted. The precipitate obtained from 12,000g high-speed centrifugation for 30 min was the inclusion body. And then, washed the inclusion body three times with inclusion body purgation buffer. The washed inclusion bodies were dissolved in inclusion body solubilisation buffer and purified by nickel affinity column. The purification process was verified by 12% SDS-PAGE. Finally, the purified recombinant protein was renatured by stepwise dialysis and finally dissolved in phosphate buffer saline (PBS). The fusion protein was concentrated using ultrafiltration tube (Millipore). Bicinchoninic acid assay (BCA) was used to measure the concentration of the fusion protein. Meanwhile, the tTF protein (without targeting peptide EG3287) was prepared as control.
Synthesis and characterisation of the magnetic targeting pro-coagulant protein
A brief description of the process of synthesis of the MTPCP is shown in . Firstly, Single core Fe3O4 nanoparticles with an average particle size of 6–10 nm were prepared using controlled co-precipitation as reported [Citation25,Citation26]. Briefly, the mixture of FeSO4·7H2O and FeC13·6H2O at a molar ratio of 1:2 (Fe2+: Fe3+) was prepared with deionised water in a three-necked flask. Then, slowly heating the reaction mixture to 80 °C., NH4OH (25%) was quickly dropped into the above reaction mixture stirring to the controlled pH at 10–11. The reaction was carried out for 30 min under N2 protection to prevent critical oxidation. The particles were collected by sedimentation with the help of an external magnetic field and washed with deoxygenated water and ethanol.
Surface modification of Fe3O4 nanoparticles was carried out via silanization to introduce amine groups as reported [Citation27]. Firstly, 100 mg of Fe3O4 nanoparticles were washed once with 20 mL of methanol, 20 mL of a mixture of methanol and toluene (1:1, v/v), and 20 mL of toluene, successively. Fe3O4 nanoparticles were then dispersed into 20 mL of toluene and 0.5 mL of APTMS [3 mM in a methanol/toluene (1:1, v/v) mixture] was added to the suspension. The suspension was further refluxed at 110 °C for 24 h under a N2 flow and vigorous stirring. The modified particles were magnetically collected, washed with methanol three times, and dried in a vacuum.
The O-Carboxymethyl chitosan coated Fe3O4 nanoparticles (OCMC/Fe3O4) was prepared as follows: 50 mg of NH2-modified Fe3O4 nanoparticles were added to 10 mL of aethanesulfonic acid buffer (MES; 0.1 M, pH = 6.5) containing 25 mg of OCMC, carbodiimide (EDC; 20 mM), and N-hydroxysulfosuccinimide (sulfo-NHS; 50 mM). The mixture was then sonicated for 10 min at 4 °C and shaken for 24 h at room temperature. The Carboxymethyl chitosan coated Fe3O4 nanoparticles were collected under an external magnetic field and washed with ethanol. An ultrafiltration centrifuge tube (Millipore) was used to concentrate the OCMC/Fe3O4.
At last, 1 mL (2 mg) of the fusion protein tTF-EG3287 was added into 1 mL of OCMC/Fe3O4 and dispersed by mechanical stirring at 4 °C. After fully mixing, carbodiimide (EDC; 20 mM) and and N-hydroxysulfosuccinimide (sulfo-NHS; 50 mM) were added into the reaction system and shaken for 24 h at room temperature. The MTPCP (tTF-EG3287@OCMC/Fe3O4) was collected under an external magnetic field and purified by HS300R dextran gel column and dialysed in PBS at 4 °C refrigerator for 24 h to remove the extra small molecule of reaction material (Fe3O4 and OCMC). Finally, the reaction product was concentrated by ultrafiltration centrifuge tube (Millipore).
The physical properties of the above products were analysed by transmission electron microscope (TEM), Malvern particle size analyser, Fourier transform infra-red spectroscopy (FTIR), X-ray diffraction (XRD) and Superconducting quantum interference devices (SQUID).
Biological functions of the magnetic targeting pro-coagulant protein
The MTPCP was designed to contain three critical biological characteristics: NRP-1 targeting ability, pro-coagulant activity and less toxic.
NRP-1 targeting ability
The NRP-1 targeting ability was assessed by confocal microscopy and flow cytometry. Previous studies confirmed that NRP-1 is highly expressed on HUVEC cell [Citation28–30]. So, we selected HUVEC cell line as the research object. First, the fusion protein tTF-EG3287 was labelled with fluorescent rhodamine B isothiocyanate (RBITC) according to the manufacturer’s protocol [Citation31]. We assess the amount of fluorescein bound to the protein by F/P value which is the ratio of the absorbance in a cuvette with a 1-cm pathlength at 494 nm (A555) and 280 nm (A280). And then using RBITC labelled fusion protein, RBITC-tTF-EG3287, to prepare the RBITC-MTPCP as described above. RBITC labelled MTPCP was co-cultured with HUVEC cell for 30 min. Then detect fluorescence intensity of the cell surface by confocal microscopy and flow cytometry after washed by PBS. Meanwhile, RBITC labelled tTF@OCMC/Fe3O4(without targeting peptide) with same protein concentration and F/P value was prepared as a control.
Pro-coagulant activity
The pro-coagulant activity of the MTPCP was assessed using Spectozyme FXa assay described by Ruf et al. and Hische et al. [Citation7,Citation32]. Briefly, 20 μL of different concentrations (0.01 ∼ 20 μmol/L) of BSA, tTF, tTF-EG3287, OCMC/Fe3O4 and tTF-EG3287@OCMC/Fe3O4 mixed with 20 μL of recombinant factor VIIa(100 nmol/L) in Tris-buffer saline were added to each well in a microtiter assay plate, and incubated at 37 °C for 10 min. And then, 20 μL of Factor X in a concentration of 10 nmol/L was added to the reaction, and subsequently incubated at 37 °C for another 10 min, to which 10 μL EDTA in a concentration of 100 mmol/L was added to quench the reaction. From that point onward, 10 μL of chromogenic substrate S2222 was added, and the plate reading was done at 405 nm in five minutes. Factor Xa activated by tTF or tTF-EG3287 was evaluated by OD value at 405 nm.
