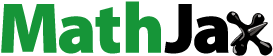
Abstract
Overexpression of CD30 has been reported on the surface of some T-cell lymphomas, especially on Hodgkin’s lymphoma (HL) and anaplastic large cell lymphoma (ALCL). CD30 targeted immunotherapy has good clinical therapy response. We have produced a novel antibody drug conjugates (ADCs)-anti-CD30-LDM, which shows attractive tumour-targeting capability and extremely potent antitumor efficacy. To further investigate biological characteristics and promote clinical translation of anti-CD30-LDM, we constructed a radiolabeled 123I-anti-CD30-LDM to evaluate the biodistribution characteristics. The anti-CD30-LDM was radioiodinated by the Iodogen method. The radiochemical purity of 123I-anti-CD30-LDM was more over 98%, and the specific activity of 240.5 MBq/mg. The stability and the specificity of 123I-anti-CD30-LDM were evaluated in vitro. Cellular binding assays were used to evaluate the binding capabilities in CD30-positive Karpas299 cells and CD30-negative Raji cells. B-NDG mice bearing Karpas 299 and Raji xenografts were used for in vivo biodistribution studies. Our results demonstrated that anti-CD30-LDM as an ideal ADC targeted to CD30, which was labelled easily with 123I and obtained the sufficient yields. The 123I-anti-CD30-LDM preserved specific binding to CD30 in vitro and uptake in tumour xenografts in B-NDG mice. These results are encouraging for anti-CD30-LDM as a promising clinical translational candidate for various CD30 positive lymphomas and other diseases.
Introduction
CD30 is a 120 kDa type I transmembrane glycoprotein belonging to the tumour necrosis factor receptor superfamily [Citation1]. Overexpression of CD30 has been reported in Hodgkin’s lymphoma (HL) and anaplastic large-cell lymphoma (ALCL). Recently, CD30-positive expression was also reported in other diseases [Citation2,Citation3]. CD30-targeted immunotherapy has been used in the recurrent, or refractory HL/ALCL [Citation4,Citation5]. The advent of antibody–drug conjugates (ADCs) has the potential to revolutionize the treatment of lymphoma [Citation6]. ADCs are designed to bind to the precise targets on tumour cells and deliver cytotoxic agents after being internalized, thus, sparing normal cells and limiting toxicities. One of the most successful ADCs is Brentuximab vedotin (BV), an anti-CD30 ADCs the microtubule-disrupting agent monomethyl auristatin E (MMAE) by a protease-cleavable linker [Citation7]. BV is a successful treatment for patients with certain types of lymphoma [Citation8]. However, many patients do not achieve complete remission and eventually become resistant to BV treatment. Furthermore, MMAE resistance has been associated with resistance to BV [Citation9]. Therefore, it is imperative to develop other cytotoxic agents that can be linked to ADCs.
Lidamycin (LDM) is a novel enediyne-containing antitumor antibiotic developed by our laboratory. LDM inhibits DNA synthesis and causes cellular DNA breakage in cancer cells [Citation10]. It contains an active enediyne chromophore (AE) responsible for the extremely potent bioactivity and a noncovalently bound apoprotein LDP, which forms a hydrophobic pocket to protect the chromophore [Citation11]. AE and LDP can be dissociated and reconstituted under certain conditions in vitro [Citation12].
We have previously described a novel ADC, anti-CD30-LDM [Citation13], which is comprised of two molecules of LDM linked to an anti-CD30 monoclonal antibody (mAb) via noncleavable linkers. Thus, the LDM is delivered to the CD30-positive cancer cells by the mAb, causes DNA damage by inducing double-strand breaks, and results in cell cycle arrest and apoptosis [Citation14, Citation15]. This ADC displays specific affinity and extremely potent cytotoxicity against CD30-overexpressing tumour cells in vitro, with IC50 (half-maximal inhibitory concentration) values ranging from 0.54 × 10−11 to 3.74 × 10−11 M. In a Karpas299 xenograft model, tumour growth was inhibited by 87.76% in mice treated with 0.7 mg/kg anti-CD30-LDM [13]. The combination of anti-CD30-LDM with crizotinib exhibits a synergistic inhibitory antitumor effect in ALCL [Citation16]. Based on the excellent characteristics of anti-CD30-LDM – high binding capability and highly specific to CD30 antigen, extremely potent cytotoxicity against CD30-overexpressing tumour cells, we further investigated the biodistribution of anti-CD30-LDM in the CD30-positive lymphoma models. Here, the labelling method and condition, physiochemical property, in vitro activity, in vivo biodistribution of anti-CD30-LDM were studied. The preliminary biodistribution data would provide the basis for further clinical translational research of anti-CD30-LDM.
