Abstract
Cervical cancer is the second most common malignant tumour threatening women’s health. In recent years, heavy-ion beam therapy is becoming a newly emerging therapeutic mean of cancer; however, radio-resistance and radiation-induced damage constitute the main obstacles for curative treatment of cervical cancer. Therefore, to identify the radiosensitizers is essential. Here, we investigated the effects of Wnt signalling pathway on the response of 12C6+ radiation in HeLa cells. XAV939, an inhibitor of Wnt signalling pathway, was added two hours before 12C6+ radiation.12C6+ radiation inhibited the viability of HeLa cells in a time-dependent manner, and inhibiting Wnt signalling using XAV939 significantly intensified this stress. Meanwhile, 12C6+ radiation induced a significant increased cell apoptosis, G2/M phase arrest, and the number of γ-H2AX foci. Supplementation with XAV939 significantly increased the effects induced by 12C6+ radiation alone. Combining XAV939 with 12C6+ irradiation, the expression of apoptotic genes (p53, Bax, Bcl-2) was significantly increased, while the expression of Wnt-related genes (Wnt3a, Wnt5a, β-catenin, cyclin D1 and c-Myc) was significantly decreased. Overall, these findings suggested that blockage of the Wnt/β-catenin pathway effectively sensitizes HeLa cells to 12C6+ irradiation, and it may be a potential therapeutic approach in terms of increasing the clinical efficacy of 12C6+ beams.
Introduction
Cervical carcinoma is one of the most common and lethal gynaecological malignancy in women worldwide, and its mortality rates only rank second to breast cancer [Citation1]. Previous studies have confirmed that the sustained high-risk human papillomavirus (HPV) infection is the prerequisite for the initiation and progression of cervical cancer [Citation2]. The conventional treatments for cervical cancer are surgery, radiotherapy, chemotherapy and molecular targeted therapy [Citation3]. Radiotherapy remains the mainstay of treatment, especially in advanced cervical cancer [Citation4]. Up to now, nearly about 70% of cancer patients have applied radiotherapy to treat tumour, which is considered as the standard treatment for locally advanced cervical cancer.
Recently, 12C6+ radiotherapy, as a highly intensive local therapy, has become one of the most used therapeutic strategies for various malignancies. Compared to conventional photon and proton irradiation, 12C6+ radiotherapy induces a stronger lethal effect on cancer cells due to the unique physical and biological effects, including superior physical dose distribution (spread-out Bragg peak) [Citation5], smaller volume of irradiated normal tissue [Citation6], higher relative biological effectiveness, lower oxygen enhancement ratio, unrepaired DNA damage and nearly unchanged radio-sensitivity within the cell cycle [Citation7–9]. This results in a cell-killing effect that is two to three times greater than that of X-rays [Citation5,Citation10]. Accumulating evidence suggests that 12C6+ irradiation has been widely used in the treatment of a variety of cancers, such as pancreatic cancer [Citation11], non-small cell lung cancer [Citation12], locally advanced cervical cancer, prostate carcinoma [Citation13] and breast cancer [Citation14]. What is more, in the stage IVA patients of cervical carcinoma, 12C6+ beam radiotherapy has respectively shown a 3-year local control and overall survival rate of 66% and 47%, indicating that it can be applied to locally advanced cervical cancer [Citation15]. Thus far, it is estimated that more than 16,000 patients have received 12C6+ treatment. In spite of the fact that 12C6+ radiotherapy can be applied for cervical cancer treatment, there are still some limitations for haematological diseases, tumours around vital organs and patients with distant metastases [Citation16,Citation17]. Therefore, the identification of high-efficiency and low-toxic radiation sensitizers for cancer to improve the radio-sensitivity is required.
The Wnt/β-catenin signalling is a highly evolutionary conserved pathway that is implicated in many vital biological processes, such as embryonic development, stem cell regeneration, tissue homeostasis and cell survival [Citation18–20]. Studies have shown Wnt/β-catenin signalling is often upregulated in a variety of cancer, which endows cells with a stem-like phenotype that enhances self-renewal ability, multi-differential potential, and induces epithelial-to-mesenchymal transition of cancer cells [Citation21]. Moreover, aberrant activation of canonical Wnt signalling is usually associated with cancer cell-elicited immunosuppression, metastasis, and increased cancer cell tolerance to chemo/radiotherapy or hormonal therapy [Citation21,Citation22]. Previous studies showed that activation of the Wnt/β-catenin signalling pathway may play a key role in the progression of cervical cancer following persistent HPV infection [Citation23]. Accordingly, inhibition of Wnt/β-catenin pathway may be a novel and attractive strategy for the treatment of cancer.
XAV939 was characterized as a small molecule inhibitor of Wnt signalling pathway, which could block Wnt signalling in cancer cell lines through binding to tankyrase (TNKS) catalytic poly-ADP-ribose polymerase (PARP) domain, in turn, results in dramatic stabilization of the Axin protein, thereby leads to inhibition of transcription regulated by β-catenin [Citation24,Citation25]. Besides, compared with other Wnt pathway inhibitors, XAV939 has a strong specificity for the Wnt signalling pathway without affecting NF-κB or TGF-β signalling pathways [Citation22]. Therefore, XAV939 may be a potential radiosensitizer for the treatment of tumours.