Cytotoxicity test (CCK-8 assay)
The cytotoxicity of the MTPCP was tested using CCK-8 kit. Two kinds of cells, HepG2 and HUVEC, are cultured to the exponential growth phase according to the above method. And then cells were digested by trypsin and re-suspended by fresh medium. 200 μL of cells suspension at densities of 1 × 105 cells/well was dispensed in 96-well plate, 6 replicate wells were set in each group, continuing to culture for 24 h. After the cells were attached, replaced fresh medium contained different concentrations of (5, 102, 550, 100, 200 μg/ml) the tTF-EG3287@OCMC/Fe3O4 which calculated based on protein concentration, and incubated for 24 h. 10 μL of CCK-8 solution was added to each well of the plate after replaced 200 μL fresh medium and incubate the plate for 3 h in the incubator. The absorbance at 450 nm was measured using a microplate reader.
Establishment and grouping of tumour model mice
Six-week-old female BALB/c nude mice were purchased from the Animal Centre of Xiamen University (Fujian, China). In this study, subcutaneous transplantation tumour models and orthotopic transplantation hepatic carcinoma models in BALB/c nude mice were constructed referenced reported methods [Citation33–35].
Treatment strategy and grouping are shown in , mice were injected 0.1 mL of saline, 0.1 mL of tTF-EG3287 (0.5 mg/mL), 0.1 mL of OCMC/Fe3O4 and 0.1 mL of magnetic targeting pro-coagulant protein with the protein concentration of 0.5 mg/mL via the tail vein. In the OCMC/Fe3O4 group and the MTPCP Group, tumour areas of mice were exposed to magnetic radiation for 30 min immediately after administration.
Table 1. Treatment strategy and grouping of experiments in mice.
Magnetic targeting ability of the magnetic targeting pro-coagulant protein
Living imaging system was used to assay magnetic targeting ability of the MTPCP in vivo. Magnetic targeting ability means that the MTPCP can be enriched in tumour vessels by applying extra magnetic field on tumour area after tail vein administration. As shown in , randomly divided 12 of HepG2 tumour bearing nude mice into 4 groups. The proteins on the tTF-EG3287 group and the MTPCP group were labelled with fluorescently Cy5.5. The ratio of the absorbance in a cuvette with a 1-cm pathlength at 650 nm (A650) and 280 nm (A280) used to calculate the F/P value. After administration, we placed a neodymium magnet on the cancer area of mice on the OCMC/Fe3O4 group and the MTPCP group for 30 min immediately. Living imaging system was performed at 30 min, 1 h, 2 h, 4 h, 6 h, 12 h, 24 h,48 h and 72 h after administration by Imaging IVIS-200 system (Caliper Life Sciences) to detect the distribution of drugs in vivo.
Antitumor activity of the magnetic targeting pro-coagulant protein in subcutaneous transplantation tumour models
The antitumor activity of the MTPCP was assessed by the tumour growth inhibition test that measured the tumour volume of BALB/c nude mice. Grouping and administration strategies as shown in , fourty of HepG2 subcutaneous transplantation tumour bearing BALB/c nude mice with similar tumour volumes were selected and randomly divided into 4 groups of 10 mice each. After continuous administration for 4 days and continuously measured tumour volume and body weight of each mouse for 7 days, mice were euthanized. Tumour tissues were weighed after blunt dissection on the seventh day. The tumour volumes were calculated according to the formula (volume = length × width2/2). The pooled results were calculated by two independent experiments. Kruskal-Wallis test followed by a Mann–Whitney U-test were used to compare the difference of the tumour volume and weight between the treatment group and the control groups. Two-sided p value <.05 were considered statistically significant.
Treatment effect of the magnetic targeting pro-coagulant protein in orthotopic transplantation models
We constructed orthotopic transplantation tumour model of hepatocellular carcinoma in mice as mentioned above. After successful establishment of HCC models, 12 of BALB/c nude mice models were randomly divided into four groups. The grouping and treatment strategy are shown in . In addition, in the OCMC/Fe3O4 group and the MTPCP group, we placed a neodymium magnet on the liver area for 30 min immediately after administration. All drugs were slowly applied intravenously via the tail veins for consecutive 4 days. Hepatic carcinoma tissues were taken out after euthanasia on the seventh day, and prepared pathological section for histological observation of treatment effect.
Results
Cloning, expression, purification and identification of the fusion protein tTF-EG3287
The SDS-PAGE analysis () showed a specific band about 35 kDa increased with induction time (0–6 h), which is consistent with the atomic mass of theoretical molecular weight of 32.3 kDa calculated by Expasy’s protparam server. The purification process of the fusion protein was monitored by SDS-PAGE analysis as shown in , the crude cell lysate before purification (lane 1) contained many hetero-proteins. After purification, the purity of the fusion protein is over 99% (lane 5), which determined by GelDoc XR system (BioRad, US). After purification, the fusion protein was confirmed by western blot analysis using His Tag monoclonal antibody. As shown in , migration size of protein was detected at about 35 kDa, which demonstrated no discrepancy compared to the SDS-PAGE analysis. The standard curve of BCA assay is shown in . The OD values at 562 nm of fusion protein tTF-EG3287 after purification and concentration was 1.836. According to the above standard curve, the protein concentration of tTF-EG3287 was about 2.2 mg/mL. In summary, the fusion protein tTF-EG3287 with high purity (over 99%) and high concentration (over 2 mg/mL) was prepared successfully.
Figure 3. Identification of the fusion protein tTF-EG3287. (A) Analysis of different induction time (0–6 h) of the fusion protein tTF-EG3287 by Coomassie-stained SDS-PAGE. (B) Purification of the fusion protein. The proteins were purified from E. coli by use of nickel column chromatography. Shown is a crude lysate (Lane1), column flow-through (Lane2), wash (Lane3,4), and elution(Lane5) for the fusion protein tTF-EG3287. (C) Western blot analysis of the purified fusion protein samples, using an anti-His-Tag antibody. (D) The standard curve of the BCA assay.