Materials and methods
Materials
Iodogen, Sigma-Aldrich Co. (St. Louis, MO); phosphate-buffered saline, pH7.4,10 mM, Shanghai Angyi Biotech Co. Ltd. (Shanghai, China); 0.9% NaCl Injection, Shijiazhuang Siyao Co. LTD., Hebei, China; n-octanol(AR), Sinopharm Chemical Reagent Co., Ltd., Shanghai, China; Sephadex PD10 Column, GE Healthcare UK Limited, Cambridge, UK; Na123I solution, High Tech of Atom Co. Ltd., Beijing, China.
Equipment
1260 HPLC, Agilent Technologies (Santa Clara, CA); GABI HPLC Radioactive Detector, Germany Elysia-Raytest GmbH, Straubenhardt, Germany; TSK gel G3000SWXL HPLC Column, 7.8 mm 30.0 cm, Japan TOSON Corporation, Tokyo, Japan; FT-603 γ-Counter, Beijing Nuclear Instrument Factory, Beijing, China;CRC15R Radioactivity Metre:US Capintec Inc.; Multi-purpose Thermostat, Tianjin autoscience Instrument Co. Ltd.; MILLIPORE Water Purification System, France Millipore SA (Millipore, Billerica, MA).
Preparation of the anti-CD30-LDM
Anti-CD30-LDM was prepared in our laboratory and stored at −80 °C. Briefly, the anti-CD30-LDP was cultured from growth medium of anti-CD30 antibody-expressing CHO cells and purified by protein G columns. The chromophore AE of LDM was separated by HPLC. Then, the AE-containing solution was mixed with the anti-CD30-LDP solution to form the enediyne-integrated ADC anti-CD30-LDM. Next, free AE was removed by ultrafiltration centrifugation. The composition of the ADC was finally confirmed by reverse-phase HPLC. For more detailed information, see literature [Citation13].
ELISA
The 96-well plates were coated with the different concentrations of recombinant CD30-Fc fusion protein (R&D Systems, Minneapolis, MN). After the wells were blocked with 2% BSA/PBST (phosphate-buffered saline with Tween), anti-CD30-LDM was incubated at varying concentrations at 37 °C for 1 h. Then, the wells were washed with PBST and incubated with an alkaline phosphatase goat anti-human IgG (Fab-specific) probe at 37 °C. After substrate was added, the reaction was stopped. The optical density at 405 nm was determined using a microplate reader (Thermo Fisher Scientific, Waltham, MA).
Flow cytometry analysis
For antibody saturation binding, 3 105 cells were incubated with increasing concentrations of anti-CD30-LDM for 1 h at 4 °C, then washed twice with ice-cold staining medium to remove free mAbs, and incubated with 1: 100 FITC-conjugated goat anti-human IgG for 1 h at 4 °C. The labelled cells were washed and resuspended in PBS, and then MFI was determined by FACS Calibur (BD Biosciences, San Jose, CA).
Preparation of the 123I-anti-CD30-LDM
Labelling
300 μL of pH 7.2 (0.05 M), 100 μL of antibody anti-CD30-LDM (10 mg/mL) and 100 μL of 1.11 GBq (30 mCi) Na123I (pH 7.0, High Tech of Atom Co. Ltd., Beijing, China) solution were added to the reaction tube containing 50 μg of Iodogen (Sigma-Aldrich, St. Louis, MO). The reaction mixture was incubated at 37 °C for 40 min, and diluted with 1 ml water. The radio labelling efficiency was analyzed by size exclusion HPLC (see HPLC Analysis Section).
Purification
The radiolabeled antibody was purified on Sephadex PD10 Column (GE Healthcare UK Limited, Little Chalfont, UK). In brief, the Sephadex PD10 Column was washed with saline for 3 times. The mixture was applied to the Column, and then the Column was washed with 10 ml of saline. Each tube received 0.5 ml of the eluent, and the activity of the eluent was measured with a radioactivity metre to draw the elution curve. The radiochemical purity after purification was analyzed by size exclusion HPLC (see HPLC Analysis Section). The specific activity of 123I-anti-CD30-LDM was calculated by the radioactivity after purification and quantification.