In this study, to provide a biological basis for the combined use of XAV939 with carbon-ion beam radiotherapy, we investigated the ability of XAV939 to sensitize a human cervical carcinoma HeLa cell line to 12C6+ beam irradiation in vitro.
Materials and methods
Cell culture and irradiation
Human cervical carcinoma cell line HeLa was obtained from the First Hospital of Lanzhou University. All cells were maintained in Dulbecco’s modified Eagle medium (DMEM; HyClone™, Logan, UT) containing 10% (v/v) heat-inactivated foetal bovine serum (FBS; HyClone™, Logan, UT, USA) and were cultured in a humidified incubator of 5% CO2 at 37 °C.
HeLa cells were plated on 35-mm plastic dishes and irradiated with 12C6+ beam at room temperature. Heavy ion beam was provided by the Heavy Ion Research Facility in Lanzhou (HIRFL, Institute of Modern Physics, Chinese Academy of Sciences, Lanzhou, China), with energy of 80 MeV/u, LET at the centre of the spread-out Bragg peak of 50 keV/µm, and dose rate of 4 Gy/min.
Chemical exposure and experimental design
XAV939 (purity ≥ 98%) was purchased from Sigma-Aldrich and was solubilized in dimethyl sulphoxide (DMSO; Sigma-Aldrich, St. Louis, MO, USA).
The cells were divided into the following five groups: (a) the control group; (b) DMSO group, which were treated with DMSO diluted 3000 times; (c) XAV939 group, which were exposed to 5 μM XAV939 before and after radiation; (d) IR (ionizing radiation) group, which received 12C6+ radiation at dose of 2 Gy and (e) XAV939 + IR group, which simultaneous received treatment of 5 μM XAV939 and irradiation. We respectively pre-treated with DMSO and XAV939 for 2 h before irradiation and kept it in the medium until collecting samples. All the tests were separately repeated three times.
Cell viability detection
Cell proliferation activity was determined using the cell count kit 8 (CCK-8; Dojindo, Kumamoto, Japan). After irradiation, cell lines were seeded in triplicate at a density of 3000, 2000, 1500, 1000 and 500 per well in 96-well plates with 200 μl of complete medium per well. After incubation for 6, 12, 24, 48, 72, 96 h, 20 µl CCK8 was added to each well. Following 2 h incubation at 37 °C, the absorbance was measured at 450 nm by using a microplate reader (Tecan infinite 200M, Männedorf, Switzerland).
Colony-forming assay
Immediately after irradiation, cells were trypsinized and resuspended in complete medium. Appropriate cell densities were seeded in triplicate into 60 mm dish. After culturing for 10–14 days, cells were fixed with 4% paraformaldehyde and dyed with 1% crystal violet. Colonies consisting of more than 50 cells were counted as surviving cells. The plating efficiency (PE) was the ratio of the number of colonies to the number of cells plated in control. The surviving fraction (SF) of the treated group was calculated as follows: SF = colonies counted/(cells seeded × PE). Survival curve parameters were determined by using the linear-quadratic equation.
Cell apoptosis assay
Apoptosis was measured with FITC Annexin V apoptosis detection kit I (BD, Franklin Lakes, NJ) and followed the manufacturer’s instructions. Cells were washed twice with cold PBS and resuspended in 150 µl 1× binding buffer, and then incubated in the dark with 5 µl FITC Annexin V and 5 µl propidium iodide (PI) for 15 min at room temperature. Fluorescence of the cells was immediately analyzed on a flow cytometer FlowSight (Amnis, Seattle, WA). Subsequently, the results were analyzed by IDEAS Application v6.0 software. At least 10,000 cells were counted for each sample.
Cell cycle assay
Following treatment with different conditions for 24 h, the cells were trypsinized and fixed with 70% ethanol for 30 min at 4 °C. Then, centrifuged and removed the supernatant. Meanwhile, added 3 ml PBS for 15 min to rehydrate the cells. After washing twice with cold PBS, the cells were incubated with 100 μl DNA Staining solution (MultiSciences, Hangzhou, China) for 30 min in the dark. FlowJo-V10 software served to analyze the results. A minimum of 10,000 cells was examined per sample.
Immunofluorescence staining of γ-H2AX and β-catenin
H2AX phosphorylation andβ-catenin expression in cells were evaluated by the immunocytochemistry assay method. Cells of different treatment groups were grown on Φ20 mm glass-bottom cell culture dishes for 4 h, respectively. Cells were then fixed with 4% fixative solution, permeabilized with 0.5% Triton X-100 and subsequently blocked using 10% bovine serum albumin (BSA) in TBST. Afterwards, cells were respectively incubated with a primary antibody against γ-H2AX (1:200) (Abcam, Cambridge, MA)or β-catenin (1:300) (Cell Signaling Technology, Inc., Beverly, MA) overnight at 4 °C, after which an FITC-labelled secondary antibody (1:1000) (Immunoway, Beijing, China) was added and incubated in the dark for 1 h at room temperature. Finally, detection was performed after the cell nuclei were counterstained with DAPI (Vector Laboratories, Burlingame, CA) for 5 min. Cell images were captured using a Zeiss LSM-700 confocal microscope (Carl Zeiss, Jena, Germany) (magnification, ×400).