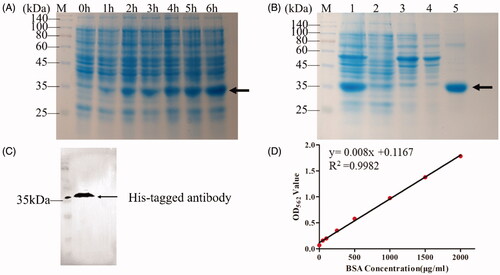
Characterisation of the magnetic targeting pro-coagulant protein
The summarised results of the characterisation are presented in . The TEM images () showed that the size of the Fe3O4(a), OCMC/Fe3O4(b) and tTF-EG3287@OCMC/Fe3O4(c) was almost uniform and most of them are approximately spherical with diameter 10 nm, which is consistent with the result of the Malvern particle size analyser (.
Figure 4. Characterisation of Fe3O4, OCMC/Fe3O4 and tTF-EG3287@OCMC/Fe3O4. (A) Transmission electron microscope image of Fe3O4(a), OCMC/Fe3O4(b) and tTF-EG3287@OCMC/Fe3O4(c), scale bar = 50nm; (B)The particle size analysis of Fe3O4(a), OCMC/Fe3O4 (b) and tTF-EG3287@OCMC/Fe3O4 (c) calculated by Malvern laser diffraction analyser; (C) X-ray diffraction (XRD) patterns of Fe3O4(a), OCMC/Fe3O4(b) and tTF-EG3287@OCMC/Fe3O4(c); (D) Fourier transform infra-red (FTIR) spectra of Fe3O4(a), OCMC(b) and OCMC/Fe3O4(c); (E) Magnetisation curve for the uncoated at Fe3O4(a), OCMC/Fe3O4(b) and tTF-EG3287@OCMC/Fe3O4(c) at 150K. (F) Stability analysis of Fe3O4(a), OCMC/Fe3O4(b) and tTF-EG3287@OCMC/Fe3O4(c) after standing for 24 h.
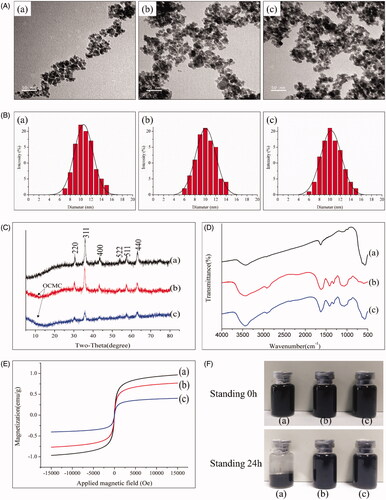
The crystal structures of Fe3O4, OCMC/Fe3O4 and tTF-EG3287@OCMC/Fe3O4 are shown in . The XRD patterns indicate iron oxide cubic structure and six peaks in 2θ of 30.1, 35.5, 43.2, 53.5,57.0 and 62.8, which were related to their corresponding indices (220), (311), (400), (422), (511) and (440), respectively. The positions and relative intensities of the diffractograms agree well with those XRD patterns of Fe3O4 in some reported studies [Citation36,Citation37], which confirmed the structure of the magnetite materials (JCPDS card no.79-0418). The weaker diffraction lines (arrow) of OCMC/Fe3O4 and tTF-EG3287@OCMC/Fe3O4 compared with Fe3O4 nanoparticles indicate that the Fe3O4 nanoparticles were covered by amorphous and retain the crystal structure.
The FTIR spectra of Fe3O4(a), OCMC(b)and OCMC/Fe3O4(c) are shown in . The characteristic peaks of Fe3O4 at 564 cm−1 and 584 cm−1 were found in curve(a) and (c). The absorption centred at 564 cm−1 and 584 cm−1 corresponding to the Fe-O vibration related to the magnetite phase. The characteristic peaks of OCMC at 1408 cm−1 and 1411 cm−1 are found in curves (b) and (c), which were (C-O) of the carboxyl groups. Compared with curve(a) and (c), The band shifts of Fe–O stretching from 564 cm−1 and 584 cm−1and of C–O bending vibration from 1408 to 1411 cm−1 are significant. This phenomenon (blue shifted) indicates that OCMC/Fe3O4 combined by chemical cross linking with the formation of new bonds between the Fe3O4 and OCMC.
The close hysteresis loops show the magnetisation curves for Fe3O4, OCMC/Fe3O4 and tTF-EG3287@ OCMC/Fe3O4 in . Due to their coercivity near to zero, Fe3O4, OCMC/Fe3O4 and tTF-EG3287@OCMC/Fe3O4 contained the superparamagnetic property, which is the feature of Fe3O4 nanoparticles. Thus, such a magnetic property for magnetic targeting pro-coagulant protein is robust enough to be quickly and easily separated from the reaction mixture by a magnetic field. The saturation magnetisation value (Ms) of pure Fe3O4 was 77.3 emu/g, which is higher than OCMC/Fe3O4 and tTF-EG3287@OCMC/Fe3O4. This might be explained by the OCMC polymer shell barriers.
Suspension stability analyses of Fe3O4(a), OCMC/Fe3O4(b)and tTF-EG3287@OCMC/Fe3O4(c) are shown in . Before standing, there is no obvious difference between the three. After standing for 24 h, Fe3O4(a) precipitated at the bottom of the solution. While, OCMC/Fe3O4(b) and tTF-EG3287@OCMC/Fe3O4(c) are not very different before standing. This result indicated that surface modification of Fe3O4 by OCMC could improve dispersion stability of Fe3O4 nanoparticles in water, and the dispersion stability of OCMC/Fe3O4(b) would not reduce after cross-linking the fusion protein(c).
In conclusion, the magnetic targeting pro-coagulant protein tTF-EG3287@OCMC/Fe3O4 was successfully prepared. And the final protein concentration of MTPCP was determined to be 0.5 mg/ml by the BCA protein assay kit.