HPLC analysis
The radio labelling efficiency and radiochemical purity were analyzed by size exclusion HPLC on an Agilent 1260 system (Agilent Technologies, San Diego CA). HPLC systems were equipped with in-line ultraviolet (280 nm) and radiation detectors. A TSK gel G3000SWXL HPLC Column (7.8 mm30.0 cm, TOSON Corporation, Tokyo, Japan) was used at a flow rate of 2 ml/min with pH 7.4 10 mM PBS solution. The radioactivity of the eluate was monitored using an in-line NaI radiodetector (Elysia-Raytest GmbH, Straubenhardt, Germany).
Octanol-Water partition coefficient (log POctanol/water)
To determine the lipophilicity of 123I-anti-CD30-LDM, approximately 18 million counts per minute of the radiolabeled antibody were diluted in 0.5 ml PBS. An equal volume of 1-octanol was added to obtain a binary phase system. After stirred in a vortex mixer for 2 min, it was separated into 2 layers by centrifugation (3500 RPM, 5 min). 0.2 ml sample was taken from each layer, and radioactivity was measured in a well-type γ-Counter (Beijing Nuclear Instrument Factory). Log P Octanol/Water = log (Counts Octanol/Counts Water) (n = 3).
Stability
Approximately 37 MBq of 123I-anti-CD30-LDM (0.1 ml) was added to 0.5 ml of foetal bovine serum (FBS) and saline. The samples were incubated at 37 °C at approximately 3.5, 7, 15 and 24 h. An equal volume of acetonitrile was added and stirred in a vortex mixer, then followed by centrifugation at 3500 RPM for 5 min to pellet the precipitated serum proteins. The supernatant was analyzed on HPLC as described above (see HPLC Analysis Section).
Cell lines
The human anaplastic large cell lymphoma (ALCL) Karpas 299 cells were purchased from BIOPIKE (Beijing, China). The Burkitt lymphoma Raji cells were provided by our laboratory. Cells were grown in RPMI-1640 medium, supplemented with 10% FBS, and 100 units/mL of both penicillin and streptomycin. Cell lines were maintained in 5% CO2 at 37 °C.
Cellular binding assays
A serial dilutions of Karpas 299 and Raji cells were collected in the range of 10 × 104 to 500 × 104 cells in triplicate. Each tube was incubated with 123I-anti-CD30-LDM (∼14 KBq [∼0.4 μCi]; ∼50 ng of proteins) in PBS containing 1% bovine serum albumin at room temperature for 2 h on an orbital shaker. Cells were washed 2 times with PBS, collected and counted using a gamma counter. The percentage of specifically bound 123I activity was assessed by comparison with standards and controls.
Biodistribution in xenograft models
All animal experiments were conducted with approval from the Institutional Animal Care and Use Committee of the Institute of Medicinal Biotechnology, Chinese Academy of Medical Sciences, China. Female B-NDG mice (18–20 g, 6–8 weeks old) were obtained from Jiansu Biocytogen Co., Ltd (Nantong, China). Aliquots of cells (5 × 106) of Karpas 299 and Raji cells were suspended in 200 μL of PBS and subcutaneously injected into the right flank of mice. Mice were monitored for general health and tumour growth and size every other day. The tumours developed for use for in vivo studies after 10–14 d, and the volume of tumour nodule is up to 300-500 mm3 and ready for biodistribution studies.
Biodistribution studies were conducted to evaluate the uptake of 123I-anti-CD30-LDM in mice bearing s.c. Karpas299 or Raji tumours. Mice were randomized into 4 groups (n = 5) before the study and the tails of mice were warmed gently with a warm bath for 5 min before receiving 123I-anti-CD30-LDM (740 KBq in 100 μL of 0.9% sterile saline for injection) via intravenous (i.v.) tail-vein injection (t = 0 h). Animals (n = 5, per group) were euthanized by CO2 inhalation at 3.5 h, 7 h, 15 h and 24 h after injection, and tissues of interest (including the tumour) were removed, rinsed in water, dried in air for 5 min, weighed, and counted using a well-type gamma counter for accumulation of 123I radioactivity. Count data were decay-corrected, and the percentage injected activity per gram (%ID/g) for each tissue sample was calculated by normalization to the total amount of activity injected.
Statistical analysis
The data are expressed as the mean ± SD. The statistical significance between two groups was determined using two-way ANOVA, followed by Student’s t-test. For all tests, p values < .05 were considered statistically significant.