Western blot analysis
Equal numbers of HeLa cells were cultured on 35 mm dishes and treated with different conditions for 24 h. The cells were then lysed with RIPA buffer (Solarbio, Beijing, China) containing PMSF for 30 min on ice, and the total protein content was determined using the BCATM protein assay kit (Pierce, Rockford, IL). Equal amounts of denatured protein samples were separated on 15% SDS-PAGE and transferred onto PVDF membrane. To prevent non-specific binding, the membranes were blocked with 5% BSA at room temperature for 1 h, and subsequently incubated overnight at 4 °C with the primary antibodies against p53 (1:1000), Bax (1:500), Bcl-2 (1:500), Wnt3a (1:1000), Wnt5a (1:500), cyclin D1 (1:500), c-Myc (1:500) (GeneTex, Inc., Irvine, CA) and β-catenin (1:4000) (Cell Signaling Technology, Inc., Beverly, MA). After washing three times with TBST, the HRP-labelled secondary antibody (1:10,000) (Bioworld, Louis Park, MN) was added to the membranes for 1 h. Following reaction with the secondary antibody, the protein expression was visualized using chemiluminescence reagents (Millipore, Darmstadt, Germany) and was analyzed by AlphaView SA software. Meanwhile, the GAPDH levels were used to normalize the results.
Statistical analyses
Measurement data were presented as the mean ± SEM. Statistical analyses wer evaluated using IBMSPSS Statistics 20 software (SPSS Inc., Chicago, IL). One-way analysis of variance (ANOVA) completed with LSD post hoc test was applied to determine the statistically significant differences among groups with a p value <.05 considered as a statistically significant difference. The figures were plotted using Origin8.0 (OriginLab, Northampton, MA).
Results
Assessment of optimal concentration of XAV939 in HeLa cells
To assess the cytotoxicity of XAV939 itself, HeLa cells were treated with several concentrations of XAV939, and CCK-8 assay showed that pre-treatment of XAV939 dramatically decreased cell viability in a concentration-dependent manner from 5 to 20 μM, and had no statistically significant difference under 5 μM compared to the control group (). To minimize its toxicity on HeLa cells, 5 μM XAV939 was selected for further study.
Figure 1. XAV939 inhibited cell viability in HeLa cells exposed to 2 Gy 12C6+ radiation. (A) CCK-8 assay used to reveal the proliferative activity of HeLa cells affected by XAV939. (B) The viability of HeLa cells was analyzed by CCK8 assay. Histogram was showed for each treatment at 61,224 h post 12C6+ radiation with or without 5 μM XAV939. The data represent the mean ± SEM of triplicate experiments. *p < .05 (vs. control group). #p < .05 (vs. radiation group).
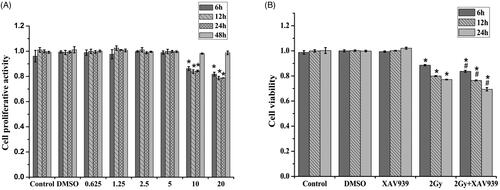
XAV939 sensitizes human cervical carcinoma cells to 12C6+ irradiation
Time course of HeLa cell proliferation in five treatments IR(–)/XAV939(–), IR(–)/DMSO(+), IR(–)/XAV939(+), IR(+)/XAV939(–) and IR(+)/XAV939(+) was assessed by CCK8 assay. As shown in , compared with the control group, 12C6+ irradiation led to decreased cell viability and growth in a time-dependent manner within 24 h, and supplementation with XAV939 was able to significantly enhance these effects. Compared with the control group, cell viability approximately reduced 23.07% (p < .05) at 24 h when treated with 2 Gy 12C6+ irradiation. When XAV939 was present 2 h before the irradiation, compared with the irradiation-only groups, the cell viability was obviously decreased from 77.12 ± 0.13% to 69.39 ± 1.34% (p < .05). Both DMSO and XAV939 treatment alone had no significant difference in proliferation within 24 h compared with the control (p > .05). The results showed that XAV939 could inhibit cell proliferation after 12C6+ radiation.
The clonogenic survival assay was used to confirm whether XAV939 treatment contributed to improving the radio-sensitivity. The results revealed that the PE of untreated cells was 53.7 ± 5.0%, and the survival fraction of HeLa cells treated with XAV939 was obviously lower after 12C6+ radiation than that of irradiation cells alone (). Our results revealed that the survival fraction of HeLa cells decreased markedly in a dose-dependent manner after 2 Gy and 4 Gy 12C6+ irradiation, approximately 65% (p < .05) and 85% (p < .05), respectively, compared with the control group. Meanwhile, after XAV939 pre-treatment both in the 2 Gy and 4 Gy irradiation group, the rate of colony formation further reduced significantly from 35.00 ± 2.25% to 26.00 ± 1.06%, 15.07 ± 3.07% to 6.77 ± 0.81%, respectively (p < .05), compared with the irradiation-only groups. Collectively, these data demonstrated that XAV939 reduced cell viability and inhibited cell proliferation after 12C6+ radiation, leading to increased radiosensitivity of HeLa cells.