Biological functions of the magnetic targeting pro-coagulant protein
The pro-coagulant activity of the MTPCP was estimated by the Spectrozyme FXa Assay as shown in . The half-maximal of tTF-EG3287@OCMC/Fe3O4 was observed at the concentration of 5 μmol/L, which was comparable to that of tTF and tTF-EG3287 protein. The results indicated that the nano-drug delivery system did not affect the pro-coagulant activity of the pro-coagulant protein.
Figure 5. Biological functions of the magnetic targeting pro-coagulant protein. (A) Spectrozyme FXa Assay of the tTF, tTF-EG3287 and tTF-EG3287@OCMC/Fe3O4. PBS and OCMC/Fe3O4 were used as negative control. Data represent the means ± SE of five independent experiments. Confocal microscopy (B) and flow cytometry (C) were used to evaluate the NRP-1 binding ability of the magnetic procoagulant protein on HUVEC cell. (D) Cytotoxicity on HepG2 and HUVEC cells after treated with tTF-EG3287@OCMC/Fe3O4 for 24 h. The values presented are the means ± SE of three independent experiments.
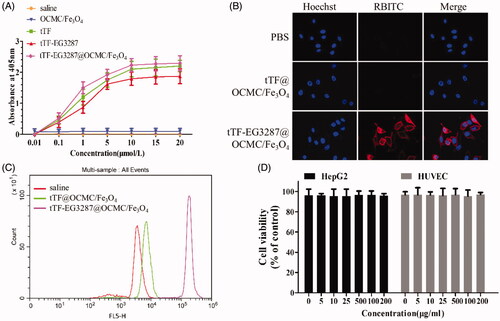
The NRP-1 binding ability of the magnetic targeting pro-coagulant protein was evaluated by confocal microscopy and flow cytometry as shown in . Strong red fluorescence signal of RBITC was found on the surface of cells treated by the magnetic targeting pro-coagulant protein tTF-EG3287@OCMC/Fe3O4. However, red fluorescence signal was not found in the PBS group and the tTF@OCMC/Fe3O4 group (without targeting peptide). Correspondingly, the flow cytometry shown that the exact fluorescence intensity of the tTF-EG3287@OCMC/Fe3O4 group was over 30-fold higher than the tTF/OCMC/Fe3O4 group which without the targeting peptide EG3287.
The cytotoxicity of the magnetic targeting pro-coagulant protein was determined by CCK-8 assay as shown in . The values presented are the means ± SE of three independent experiments. The tTF-EG3287@OCMC/Fe3O4 was shown to have no apparent cytotoxic effect on the HUVEC and HepG2 cells after 24 h co-culture. The cell viability of two cells at different concentrations were all over 95% of control, indicating that the MTPCP tTF-EG3287@OCMC/Fe3O4 did not cause a significant cell death and has low cytotoxicity. Besides, the MTPCP did not present concentration-dependent toxicity.
Magnetic targeting ability of the magnetic targeting pro-coagulant protein
The magnetic targeting ability of the magnetic targeting pro-coagulant protein was seen at various time interims in HepG2 tumour-bearing BALB/c nude mice. We set the fluorescence signal of saline group as background to remove the natural fluorescence of the mice. The protein concentration of Cy5.5-tTF-EG3287 and Cy5.5-tTF-EG3287@OCMC/Fe3O4 was 0.5 mg/mL, and the F/P value of the Cy5.5-tTF-EG3287 and Cy5.5-tTF-EG3287@OCMC/Fe3O4 were 1.82 and 1.75, respectively. Which means the amount of Cy5.5 bound to the protein of Cy5.5-tTF-EG3287 and Cy5.5-tTF-EG3287@OCMC/Fe3O4 were roughly the same.
As shown in ), in the Cy5.5-tTF-EG3287@OCMC/Fe3O4 group, the fluorescence signal of Cy5.5 was clearly detected on tumour area and hepatic zones of mice at 30 min after administration, and fluorescence signal on tumour area reached its maximum at 2 h and stayed more constant up to 12 h. While, no significant fluorescence was detected on tumour area of mice in the OCMC/Fe3O4 group, which indicated that the fusion protein tTF-EG3287 can be delivered to the tumour vessels by the drug delivery system (OCMC/Fe3O4). However, in the Cy5.5-tTF-EG3287 group, the fluorescence signal was detected in tumour area at 4 h after administration, which was much weaker than the tTF-EG3287@OCMC/Fe3O4 group. This can be explained by the NRP-1 targeting ability of the fusion protein itself. In addition, the fluorescence on the liver was stronger than that of the Cy5.5-tTF-EG3287@OCMC/Fe3O4 group within 4 h after administration.
Figure 6. Magnetic targeting ability of the magnetic targeting pro-coagulant protein detected by in vivo imaging system. (A) Delegate in vivo fluorescence images at various times after tail vein infusion with saline(a), OCMC/Fe3O4(b) and Cy5.5-tTF-EG3287(c) and Cy5.5-tTF-EG3287@OCMC/Fe3O4(d). As the reference scale on the right, different colours indicates different fluorescence intensities. Blue indicates strong fluorescence signal and red colour indicates a weak signal. (B) Cy5.5 fluorescence intensity in tumour tissues collected at 72 h following injection. Results were presented as means ± SE for a group of 3 mice.
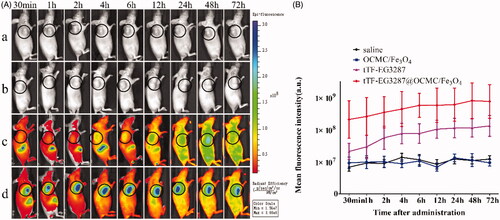
Regions of interest (ROI) were drawn over the tumour area of photon emission and quantified using the Living Image software, as shown in . The mean fluorescence value of tumour tissue in the Cy5.5-tTF-EG3287@OCMC/Fe3O4 group was higher than control groups.
The living imaging was indicated that OCMC/Fe3O4 is a suitable delivery carrier for the fusion protein tTF-EG3287, which further indicated that the MTPCP contains magnetic targeting ability in vivo.