Results
Anti-CD30-LDM had high-affinity binding to CD30 antigen and CD30-positive cell lines
Even though the biodistribution and metabolism of ADCs (anti-CD30-LDM) can be inferred from studies with antibodies alone (anti-CD30), there is evidence that these characteristics of ADCs may be different than that of pure antibodies [Citation17]. We first detected the antigen-binding properties of anti-CD30-LDM. The binding of anti-CD30-LDM to recombinant human CD30 was assayed by ELISA. Anti-CD30-LDM showed a saturable dose–response curve as well as the anti-CD30-LDP (). It indicated that the anti-CD30-LDM after the conjugation of AE kept the similar specific antigen-binding activity of anti-CD30-LDP.
Figure 1. Anti-CD30-LDM retains the specific binding to CD30 antigen and CD30 positive cells in vitro. (A) Binding activity of anti-CD30-LDM and anti-CD30-LDP to recombinant human CD30 by ELISA. (B) Binding curves of increasing concentrations of anti-CD30-LDM and anti-CD30-LDP to Karpas 299 cancer cells by FACS analysis. The vertical axis represents the MFI values.
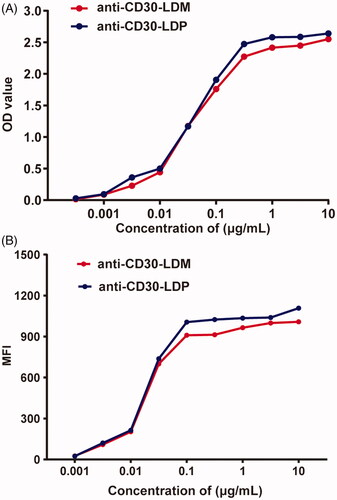
The binding affinity to CD30+ Karpas 299 cells was further investigated by FACS. Measurement of the binding of anti-CD30-LDM after incubation at different concentrations with identical numbers of cells revealed a saturable dose–response curve similar to the anti-CD30-LDP (). The results demonstrated that anti-CD30-LDM not only recognized CD30 antigen, but also had high-affinity and specific binding with native CD30 on the surface of lymphoma cells.
Radiolabeling and physical characterization of 123I- anti-CD30-LDM
Initial labelling efficiency of the 123I-anti-CD30-LDM at 37 °C for 40 min, 2 h and 3.5 h was 20%, 30% and 59%, respectively (100 μg anti-CD30-LDM, 4–5 mCi Na123I and 50 μg Iodogen). However, when 1 mg of anti CD30-LDM and 555 MBq were used, labelling efficiency was increased to 70% under the conditions of 37 °C for 40 min. HPLC chromatogram of labelling efficiency analysis was shown in . The elution curve of 123I-anti-CD30-LDM on PD10 Column eluting with saline is shown in . The 123I-anti-CD30-LDM was eluted firstly (tube 6th to 8th), then unlabelled 123I was eluted secondly (tube 11th to 17th). Finally, 123I-anti-CD30-LDM was successfully prepared by this method, and the radiochemical yield was 45% (without correcting for decay). After purification by Sephadex PD-10 columns, 123I-anti-CD30-LDM was formulated in sterile saline for injection with a radiochemical purity of more than 98%. The retention time of 123I-anti-CD30-LDM was consistent with the unlabelled anti-CD30-LDM () by HPLC analysis. The radioactivity concentration was 329 MBq/mL (8.9 mCi/mL). The specific activity was more than 240.5 MBq/mg (36.1 TBq/μmol) of protein. The total preparation time was 60 min.
Figure 2. The labelling efficiency of 123I-anti-CD30-LDM. (A) The labelling efficiency by HPLC (radiation detector) after purification of the 123I-anti-CD30-LDM. (B) The labelling efficiency by UV HPLC detector after purification of the anti-CD30-LDM.