Figure 2. XAV939 could increase cellular radioresistance in HeLa cells. (A) Representative images of colony forming potential of HeLa cells in different treatment groups. (B) Cell surviving fraction curve was performed with cell clonogenic survival assay. Cells were pre-treated with or without XAV939 for 2 h before 12C6+ radiation and plated for survival. Colonies consisting of more than 50 cells were scored. The data represent the mean ± SEM of triplicate measurements. All experiments were performed in three independent experiments. *p < .05 (vs. control group). #p < .05 (vs. radiation group).
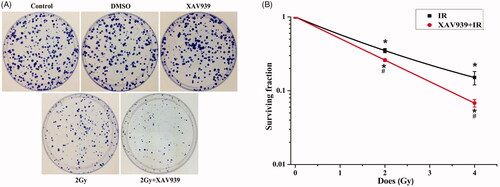
Effect of XAV939 on proteins involved in Wnt/β-catenin pathway in irradiated HeLa cells
The HeLa cells were treated with irradiation alone or combined with XAV939 (5 µM) for 24 h, respectively. Western blot assay was performed to detect related proteins of the Wnt signalling pathway. The study found there was a significant difference in the expression of β-catenin, Wnt3a, Wnt5a, cyclin D1 and c-Myc among the groups. Our results showed that, compared with the irradiation-only alone, the combination treatment with 5 µM XAV939 and 12C6+ beam induced obvious decrease in the expression of β-catenin (1.24-fold, p < .05), Wnt3a (1.71-fold, p < .05), Wnt5a (1.74-fold, p < .05), cyclin D1 (1.49-fold, p < .05) and c-Myc (1.74-fold, p < .05) (). Therefore, XAV939 might contribute to radio-sensitivity by inhibiting protein activity involved in Wnt/β-catenin signalling pathway.
Figure 3. Effect of XAV939 on proteins involved in Wnt/β-catenin pathway in irradiated HeLa cells. (A) Representative western blot images. (B) Quantitative analysis of the expression of Wnt5a, Wnt3a, β-catenin, cyclin D1 and c-Myc were represented by column graphs. GAPDH was used as a loading control. (C) Subcellular localization of β-catenin was examined using confocal microscopy (green). Nuclei were counterstained with DAPI (blue). All experiments were performed in triplicate. The data are expressed as the mean ± SEM. *p < .05 (vs. control group). #p < .05 (vs. radiation group).
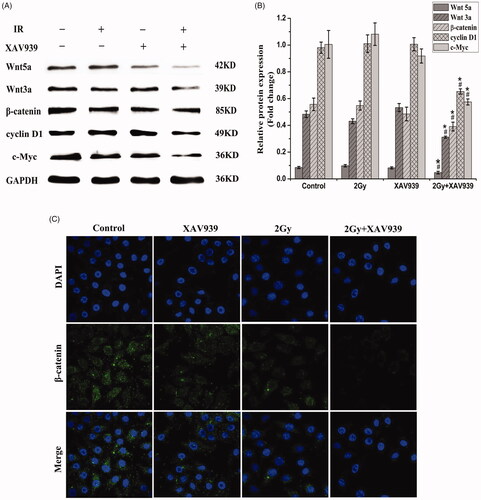
XAV939 induces G2/M arrest in irradiated HeLa cells
It has been proposed that the alteration of cell cycle progression post-irradiation closely related to cell sensitivity [Citation26]. Therefore, we examined the effects of 12C6+ beam irradiation alone and in combination with XAV939 on cell cycle distribution using flow cytometry after irradiated 24 h. As shown in , the G2/M proportion of cells with irradiation treatment was more pronounced than that of control cells (34.20 ± 1.31% vs. 19.93 ± 2.37%, p < .05). Additionally, these effects were largely strengthened by supplement with XAV939 administration (44.90 ± 0.85% vs. 34.20 ± 1.31%, p < .05), which may contribute to the decreased cell viability.
Figure 4. XAV939 enhances radiation-induced G2/M phase arrest in HeLa cells. (A) Cell cycle distribution in HeLa cells pre-treated with or without XAV939 before 2 Gy 12C6+ radiation at the time point of 24 h. Different treatment cells were stained with PI and detected for DNA content using flow cytometry. (B) Quantitative analysis of the percentage of the cell population in each phase after exposure to 2 Gy 12C6+ beam for 24 h. The figure shows the mean ± SEM calculated from three independent experiments. *p < .05 (vs. control group). #p < .05 (vs. radiation group).