Antitumor activity of the magnetic targeting pro-coagulant protein in subcutaneous transplantation tumour models
HepG2 subcutaneous transplantation tumour bearing BALB/c nude mice were used to assess the antitumor activity of the MTPCP. The tumour volume change after treatment of each group was shown in , a statistically significant reduction in tumour growth rate was observed on the fourth day after the first treatment injection. The pooled results of two independent experiments are presented in . In addition, as shown in , the body weight of mice of each group did not show significant differences (p = .124).
Figure 7. Effect of the magnetic procoagulant protein on growth of tumour in mice models. (A) Tumour volume of HepG2 tumour-bearing mice of each groups. Data are presented as means ± SE of two independent experiments. Statistical comparisons were done for each time point. Asterisks, statistical significance between tTF-EG3287@OCMC/Fe3O4 and controls (saline, OCMC/Fe3O4 and tTF-EG3287). Arrows, time points of injection. (B) Average body weight of mice in each group, data represent the means ± SE of two independent experiments. (C) Average tumour weights of HepG2 tumour-bearing mice of each groups after treatment, data are presented as means ± SE of two independent experiments. (D) Representative pictures of the HepG2 tumour bearing mice at the end of treatment (Day 7) by saline (a), OCMC/Fe3O4 (b), tTF-EG3287 (c) and tTF-EG3287@OCMC/Fe3O4 (d).
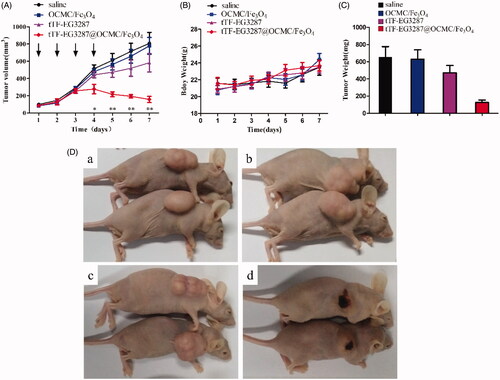
Table 2. Antitumor activity of the magnetic procoagulant protein in mice.
After seven days of treatment, mice were euthanized, and the subcutaneous tumour were removed and weighed. As shown in , the reduction in tumour volume correlated with a decrease in average tumour weight, the weight of tumour in the treatment group was significantly lower than the control groups (p < .001). However, there was no significant difference in both tumour volume and tumour weight between these three control groups (p = .261). The representative picture of the tumours after treated in each group was shown in . The difference in size and appearance of the MTPCP-treated tumours showed obvious signs of haemorrhage and necrosis in contrast to the vital appearance of control tumours.
Treatment effect of the magnetic targeting pro-coagulant protein in orthotopic transplantation models
In this study, we successfully constructed orthotopic transplantation hepatic carcinoma models for assessed the treatment effect of the magnetic targeting pro-coagulant protein. As shown in Supplementary Figure S2, the red frame represents the domain of cancer tissue. After treated by the MTPCP for 4 consecutive days, obvious thrombus was found in the blood vessels of hepatic carcinoma, as shown in ), the arrow represents the thrombus. Moreover, necrotic area was observed around the embolised blood vessels, as shown in , while thrombosis and cell necrosis were negative in the control groups (saline, OCMC/Fe3O4 and tTF-EG3287) as shown in .
Figure 8. Histology studies of the treatment effect of the magnetic procoagulant protein in subcutaneous transplantation tumour models. (A ∼ C) Representative picture of the cancer tissue after treatment of controls groups (saline (A), OCMC/Fe3O4 (B) and tTF-EG3287 (C)) stained with haematoxylin and eosin. Obvious thrombus (D ∼ F) and necrotic area (G ∼ I) were found in the blood vessels of hepatic carcinoma after treated by the MTPCP tTF-EG3287@OCMC/Fe3O4 for 4 consecutive days.
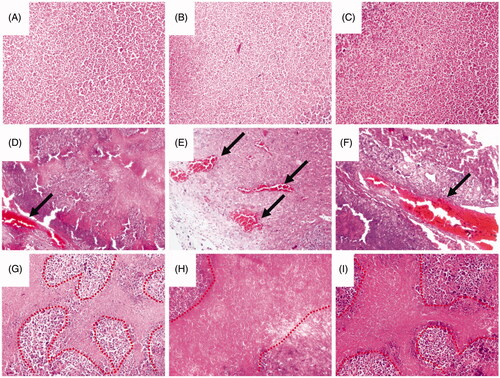
Safety and tolerability
During the study period, no mortality or morbidity was observed in any group of tumour-bearing mice, and differences in body weight was not observed between groups (. Which indicates that the MTPCP with a concentration of 0.5 mg/mL and at a dosage of 100 μL, about 25 mg/kg body weight for each injection, was not obviously toxic to the animals. In addition, histological analysis also showed no visible thrombosis in the major organs (heart, liver, spleen, lung, kidney and brain) of the tumour-bearing mice after four times of administrations (), indicating that the MTPCP is well tolerated.
Figure 9. Thrombotic risk assessment in the normal organs of the treated mice. Histological analysis of various normal organs of tumour-bearing mice treated with the MTPCP. Sections of heart (A), liver (B), spleen (C), lung (D), kidney (E) and brain (F) were stained with haematoxylin and eosin (H&E).