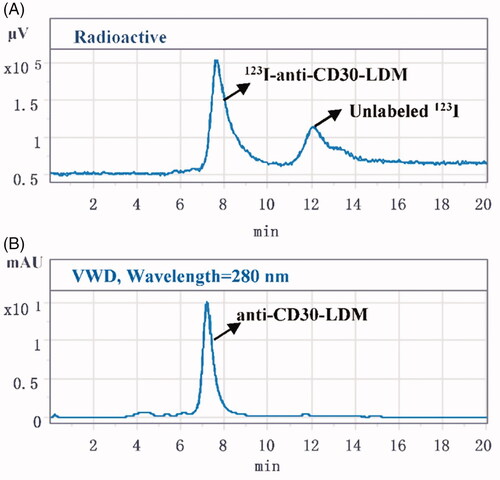
Figure 4. The radiochemical purity of 123I-anti-CD30-LDM after purification. (A) Analysis by HPLC (radiation detector) after purification of the 123I-anti-CD30-LDM. (B) Analysis by UV HPLC detector after purification of the anti-CD30-LDM.
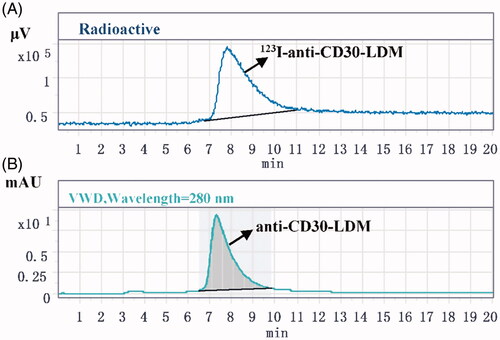
To determine the lipophilicity of the radiolabeled antibody of 123I-anti-CD30-LDM, the Octanol-water partition coefficient was determined. The Log P Octanol/Water value was -2.47 ± 0.03 (n = 3), indicating that 123I-anti-CD30-LDM has good water solubility.
Both stability of 123I-anti-CD30-LDM on saline and FBS were tested when it was incubated at 37 °C for 24 h. The radiochemical purity at 24 h was 97% in saline, and 94% in FBS () compared with the original. The stability test showed there was not a significant deiodination process of 123I-anti-CD30-LDM in vitro.
Cellular binding abilities of 123I-anti-CD30-LDM
To confirm that the conjugation and 123I-radiolabeling anti-CD30-LDM did not compromise antibody immunoreactivity, cellular binding assays were performed (). Briefly, different numbers of Karpas 299 and Raji cells were incubated with 123I-anti-CD30-LDM for 2 h. Cells were then counted using a gamma counter. The percentage of specifically bound 123I activity was calculated. The data revealed that as the numbers of CD30-positive Karpas299 cells increased (in the range of 10–500 104 cells/tube), the binding of 123I-anti-CD30-LDM also increased to more than 98% specifically binding activity. Control experiments using CD30-negative Raji cells showed no specific binding. Collectively, these data confirmed that 123I-anti-CD30-LDM retained high immunoreactivity and specificity for native CD30 antigen on tumour cells.
Biodistribution data
Ex vivo biodistribution studies were conducted at 3.5, 7, 15 and 72 h after administration of 123I-anti-CD30-LDM in separate groups of Karpas299 and Raji tumour-bearing mice. It was shown that the radiotracer displays a background distribution and tumour uptake ( and ). Tumour uptake in the CD30-positive Karpas299 model was 4.58 ± 1.06 percentage injected activity (%ID)/g at 24 h after administration. In contrast, accumulation of radioactivity in the Raji CD30-negative model was significantly lower, only 2.75 ± 0.47%ID/g at 24 after administration (p < .01 for comparisons between tumour models).
Figure 7. The biodistribution of 123I-anti-CD30-LDM in Karpas 299 and Raji tumour-bearing nude mice (data were shown as mean SD, %ID/g, n = 5).
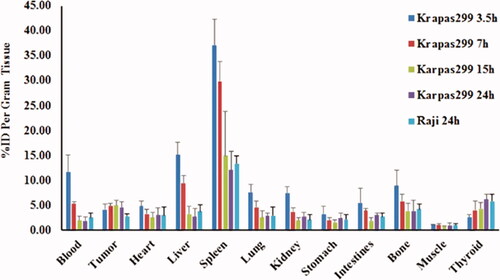
Table 1. Biodistribution of 123I-anti-CD30-LDM in mice bearing Karpas 299 and Raji tumours.
The highest accumulation of 123I radioactivity among normal organs was found in the spleen, with 37.07 ± 5.29% ID/g at 3.5 h after injection in Karpas299 model. At 24 h after radiotracer administration, and spleen uptake of 123I radioactivity was 12.05 ± 3.79% ID/g at 24 h in Karpas299 model, and 13.32 ± 1.56% ID/g in the CD30-negative Raji model, which was the same as in the CD30 positive model. Among other tissues, blood, heart, liver and bone showed higher accumulation of 123I-anti-CD30-LDM. The uptake of thyroid to 123I-anti-CD30-LDM was slightly increased from 3.5 to 24 h, which indicated in vivo deiodination of 123I-anti-CD30-LDM was appeared within acceptable range.