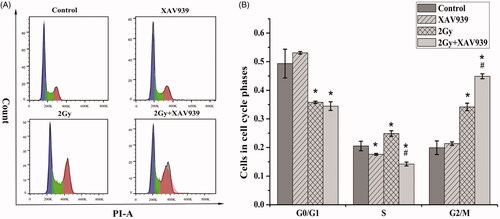
XAV939 induces DNA damage in irradiated HeLa cells
Similar results were drawn on the expression of phosphorylated histone H2AX (γ-H2AX), the biomarker of DNA double-strand breaks (DSBs). In the present study, a cell immunofluorescence assay was applied to assess the effects of XAV939on DNA damage accumulation after irradiation in HeLa cells. As shown in , the present study showed a much more γ-H2AX foci were observed in the nuclei after exposure to 2 Gy 12C6+ beams than control cells, and the addition of XAV939 obviously increased H2AX phosphorylation in the irradiation HeLa cells relative to the irradiation alone. These data revealed that XAV939 may sensitize cervical cancer cells to 12C6+ radiation by suppressing DNA damage repair capacity and accumulating unrepaired lethal DNA lesions in the cells.
XAV939 induces apoptosis in irradiated HeLa cells
At 24 h after 2 Gy 12C6+ beam irradiation, apoptosis of HeLa cells was examined by using flow cytometry after Annexin V-FITC/PI staining. Our results indicated that there was no significant difference in apoptosis rate among the groups for control, DMSO and XAV939 treatment alone (p > .05). However, cells treated with 12C6+ radiation-induced more apoptosis than the control group (14.51-fold, p < .05). Moreover, the data demonstrated XAV939 dramatically enhanced IR-induced apoptosis, with a 46.53% (p < .05) increase of apoptotic ratio in comparison with that of cells exposed to IR ().
Figure 6. Combination XAV939 with 12C6+ radiation increased the apoptosis rate in HeLa cells. (A) HeLa cells from different treatment groups were incubated for 24 h and cell apoptosis was subsequently detected using co-staining with Annexin V-FITC and PI flow cytometry. (B) Quantitative analysis of apoptosis in different treatment groups. The apoptosis percentages of HeLa cells show early (Annexin V + and PI–) and late (Annexin V + and PI+) apoptotic cells. (C) Expression of apoptosis-associated proteins, including Bcl-2, Bax and p53 were analysed by western blot assay. GAPDH protein was used as an internal control. (D) Quantitative analysis of the Bcl-2, Bax and p53 proteins is represented by column graphs. Bars are mean ± SEM from three independent experiments. *p < .05 (vs. control group). #p < .05 (vs. radiation group).
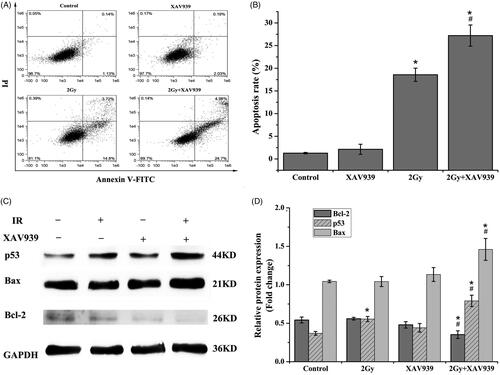
Since XAV939 pre-treatment had an obvious response on 12C6+ irradiation-induced apoptosis, the possible molecular mechanisms were further analyzed. We investigated the induction of apoptosis by irradiation with or without XAV939 using western blotting assay. As shown in , treatment with XAV939 alone has no obvious differences in the expression of pro-apoptotic p53 and Bax and apoptotic-profilin Bcl-2. However, combined XAV939 and heavy ion beams treatment resulted in an increase in the expression of p53 (1.79-fold, p < .05) and Bax (1.29-fold, p < .05) in conjunction with the down-regulation expression of Bcl-2 (1.36-fold, p < .05), compared with heavy-ion beams radiation. Together, these results concluded that the induction of apoptosis was effectively enhanced by XAV939 in irradiated HeLa cells.
Discussion
12C6+ radiotherapy is increasingly becoming a novel treatment strategy for locally advanced cervical cancer due to its unique physical and biological effects. In spite of the fact that carcinogenesis and 12C6+ beam therapy have been studied extensively, there is still space to improve the radio-sensitivity to cervical cancer [Citation4,Citation27]. It was proved that Wnt signalling pathway may play an important role in the development of cervical cancer because the activation of the Wnt/β-catenin pathway is indispensable to induce the transformation of HPV immortalized cells [Citation2,Citation28]. Thus, Wnt/β-catenin signalling has increasingly become a promising therapeutic target in antitumor therapy. Currently, there are several ongoing clinical trials of Wnt signalling pathway inhibitors that have been used to restrain tumour growth. XAV939 is a small molecule TNKS inhibitor targeting Wnt signalling pathway, which inhibits the abnormal activation of Wnt/β-catenin without affecting the normal function of cells [Citation21,Citation22]. Therefore, XAV939 is considered as a potential adjuvant to radiotherapy. This work provides first-hand information about the radio-sensitization effects of XAV939 on HeLa cells.