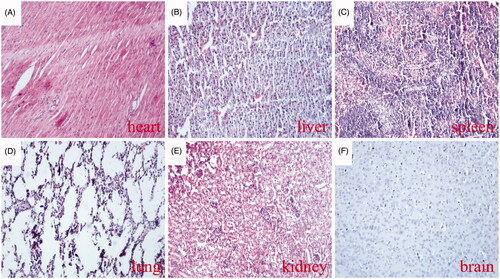
Discussion
Over the past years, many efforts have been undertaken to apply vascular disrupting agents as an additional way of cancer therapy. The concept that breaking blood supply of the tumour to inhibit tumour growth is remarkably potential due to the near universality of its application and the high efficiency of its treatment. It is well known that basically all solid tumours depend on a blood supply for growth and metastasis of cancer cells, which mean tumour embolic therapy can be applied to a variety of cancers. Besides, cytotoxic anticancer drugs like chemotherapeutic drugs have to reach every cancer cell directly to obtain the maximum therapeutic effect. But in practice, this is very difficult to achieve. It is possible to contact up to 109 of cells even in very small tumour deposits of 1 cm3. However, every vessel supplies the nutrition for, and removes waste products from, many thousands of tumour cells. Therefore, blockage of one tumour-supplying vessel might then efficiently lead to thousands of downstream cancer cells death [Citation38,Citation39,Citation40,Citation41].
Given the advantages of embolic therapy of tumour, we have designed and constructed a magnetic targeting pro-coagulant protein (MTPCP) for embolic therapy of tumour. The MTPCP is designed to contain three main biological characteristics – pro-coagulant activity, NRP-1 targeting ability and low toxicity, as shown in . The magnetic targeting ability is understood as drugs could be guided and enrich in the target organ by an external magnetic field [Citation42,Citation43]. As shown in , a high-intensity fluorescent signal was detected only in the tumour region of mice in the tTF-EG3287@OCMC/Fe3O4 group. Although the fusion protein tTF-EG3287 has NRP-1 targeting ability itself, the fluorescence intensity of the tumour area is significantly weaker than the tTF-EG3287@OCMC/Fe3O4 group. Which indicated that the nano-iron oxide delivery system facilitates drug (the fusion protein) enrichment in targeted areas. HepG2 tumour bearing nude mice models were used to evaluate the inhibition of tumour growth. As shown in , the MTPCP resulted in significant tumour growth retardation in tumour size and tumour weight in comparison with controls. After tumour-supplying vessels were blocked, the volume of the tumour increases at a significantly slower pace, and even the volume becomes smaller than that before therapy because of tumour tissue ischaemia ().
In clinical practice, the transarterial embolisation (TAE) and the transarterial chemoembolization (TACE) are widely used locoregional therapy in the treatment of hepatocellular carcinoma (HCC), especially for unresectable intermediate and advanced HCC [Citation44,Citation45]. While, TAE/TACE is still facing many problems as summarised follow: Firstly, the principle of TAE/TACE is based on the concept that liver tumours derive over 90% of their blood from the hepatic artery. So, arterial embolisation preferentially interrupts the tumour’s blood supply and inhibits growth [Citation46,Citation47]. However, even if the hepatic artery is completely blocked, liver tumours still have about 10% of their blood supplied by the portal vein. Moreover, TAE/TACE therapy may damage normal liver tissue that is supplied by the hepatic artery [Citation48]. Secondly, embolic agents used for TAE/TACE do not have a tumour blood vessel targeting ability. Off-target delivery of embolic agents such as reflux into healthy surrounding tissue is a potential side effect that may cause complications like ulceration of the gut or cholecystitis, or even lethal side effects also [Citation49]. Thirdly, the complexity of procedure is also a limitation. Anti-cancer drugs, embolisation particles, or drug eluting beads delivered to the cancerous mass via interventional surgery. The procedure is normally carried out by specialised techniques and devices with appropriate interventional occlusion training in a hospital setting [Citation50].
The embolic agent designed in this study might solve the above three main shortcomings of TAE/TACE. Our MTPCP, tTF-EG3287@OCMC/Fe3O4, it is not an embolic material itself, but it can block the blood supply with a specific mechanism which targets the NRP-1 expressed on tumour vascular endothelial cells, and then inducing thrombosis in the tumour blood vessels. Which means the occluded tumour blood vessel is exactly the site of pro-coagulant protein bound, as shown in . Theoretically, using our magnetic targeting pro-coagulant protein instead of the traditional embolic agents of TACE/TAE may significantly reduce the risk of ectopic embolisation. More importantly, as mentioned above, in clinical practice, tumour embolic therapy is mainly used for hepatocellular carcinoma due to the special dual blood supply system of the liver and HCC, which allows the administration of chemotherapeutic and embolisation agents selectively into the artery supplying the tumour [Citation51]. However, our MTPCP can be applied to almost all solid tumours due to its NRP-1 targeting ability, and NRP-1 high expression has been shown to be elevated in a number of human patient tumour samples and tumour vessels. In our study, we constructed orthotopic liver transplantation models in mice to assess the treatment effect of the MTPCP. We first compared the differences of NRP-1 expression between normal and tumorous tissues by immunohistochemistry, as shown in Supplementary Figure S3. The expression of NRP-1 in liver cancer tissues is significantly higher than that in normal liver tissues. As shown in , obvious thrombus was found in the blood vessels of hepatic carcinoma, and clear necrotic area was observed around the embolised blood vessels. In addition, the MTPCP is well tolerated. During our study period, no any indication of hypersensitivity or immunogenicity were observed. The MTPCP used in this study contains tTF-EG3287 and OCMC/Fe3O4 two components. In theory, OCMC/Fe3O4 does not have the risk of hypersensitivity or immunogenicity. And tTF or its recombination form have been reported by several studies [Citation7–11], all of those studies indicated that tTF is well tolerated.
In summary, we have successfully prepared the magnetic targeting pro-coagulant protein, tTF-EG3287@OCMC/Fe3O4. This is the first report to describe the construction of a magnetic targeting pro-coagulant protein for embolic therapy of solid tumours. We have shown that the MTPCP can be attracted on the tumour area by magnetic field and induce extensive thrombosis in tumour vessels, thus leading to effective antitumor therapy in both subcutaneous transplantation and orthotopic transplantation tumour models in mice. Moreover, the MTPCP displays properties quite different from those of conventional embolic substances. Various applications in endovascular therapy thus appear likely. The safety and efficacy of endovascular procedures for tumours are expected to improve as experience with the MTPCP is accumulated.
Ethics approval and consent to participate
All procedures were carried out in accordance with the National Institutes of Health Guide for the Care and Use of Laboratory Animals and were approved by the Animal Ethical and Welfare Committee of Xiamen University.