The tumour to muscle ratios and tumour to blood ratios were gradually increased. Maximum values of tumour uptake for 123I-anti-CD30-LDM in CD30 (+) karpas299 models showed 2-fold higher than in CD30 (-) Raji models (Tumour/Blood). The tumour/background ratios obtained after 24 h were the highest for 123I-anti-CD30-LDM in Karpas299 model ( and , p < .01 for comparisons between tumour models).
Discussion
On the basis of clinical use of brentuximab vedotin, anti-CD30 ADCs offer a promising alternative treatment option for refractory Hodgkin lymphomas and non-Hodgkin lymphomas [Citation18,Citation19]. Despite high response rates, many patients do not achieve complete remission and develop BV-resistant disease [Citation20–22]. MMAE resistance has been reported as one of the BV resistance mechanisms explored from patients [Citation9]. The novel type of ADC-anti-CD30-LDM, differed from the mechanism of MMAE. LDM does not block the polymerization of tubulin but exerts its cytotoxic effects through inducing DNA damage [Citation23]. Therefore, we speculated that anti-CD30-LDM might have the potential to overcome resistance induced by BV. We had reported anti-CD30-LDM either alone or combination with crizotinib show excellent antitumor activities in CD30+/ALK + anaplastic large cell lymphoma both in vitro and in vivo [Citation13,Citation16]. It indicated that anti-CD30-LDM could be a promising candidate for the treatment of CD30+ lymphomas.
To promote the clinical translational of anti-CD30-LDM, we would to further investigate the feasible use of isotope labelled with anti-CD30-LDM in the current study. By using the Iodogen method, 123I-anti-CD30-LDM was found to be stable with high immunoreactivity in vitro. 123I-anti-CD30-LDM was specifically taken up by CD30 (+) Karpas299 cells at a much higher binding ratio than that in CD30 (−) Raji cells. Moreover, in the biodistribution study of tumour xenografts, obvious radioactivity could be detected in tumour area of CD30 (+) Karpas299 tumour-bearing mice (4.98 ± 0.99%ID/g). However, for the radioactivity in tumour area of the CD30 (−) Raji tumour-bearing mice, the uptake is only 2.75 ± 0.47%ID/g. This biodistribution studies demonstrated that tumour uptake for 123I-anti-CD30-LDM in CD30 (+) models was 2-fold higher than in CD30 (−) models.
The antibodies used in this experiment can be labelled easily and sufficient yields obtained with 123I. We report here for the first time the biodistribution properties of 123I-anti-CD30-LDM in the B-NDG mice engrafted with CD30 positive Karpas 299 cells and CD30 negative Raji cells. Only two previous reports have described CD30 radioimmunotherapy (RIT) in B-NDG mice. An important consideration in the design of RICs is the residence time in blood.
The restricted expression of CD30 limits non-specific background uptake in organs such as the spleen suggests a promising role for CD30-targeted RIT [Citation24]. Therefore, some studies have attempted to target CD30 with RIT. The first feasibility study of treatment with 131I-Ki-4 was used for the cell toxic beta-emitter in patients with refractory, advanced-stage HL was completed in 2005 [Citation25]. In animal model, 211At radiolabeled HeFi-1 was very promising for the treatment of CD30-expressing leukemias and lymphomas, and the combination regimen of 211At-HeFi-1 with unmodified HeFi-1 enhanced the therapeutic efficacy [Citation26]. Another study with anti-CD30 RICs for treatment of HL was performed and published in 2010[27]. In this study, by comparison of the binding affinity to a CD30-positive HL cell and biodistribution in HL bearing mice of anti-CD30 RICs of varying structure and labelling nuclides, they found in vitro tumour cell affinities of all RICs were promising. However, in vivo biokinetics tested in the mouse model did not meet expectations. Therefore, as a sensible supplementary therapy for treatment of HL, the development of more effective new anti-CD30 conjugates is very important. In the context of CD30-imaging radiotracer, Rylova et al. reported the anti-CD30 (AC-10) labelled with 89Zr was a sensitive PET agent with high tumour-to-normal-tissue contrast in HL model [Citation24]. Kang et al. reported that 89Zr-radiolabeled Brentuximab vedotin (BV) was developed for in vivo tracking of BV and imaging CD30 expression in lung cancer models [Citation28].