XAV939 was introduced to combine with 12C6+ radiation for its specific inhibition of Wnt/β-catenin signalling for the first time. A series of studies have manifested XAV939 can inhibit the viability of breast, ovarian [Citation29], colon, lung, neuroblastoma cancer cells [Citation30] and leiomyoma cell by blocking Wnt signalling pathway [Citation31,Citation32]. To evaluate whether XAV939 had toxic effects on HeLa cells, a CCK-8 assay was used. In the present study, significant differences were observed in a concentration-dependent manner; however, there were no statistical significant differences as time increased. Our results implied that cells treated with 5 μM XAV939 from 6 h to 96 h had no obvious effect on cell proliferation. Therefore, the 5 μM XAV939 was selected as an adequate concentration for this study; same as previous studies which showed 5 μM XAV939 had no significant effect on proliferation and viability of LNCaP cells [Citation21]. Meanwhile, our study demonstrated following the treatment of 12C6+ radiation, an obvious decrease in cell viability of HeLa cells, evaluated using a CCK-8 assay, were clearly observed. However, when XAV939 was present 2 h before the irradiation, the inhibition of cell viability of HeLa cells was strengthened dramatically compared with irradiation alone, indicating a significant interaction between XAV939 and radio-sensitivity in HeLa cells. Comparable results were found when detecting the clonogenic capacity of HeLa cells after XAV939 and irradiation treatments. HeLa cells treated with a combination of XAV939 and 12C6+ irradiation showed greater clone-forming capability suppression relative to cells treated with XAV939 or irradiation alone; same as the previous studies which revealed the co-administration of XAV939 and γ-ray inhibited MB cells proliferation and clonogenic capacity, and decreased their efficacy in repairing DNA damage, and increased irradiation-induced cell mortality [Citation33].
We further analyzed the possible mechanisms that might be implicated in XAV939-induced radiation sensitization. XAV939 may suppress the activity of TNKS and stabilize Axin levels, thereby blocking the accumulation of β-catenin in the cytoplasm [Citation34,Citation35]. β-Catenin, as the central effector, plays a key role in the Wnt/β-catenin signalling cascade [Citation36], whether the canonical Wnt pathway is activated or does not depend on the stability of β-catenin in the cytosol [Citation37]. In the absence of activated Wnt signalling, β-catenin is actively degraded to maintain a low level of β-catenin in the cytosol. However, when canonical Wnt signalling is activated, β-catenin accumulates in the cytosol and migrates to the nucleus, where it interacts with the T-cell factor/lymphocyte enhancer factor and co-activators to stimulate the transcription of the downstream target genes, such as c-Myc, cyclin D1, thus promoting uncontrolled cell proliferation [Citation38,Citation39]. It was demonstrated that XAV939 could suppress cell growth and proliferation in neuroblastoma cell lines through the inhibition of TNKS1 [Citation37]. Moreover, accumulated evidence has shown that XAV939 sensitizes colorectal cancer cells to irradiation via the inhibition of β-catenin [Citation40]. Additionally, it was also reported that nuclear β-catenin regulates the transcription of cyclinD1 and c-Myc, which are implicated in cell cycle progression [Citation41]. In the present study, western blot assay was performed to evaluate the expression of proteins involved in Wnt/β-catenin signalling pathway. The results indicated that the combination of 12C6+ irradiation with XAV939 obviously reduced the expression of Wnt3a, Wnt5a, β-catenin, cyclin D1 and c-Myc induced by 12C6+ irradiation alone, suggesting XAV939 may exert the inhibition effects by reducing the expression of the key protein involved in the Wnt signalling pathway.
It is widely accepted that a primary mechanism of 12C6+ irradiation is to induce DNA DSBs of tumour cells [Citation42]. Histone γ-H2AX is an important sensor of DSBs after irradiation treatment [Citation43,Citation44]. Effects of XAV939 and irradiation on DNA damage accumulation in HeLa cells were analyzed using immunofluorescence staining. Our study showed the combination of 5 μM XAV939 and irradiation with 12C6+ beam significantly increased the H2AX phosphorylation at 4 h after irradiation compared to irradiation alone, indicating inhibition of DNA repair ability and the accumulation of unrepaired lethal DNA damage in the cells after XAV939 treatment. Meanwhile, previous studies indicated that blocking the activity of canonical Wnt signalling in colorectal and other Wnt-deregulated cancers leads to cell-cycle arrest in the G1 phase, thereby inhibiting the growth of cancer cells [Citation31]. Converging evidence also showed XAV939 dramatically improved the sensitivity of colon cancer cell lines to chemotherapy by inducing cellular apoptosis [Citation45]. In this study, we observed XAV939 markedly enhanced irradiation-induced apoptosis and G2/M cell cycle arrest. What is more, we also demonstrated that, compared with irradiation alone, p53 and pro-apoptosis protein Bax was vastly upregulated in IR(+)/XAV939(+) group, whereas the expression of anti-apoptotic protein Bcl2 was significantly downregulated. Cellular responses to DNA damage, including apoptosis and cell cycle arrest, are the critical factors determining the cancer prognosis after radiotherapy [Citation46]. Here, we concluded that XAV939 contributed to HeLa cell radio-sensitivity by promoting 12C6+ irradiation-induced apoptosis, G2/M arrest and DNA damage. Hence, XAV939 exhibited potent radio-sensitizing effects to 12C6+ irradiation HeLa cells.