Abbreviations | ||
MTPCP | = | Magnetic targeting pro-coagulant protein |
tTF | = | Truncated tissue factor |
OCMC | = | O-Carboxymethyl chitosan |
NRP-1 | = | Neuropilin-1 |
TACE | = | Transarterial chemoembolization |
TAE | = | Transarterial embolisation |
Supplementary_Figures.docx
Download MS Word (380.4 KB)Disclosure statement
The authors report no conflicts of interest in this work. Guoqiu Wu, Fanghong Luo, Ting Wu and Jianghua Yan share co-corresponding author.
Additional information
Funding
References
- Vaidya S, Tozer KR, Chen J. An overview of embolic agents[J]. Semin Intervent Radiol. 2008;25(03):204–215.
- Marelli L, Stigliano R, Triantos C, et al. Transarterial therapy for hepatocellular carcinoma: which technique is more effective? A systematic review of cohort and randomized studies[J]. Cardiovasc Intervent Radiol. 2007;30(1):6–25.
- Li S, Jiang Q, Liu S, et al. A DNA nanorobot functions as a cancer therapeutic in response to a molecular trigger in vivo[J]. Nat Biotechnol. 2018; 36(3):258–264.
- Muller YA, Ultsch MH, de Vos AM. The crystal structure of the extracellular domain of human tissue factor refined to 1.7 angstrom resolution[J]. J Mol Biol. 1996;256(1):144–159.
- Drozdowska J. [Tissue factor in endothelial cells-its structure and function according to the current literature]. Postepy Biochem. 2012;58(3):273–280.
- Huang X, Molema G, King S. Tumor infarction in mice by antibody-directed targeting of tissue factor to tumor vasculature[J]. Science. 1997;275(5299):547–550.
- Ruf W, Rehemtulla A, Morrissey JH, et al. Phospholipid-independent and -dependent interactions required for tissue factor receptor and cofactor function. J Biol Chem. 1991;266(24):16256–16266.
- Hu P, Yan J, Sharifi J, et al. Comparison of three different targeted Tissue Factor fusion proteins for inducing tumor vessel thrombosis[J]. Cancer Res. 2003;63(16):5046–5053.
- Kessler T, Bieker R, Padró T, et al. Inhibition of tumor growth by RGD Peptide-Directed delivery of truncated tissue factor to the tumor vasculature[J]. Clin Cancer Res. 2005;11(17):6317–6324.
- Persigehl T, Ring J, Bremer C, et al. Non-invasive monitoring of tumor-vessel infarction by retargeted truncated tissue factor tTF–NGR using multi-modal imaging[J]. Angiogenesis. 2014;17(1):235–246.
- Shi Q, Zhang Y, Liu S, et al. Specific tissue factor delivery using a tumor-homing peptide for inducing tumor infarction[J]. Biochem Pharmacol. 2018;156:501–510.
- Zou M, Samiullah M, Xu P, et al. Construction of novel procoagulant protein targeting Neuropilin-1 on tumor vasculature for tumor embolization therapy[J]. J Drug Target. 2019;27(8):885–895.
- Kurschat P, Bielenberg D, Rossignol-Tallandier M, et al. Neuron restrictive silencer factor NRSF/REST is a transcriptional repressor of neuropilin-1 and diminishes the ability of semaphorin 3A to inhibit keratinocyte migration[J]. J Biol Chem. 2006;281(5):2721–2729.
- Latil A, Bièche I, Pesche S, et al. VEGF overexpression in clinically localized prostate tumors and neuropilin-1 overexpression in metastatic forms[J]. Int J Cancer. 2000;89(2):167–171.
- Bachelder RE, Crago A, Chung J, et al. Vascular endothelial growth factor is an autocrine survival factor for neuropilin-expressing breast carcinoma cells[J]. Cancer Res. 2001;61(15):5736–5740.
- Fakhari M, Pullirsch D, Abraham D, et al. Selective upregulation of vascular endothelial growth factor receptors neuropilin-1 and -2 in human neuroblastoma.[J]. Cancer. 2002;94(1):258–263.
- Bwc T, Volpert M, Ratther E, et al. Neuropilin-1 is upregulated in the adaptive response of prostate tumors to androgen-targeted therapies and is prognostic of metastatic progression and patient mortality[J]. Oncogene. 2017;36(24):3417–3427.
- Jia H, Bagherzadeh A, Hartzoulakis B, et al. Characterization of a bicyclic peptide neuropilin-1 (NP-1) antagonist (EG3287) reveals importance of vascular endothelial growth factor exon 8 for NP-1 binding and role of NP-1 in KDR signaling.[J]. J Biol Chem. 2006;281(19):13493–13502.
- Shi Z, Neoh KG, Kang ET, et al. (Carboxymethyl)chitosan-Modified superparamagnetic iron oxide nanoparticles for magnetic resonance imaging of stem cells[J]. ACS Appl Mater Interfaces. 2009;1(2):328–335.
- Bhattacharya D, Das M, Mishra D, et al. Folatereceptor targeted, carboxymethyl chitosan functionalized iron oxide nanoparticles: a novel ultradispersed nanoconjugates for bimodal imaging[J]. Nanoscale. 2011;3(4):1653–1662.
- Dusza A, Wojtyniak M, Nedelko N, et al. Magnetic behavior of O-Carboxymethylchitosan bounded with iron oxide particles[J]. IEEE Trans Magn. 2010;46(2):459–462.
- Arias LS, Pessan JP, Vieira APM, et al. Iron oxide nanoparticles for biomedical applications: a perspective on synthesis, drugs, antimicrobial activity, and toxicity[J]. Antibiotics. 2018;7(2):46–78.
- Xu J, Du Y, Liu XJ, et al. Recombinant EGFR/MMP-2 bi-targeted fusion protein markedly binding to non-small-cell lung carcinoma and exerting potent therapeutic efficacy[J]. Pharmacol Res. 2017;126:66–76.