The main problem with the radioactive anti-CD30 mAbs, was their high and persistent radioactivity concentration in blood. It is known that a soluble form of CD30 (sCD30) is elevated in serum of patients with HL by shedding [Citation29]. The extracellular, sCD30 produced by shedding is 90 kDa in size and can be bound either by anti-CD30 antibody or by the applied RICs [Citation30,Citation31]. This was the decisive obstacle for application of the anti-CD30 RICs selected [Citation27]. In our case, although blood uptake is higher up to 11.68 ± 3.40%ID/g at 3.5 h, but it graduated down to average 1.82 ± 0.88%ID/g in Karpas299 and 2.75 ± 0.47%ID/g in Raji at 24 h. Both the tumour/blood and the tumour/muscle ratio increase steadily over the course of time in karpas299 models. This will allow delivery of high doses of radiation precisely to the tumour while minimal doses to normal tissues.
In conclusion, anti-CD30-LDM can be labelled with 123I easily and sufficient yields were obtained by our methods. After successful labelling and measurement of tumour and organ uptake for 123I-anti-CD30-LDM, we conclude that 123I-anti-CD30-LDM preserved specific binding to CD30 in vitro and uptake in tumour xenografts in B-NDG mice. Our research provides a basis of anti-CD30-LDM as a promising candidate for further clinical translational study targeting to CD30 positive lymphomas and other diseases.
Data availability statement
The authors confirm that the data supporting the findings of this study are available within the article.
Disclosure statement
No potential conflict of interest was reported by the authors.
Additional information
Funding
References
- Vaklavas C, Forero-Torres A. Safety and efficacy of brentuximab vedotin in patients with Hodgkin lymphoma or systemic anaplastic large cell lymphoma. Ther Adv Hematol. 2012;3(4):209–225.
- Shinoda K, Sun X, Oyamada A, et al. CD30 ligand is a new therapeutic target for central nervous system autoimmunity. J Autoimmun. 2015;57:14–23.
- Blatt K, Cerny-Reiterer S, Schwaab J, et al. Identification of the Ki-1 antigen (CD30) as a novel therapeutic target in systemic mastocytosis. Blood. 2015;126(26):2832–2841.
- Muta H, Podack ER. CD30: from basic research to cancer therapy. Immunol Res. 2013;57(1–3):151–158.
- Hombach AA, Gorgens A, Chmielewski M, et al. Superior Therapeutic Index in lymphoma therapy: CD30(+) CD34(+) hematopoietic stem cells resist a chimeric antigen receptor T-cell attack. Mol Ther. 2016;24(8):1423–1434.
- Jagadeesh D, Smith MR. Antibody Drug Conjugates (ADCs): changing the treatment landscape of lymphoma. Curr Treat Options Oncol. 2016;17(10):55.
- Chen R, Gopal AK, Smith SE, et al. Five-year survival and durability results of brentuximab vedotin in patients with relapsed or refractory Hodgkin lymphoma. Blood. 2016;128(12):1562–1566.
- Horwitz S, O'Connor OA, Pro B, et al. Brentuximab vedotin with chemotherapy for CD30-positive peripheral T-cell lymphoma (ECHELON-2): a global, double-blind, randomised, phase 3 trial. Lancet. 2019;393(10168):229–240.
- Chen R, Hou J, Newman E, et al. CD30 downregulation, MMAE resistance, and MDR1 upregulation are all associated with resistance to brentuximab vedotin. Mol Cancer Ther. 2015;14(6):1376–1384.
- Shao RG, Zhen YS. Enediyne anticancer antibiotic lidamycin: chemistry, biology and pharmacology. ACAMC. 2008;8(2):123–131.
- Guo XF, Zhu XF, Shang Y, et al. A bispecific enediyne-energized fusion protein containing ligand-based and antibody-based oligopeptides against epidermal growth factor receptor and human epidermal growth factor receptor 2 shows potent antitumor activity. Clin Cancer Res. 2010;16(7):2085–2094.
- Tanaka T, Fukuda-Ishisaka S, Hirama M, et al. Solution structures of C-1027 apoprotein and its complex with the aromatized chromophore. J Mol Biol. 2001;309(1):267–283.