To sum up, our in vitro data exhibit the closed correlation among XAV939, radio-sensitivity and Wnt signalling pathway in cervical cancer cells. It is the first proof up to date that XAV939 has the potential to sensitize HeLa cells to 12C6+ irradiation through the inhibition of Wnt/β-catenin signalling pathway. However, it is imperative to further dig out the effects of XAV939 on radio-sensitivity using tumour-bearing animal model and clinical experiments in the near future.
Acknowledgements
We express our thanks to the accelerator crew at the HIRFL, Institute of Modern Physics, Chinese Academy of Sciences, China.
Disclosure statement
The authors have declared that no competing interest exists.
Additional information
Funding
References
- Saei Ghare Naz M, Kariman N, Ebadi A, et al. Educational interventions for cervical cancer screening behavior of women: a systematic review. Asian Pac J Cancer Prev. 2018;19:875–884.
- Manzo-Merino J, Contreras-Paredes A, Vazquez-Ulloa E, et al. The role of signaling pathways in cervical cancer and molecular therapeutic targets. Arch Med Res. 2014;45(7):525–539.
- Wang L, Zhao Y, Wang Y, et al. The role of galectins in cervical cancer biology and progression. Biomed Res Int. 2018;2018:2175927.
- Di C, Sun C, Li H, et al. Diallyl disulfide enhances carbon ion beams-induced apoptotic cell death in cervical cancer cells through regulating Tap73/DeltaNp73. Cell Cycle. 2015;14(23):3725–3733.
- Oike T, Niimi A, Okonogi N, et al. Visualization of complex DNA double-strand breaks in a tumor treated with carbon ion radiotherapy. Sci Rep. 2016;6(1):22275.
- Kubo N, Noda SE, Takahashi A, et al. Radiosensitizing effect of carboplatin and paclitaxel to carbon-ion beam irradiation in the non-small-cell lung cancer cell line H460. J Radiat Res. 2015;56(2):229–238.
- Jin X, Li F, Zheng X, et al. Carbon ions induce autophagy effectively through stimulating the unfolded protein response and subsequent inhibiting Akt phosphorylation in tumor cells. Sci Rep. 2015;5(1):13815.
- Zhang P, Hu X, Liu B, et al. Effects of 12C6+ heavy ion radiation on dendritic cells function. Med Sci Monit. 2018;24:1457–1463.
- Kamada T, Tsujii H, Blakely EA, et al. Carbon ion radiotherapy in Japan: an assessment of 20 years of clinical experience. Lancet Oncol. 2015;16(2):e93–e100.
- Ando K, Kase Y. Biological characteristics of carbon-ion therapy. Int J Radiat Biol. 2009;85(9):715–728.
- Steitz J, Naumann P, Ulrich S, et al. Worst case optimization for interfractional motion mitigation in carbon ion therapy of pancreatic cancer. Radiat Oncol. 2016;11(1):134.
- Keta OD, Todorovic DV, Bulat TM, et al. Comparison of human lung cancer cell radiosensitivity after irradiations with therapeutic protons and carbon ions. Exp Biol Med (Maywood). 2017;242(10):1015–1024.
- Glowa C, Peschke P, Brons S, et al. Carbon ion radiotherapy: impact of tumor differentiation on local control in experimental prostate carcinomas. Radiat Oncol. 2017;12(1):174.
- Zhou C, Rong Y, Konishi T, et al. Effect of carbon-ion radiation on drug transporters organic anion transporting polypeptides in breast cancer cells. Radiat Res. 2017;187(6):689–700.
- Shiba S, Wakatsuki M, Kato S, et al. Carbon-ion radiotherapy for locally advanced cervical cancer with bladder invasion. J Radiat Res. 2016;57(6):684–690.
- Kim EH, Kim MS, Furusawa Y, et al. Metformin enhances the radiosensitivity of human liver cancer cells to ϒ-rays and carbon ion beams. Oncotarget. 2016;7(49):80568–80578.
- Vigano L, Capussotti L, De Rosa G, et al. Liver resection for colorectal metastases after chemotherapy: impact of chemotherapy-related liver injuries, pathological tumor response, and micrometastases on long-term survival. Ann Surg. 2013;258:731–740.
- Ge YX, Wang CH, Hu FY, et al. New advances of TMEM88 in cancer initiation and progression, with special emphasis on Wnt signaling pathway. J Cell Physiol. 2018;233(1):79–87.
- Jing Q, Li G, Chen X, et al. Wnt3a promotes radioresistance via autophagy in squamous cell carcinoma of the head and neck. J Cell Mol Med. 2019;23(7):4711–4722.
- Yang G, Shen T, Yi X, et al. Crosstalk between long non-coding RNAs and Wnt/beta-catenin signalling in cancer. J Cell Mol Med. 2018;22(4):2062–2070.
- Stakheev D, Taborska P, Strizova Z, et al. The WNT/β-catenin signaling inhibitor XAV939 enhances the elimination of LNCaP and PC-3 prostate cancer cells by prostate cancer patient lymphocytes in vitro. Sci Rep. 2019;9(1):4761.
- Guo W, Shen F, Xiao W, et al. Wnt inhibitor XAV939 suppresses the viability of small cell lung cancer NCI-H446 cells and induces apoptosis. Oncol Lett. 2017;14:6585–6591.