- Xu J, Liu XJ, Li L, et al. An engineered TIMP2-based and enediyne-integrated fusion protein for targeting MMP-14 shows potent antitumor efficacy[J]. Oncotarget. 2015;6(28):26322–26334.
- Meng H, Zhang Z, Zhao F, et al. Orthogonal optimization design for preparation of Fe3O4 nanoparticles via chemical coprecipitation[J]. Appl Surf Sci. 2013;280(8):679–685.
- Mikhaylova M, Kim DK, Berry CC, et al. BSA immobilization on Amine-Functionalized superparamagnetic iron oxide nanoparticles[J]. Chem Mater. 2004;16(12):2344–2354.
- Zhang C, Jugold M, Woenne EC, et al. Specific targeting of tumor angiogenesis by RGD-conjugated ultrasmall superparamagnetic iron oxide particles using a clinical 1.5-T magnetic resonance scanner.[J]. Cancer Res. 2007;67(4):1555–1562.
- Miao HQ, Soker S, Feiner L, et al. Neuropilin-1 mediates Collapsin-1/Semaphorin III inhibition of endothelial cell motility: functional competition of Collapsin-1 and vascular endothelial growth factor-165[J]. J Cell Biol. 1999;146(1):233–242.
- Pan Q, Chathery Y, Wu Y, et al. Neuropilin-1 binds to VEGF121 and regulates endothelial cell migration and sprouting[J]. J Biol Chem. 2007;282(33):24049–24056.
- Thomas N, Bechet D, Becuwe P, et al. Peptide-conjugated chlorin-type photosensitizer binds neuropilin-1 in vitro and in vivo[J]. J Photochem Photobiol B. 2009;96(2):101–108.
- Kuo CF, Tsao N, Chou HH, et al. Release of FITC-BSA from poly(l-lactic acid) microspheres analysis using flow cytometry.[J]. Colloids Surf B Biointerfaces. 2012;89(1):271–276.
- Hische EA, Tutuarima JA, van der Helm HJ. Spectrophotometry of tissue thromboplastin in cerebrospinal fluid[J]. Clin Chem. 1981;27(8):1427–1430.
- Sun FX, Tang ZY, Liu KD, et al. Establishment of a metastatic model of human hepatocellular carcinoma in nude mice via orthotopic implantation of histologically intact tissues[J]. Int J Cancer. 1996;66(2):239–243.
- Zhao GJ, Xu LX, Chu ES, et al. Establishment of an orthotopic transplantation tumor model of hepatocellular carcinoma in mice.[J]. World J Gastroenterol. 2012;18(47):7087–7092.
- Schmitz V, Tirado-Ledo L, Tiemann K, et al. Establishment of an orthotopic tumour model for hepatocellular carcinoma and non-invasive in vivo tumour imaging by high resolution ultrasound in mice[J]. J Hepatol. 2004;40(5):787–791.
- Sitthichai S, Pilapong C, Thongtem T, et al. CMC-coated Fe 3 O 4 nanoparticles as new MRI probes for hepatocellular carcinoma[J]. Appl Surf Sci. 2015;356:972–977.
- Hong RY, Feng B, Chen LL, et al. Synthesis, characterization and MRI application of dextran-coated Fe3O4 magnetic nanoparticles[J]. Biochem Eng J. 2008;42(3):290–300.
- Burrows FJ, Thorpe PE. Vascular targeting-a new approach to the therapy of solid tumors]. Pharmacol Ther. 1994;64(1):155–174.
- Tie J, Desai J.Antiangiogenic therapies targeting the vascular endothelia growth factor signaling system. Crit Rev Oncog. 2012;17(1):51–67. 22471664 doi:10.1615/critrevoncog.v17.i1.50.
- Wick W, Platten M, Wick A, et al. Editor's choice: Current status and future directions of anti-angiogenic therapy for gliomas[J]. Neuro Oncol. 2016;18(3):315–328.
- Jayson G C, Kerbel R, Ellis L M, et al. Antiangiogenic therapy in oncology: current status and future directions. The Lancet. 2016;388(10043):518–529. doi:10.1016/S0140-6736(15)01088-0.
- Lübbe AS, Alexiou C, Bergemann C. Clinical applications of magnetic drug targeting.[J]. J Surg Res. 2001;95(2):200–206.
- Krukemeyer MG, Wagner W, Jakobs M, et al. Tumor regression by means of magnetic drug targeting[J]. Nanomedicine. 2009;4(8):875–882.
- Cammà C, Schepis F, Orlando A, et al. Transarterial chemoembolization for unresectable hepatocellular carcinoma: Meta-Analysis of randomized controlled trials1[J]. Radiology. 2002;224(1):47–54.
- Wu CC, Ho YZ, Ho WL, et al. Preoperative transcatheter arterial chemoembolization for resectable large hepatocellular carcinoma: a reappraisal.[J]. Br J Surg. 1995;82(1):122–126.
- Miraglia R, Pietrosi G, Maruzzelli L, et al. Efficacy of transcatheter embolization/chemoembolization (TAE/TACE) for the treatment of single hepatocellular carcinoma[J]. World J Gastroenterol. 2007;13(21):2952–2955.
- Park YN, Yang CP, Fernandez GJ, et al. Neoangiogenesis and sinusoidal “in dysplastic nodules of the liver”. Am J Surg Pathol. 1998;22(6):656–662.
- Stuart K. Chemoembolization in the management of liver tumors[J]. Oncologist. 2003;10(02):425–437.
- Osuga K, Maeda N, Higashihara H, et al. Current status of embolic agents for liver tumor embolization[J]. Int J Clin Oncol. 2012;17(4):306–315.
- Tam KY, Leung CF, Wang YXJ. Chemoembolization agents for cancer treatment[J]. Eur J Pharm Sci. 2011;44(1–2):1–10.
- Guan YS, Hu Y, Liu Y. Multidetector-row computed tomography in the management of hepatocellular carcinoma with transcatheter arterial chemoembolization.[J]. J Gastroenterol Hepatol. 2006;21(6):941–946.