- Wang R, Li L, Zhang S, et al. A novel enediyne-integrated antibody-drug conjugate shows promising antitumor efficacy against CD30(+) lymphomas. Mol Oncol. 2018;12(3):339–355.
- Beerman TA, Gawron LS, Shen B, et al. The radiomimetic enediyne, 20'-deschloro-C-1027 induces inter-strand DNA crosslinks in hypoxic cells and overcomes cytotoxic radioresistance. DNA Repair (Amst). 2014;21:165–170.
- Beerman TA, Gawron LS, Shin S, et al. C-1027, a radiomimetic enediyne anticancer drug, preferentially targets hypoxic cells. Cancer Res. 2009;69(2):593–598.
- Wang R, Li L, Duan A, et al. Crizotinib enhances anti-CD30-LDM induced antitumor efficacy in NPM-ALK positive anaplastic large cell lymphoma. Cancer Lett. 2019;448:84–93.
- Han TH, Zhao B. Absorption, distribution, metabolism, and excretion considerations for the development of antibody-drug conjugates. Drug Metab Dispos. 2014;42(11):1914–1920.
- Prince HM, Kim YH, Horwitz SM, et al. Brentuximab vedotin or physician's choice in CD30-positive cutaneous T-cell lymphoma (ALCANZA): an international, open-label, randomised, phase 3, multicentre trial. Lancet. 2017;390(10094):555–566.
- O'Connor OA, Lue JK, Sawas A, et al. Brentuximab vedotin plus bendamustine in relapsed or refractory Hodgkin's lymphoma: an international, multicentre, single-arm, phase 1-2 trial. Lancet Oncol. 2018;19(2):257–266.
- Cheah CY, Chihara D, Horowitz S, et al. Patients with classical Hodgkin lymphoma experiencing disease progression after treatment with brentuximab vedotin have poor outcomes. Ann Oncol. 2016;27(7):1317–1323.
- Pro B, Advani R, Brice P, et al. Five-year results of brentuximab vedotin in patients with relapsed or refractory systemic anaplastic large cell lymphoma. Blood. 2017;130(25):2709–2717.
- Poston JN, Fromm JR, Rasmussen HA, et al. A pilot study of weekly brentuximab vedotin in patients with CD30+ malignancies resistant to brentuximab vedotin every 3 weeks. Br J Haematol. 2018;186(1):159–162.
- Bilardi RA, Kimura KI, Phillips DR, et al. Processing of anthracycline-DNA adducts via DNA replication and interstrand crosslink repair pathways. Biochem Pharmacol. 2012;83(9):1241–1250.
- Rylova SN, Del Pozzo L, Klingeberg C, et al. Immuno-PET imaging of CD30-positive lymphoma using 89Zr-desferrioxamine-labeled CD30-specific AC-10 antibody. J Nucl Med. 2016;57(1):96–102.
- Schnell R, Dietlein M, Staak JO, et al. Treatment of refractory Hodgkin's lymphoma patients with an iodine-131-labeled murine anti-CD30 monoclonal antibody. JCO. 2005;23(21):4669–4678.
- Zhang M, Yao Z, Patel H, et al. Effective therapy of murine models of human leukemia and lymphoma with radiolabeled anti-CD30 antibody, HeFi-1. Proc Natl Acad Sci USA. 2007;104(20):8444–8448.
- Dietlein M, Borner SM, Fischer T, et al. Development of anti-CD30 radioimmunoconstructs (RICs) for treatment of Hodgkin's lymphoma. Studies with cell lines and animal studies. Nuklearmedizin. 2010;49(3):97–105.
- Kang L, Jiang D, Ehlerding EB, et al. Noninvasive trafficking of brentuximab vedotin and PET imaging of CD30 in lung cancer murine models. Mol Pharm. 2018;15(4):1627–1634.
- Plattel WJ, Alsada ZN, van Imhoff GW, et al. Biomarkers for evaluation of treatment response in classical Hodgkin lymphoma: comparison of sGalectin-1, sCD163 and sCD30 with TARC. Br J Haematol. 2016;175(5):868–875.
- Horie R, Watanabe T. CD30: expression and function in health and disease. Semin Immunol. 1998;10(6):457–470.
- Purdue MP, Lan Q, Kemp TJ, et al. Elevated serum sCD23 and sCD30 up to two decades prior to diagnosis associated with increased risk of non-Hodgkin lymphoma. Leukemia. 2015;29(6):1429–1431.