- Perez-Plasencia C, Duenas-Gonzalez A, Alatorre-Tavera B. Second hit in cervical carcinogenesis process: involvement of Wnt/beta catenin pathway. Int Arch Med. 2008;1(1):10.
- Afifi MM, Austin LA, Mackey MA, et al. XAV939: from a small inhibitor to a potent drug bioconjugate when delivered by gold nanoparticles. Bioconjug Chem. 2014;25(2):207–215.
- Pan F, Shen F, Yang L, et al. Inhibitory effects of XAV939 on the proliferation of small-cell lung cancer H446 cells and Wnt/β-catenin signaling pathway in vitro. Oncol Lett. 2018;16:1953–1958.
- Mao A, Zhao Q, Zhou X, et al. MicroRNA-449a enhances radiosensitivity by downregulation of c-Myc in prostate cancer cells. Sci Rep. 2016;6(1):27346.
- Durante M. New challenges in high-energy particle radiobiology. Br J Radiol. 2014;87(1035):20130626.
- Uren A, Fallen S, Yuan H, et al. Activation of the canonical Wnt pathway during genital keratinocyte transformation: a model for cervical cancer progression. Cancer Res. 2005;65:6199–6206.
- Bocchicchio S, Tesone M, Irusta G. Convergence of Wnt and Notch signaling controls ovarian cancer cell survival. J Cell Physiol. 2019;234(12):22130–22143.
- Suebsoonthron J, Jaroonwitchawan T, Yamabhai M, et al. Inhibition of WNT signaling reduces differentiation and induces sensitivity to doxorubicin in human malignant neuroblastoma SH-SY5Y cells. Anticancer Drugs. 2017;28(5):469–479.
- Ono M, Yin P, Navarro A, et al. Inhibition of canonical WNT signaling attenuates human leiomyoma cell growth. Fertil Steril. 2014;101(5):1441–1449.
- Ma L, Wang X, Jia T, et al. Tankyrase inhibitors attenuate WNT/β-catenin signaling and inhibit growth of hepatocellular carcinoma cells. Oncotarget. 2015;6(28):25390–25401.
- Renna C, Salaroli R, Cocchi C, et al. XAV939-mediated ARTD activity inhibition in human MB cell lines. PLoS One. 2015;10(4):e0124149.
- Tai D, Wells K, Arcaroli J, et al. Targeting the WNT signaling pathway in cancer therapeutics. Oncologist. 2015;20(10):1189–1198.
- Krishnamurthy N, Kurzrock R. Targeting the Wnt/β-catenin pathway in cancer: update on effectors and inhibitors. Cancer Treat Rev. 2018;62:50–60.
- Tortelote GG, Reis RR, de Almeida Mendes F, et al. Complexity of the Wnt/betacatenin pathway: searching for an activation model. Cell Signal. 2017;40:30–43.
- Tian XH, Hou WJ, Fang Y, et al. XAV939, a tankyrase 1 inhibitor, promotes cell apoptosis in neuroblastoma cell lines by inhibiting Wnt/β-catenin signaling pathway. J Exp Clin Cancer Res. 2013;32(1):100.
- Hu XY, Hou PF, Li TT, et al. The roles of Wnt/β-catenin signaling pathway related lncRNAs in cancer. Int J Biol Sci. 2018;14(14):2003–2011.
- Taciak B, Pruszynska I, Kiraga L, et al. Wnt signaling pathway in development and cancer. J Physiol Pharmacol. 2018;69(2):185–196.
- Emons G, Spitzner M, Reineke S, et al. Chemoradiotherapy resistance in colorectal cancer cells is mediated by Wnt/β-catenin signaling. Mol Cancer Res. 2017;15(11):1481–1490.
- Yamada N, Noguchi S, Mori T, et al. Tumor-suppressive microRNA-145 targets catenin δ-1 to regulate Wnt/β-catenin signaling in human colon cancer cells. Cancer Lett. 2013;335(2):332–342.
- Che Y, Li J, Li Z, et al. Osthole enhances antitumor activity and irradiation sensitivity of cervical cancer cells by suppressing ATM/NF-κB signaling. Oncol Rep. 2018;40:737–747.
- Ma H, Takahashi A, Yoshida Y, et al. Combining carbon ion irradiation and non-homologous end-joining repair inhibitor NU7026 efficiently kills cancer cells. Radiat Oncol. 2015;10(1):225.
- Fu HC, Yang YC, Chen YJ, et al. Increased expression of SKP2 is an independent predictor of locoregional recurrence in cervical cancer via promoting DNA-damage response after irradiation. Oncotarget. 2016;7(28):44047–44061.
- Wu X, Luo F, Li J, et al. Tankyrase 1 inhibitor XAV939 increases chemosensitivity in colon cancer cell lines via inhibition of the Wnt signaling pathway. Int J Oncol. 2016;48(4):1333–1340.
- Nie X, Guo E, Wu C, et al. SALL4 induces radioresistance in nasopharyngeal carcinoma via the ATM/Chk2/p53 pathway. Cancer Med. 2019;8(4):1779–1792.