Abstract
In this paper, the first bio-nano colloid including manganese oxide nanoparticles and copper nanocomplex in the presence of Amaranthus spinosus as one unwanted plant was prepared (Mn3O4/CuL bio-nanocolloid). As-prepared bio nanocolloid was analysed completely by different techniques such as FT-IR, ICP-AES, SEM, EDX, TEM and elemental analysis to have the size, structure, morphology and elements in this compound. This bio-nanocolloid showed high catalytic activity towards green oxidation reactions of alcohols using hydrogen peroxide under solvent free conditions. The longevity, easy and practical recoverability of the solid catalyst was also confirmed for six times. The natural starting material for a long-term stability catalyst preparation, using ideal oxidant (H2O2), solventless and easy work up show the great potential in scalability for actual industries applications.
Introduction
Nanocomposites, having several outstanding properties as compared to conventional monolithic materials, are one of the most important classes of the engineered compounds [Citation1]. Their possibilities in tuning the properties of nanocolloid composites gained much importance in different fields commercially and technologically [Citation2–6]. In this regards, chemical synthesis of nanocomposite materials reduces their application in various field because chemical materials can impart toxicity to them. Toxicity related to these kinds of compounds has been directly linked to the use of chemicals as reducing and capping agent [Citation7–12]. Recently, some studies have used less toxic chemical reducing agents, but these studies have used acidic/basic environment or surfactant for their synthesis, which can be toxic to environment and living organisms. Hence, the preparation of nanocolloid composites special metal matrix composites using natural substrates such as plants is becoming popular and attractive day by day to minimum or no use of harmful chemicals [Citation13]. These methods are not only eco-friendly but also less time consuming, cost effective, stable operation, efficient and safer [Citation14–19]. Among plants, weeds are plants that grow where they are not wanted and are therefore bad news to crop farmers. While, they can be very useful for many fields [Citation20–24]. They find various potential applications such as catalysts, solar cells and treatment of water, pharmaceutical industry and Li-air batteries due to their low cost, earth abundance, environmental friendliness and variable oxidation states [Citation25–29].
Oxidation reaction is one of the most important reactions in pharmaceutical industries [Citation30]. The safety of oxidation systems in large scale can influence on the hesitation to employ. Fuel, energy and oxidant are three factors in reactors for performing oxidation reactions in industry [Citation31]. Hence, green strategies attract so much attention as economical and environmental point of view. Oxidation of alcohols to aldehydes, esters and carboxylic acids, and ketones are one of the most essential chemical processes in both academic and industrial research, because alcohols can transfer to valuable products for pharmaceuticals, dyestuffs, agrochemicals and fragrances industries [Citation31–34]. Many applied homogeneous catalysts for these reactions created many challenges from the environmental point of view. Hence, increasing efforts have been devoted in developing heterogeneous catalysts. Recently, metal matrix nanocomposites including metal oxides or/and nano transition metal oxide could replace instead of heterogeneous catalyst. Particularly and ideally in aqueous solution, H2O2 is greatly attractive for releasing active oxidative species and producing water as the only by-product. In this context, employing nano metal oxides along with nano-transition metal complex to fabricate efficient catalysts for alcohols oxidation with green oxidant is highly desirable [Citation35].
To reach this goal and in continuing our works on synthesis of novel nanocatalysts for industrial application, we made copper nanocomplex (CuL) and fabricated nanocolloid including CuL nanocomplex based on Mn3O4 nanoparticles using ultrasonic irradiation in the presence of Amaranthus spinosus (AS). The catalytic activities of it were considered as a heterogeneous catalyst for selective oxidation of primary and secondary alcohols to aldehydes and ketones products, respectively. As practical application point of view, H2O2 was used as ideal oxidant under solvent free conditions.
Materials and methods
Amaranthus spinosus was collected from Mahmoodabad village, Jiroft, Iran. Cu(NO3)2, 2-hydroxynaphthalene-1-carbaldehyde and manganese nitrate tetrahydrate were purchased from Sigma-Aldrich (St. Louis, MO). 2-Amino pyridine and solvents were provided from Across and Merk Company, Iran, respectively. FT-IR spectra of L ligand, CuL nanocomplex and Mn3O4/CuL bio-nanocolloid were recorded by FT-IR spectrophotometer (NICOLET iS10). X-ray energy-dispersive spectroscopy (EDS) detector (IE 300X, Oxford Semiconductor Co., Ltd., Oxford, UK) attached to the SEM was used for analysis of morphology and percentage of elements in this novel nano-colloid. TEM microscope (Philips CM30, Eindhoven, Netherlands) was employed for observing the shape of it. An ultrasonic bath was used for synthesis of CuL nanocomplex and Mn3O4/CuL bio-nanocolloid (Ultrasonic cleaner 5200iS3 model, SolTEC, Milan, Italy, frequency 40 kHz). XRD patterns of nanocomposite and nanoparticles were determined by Philips Netherland (Eindhoven, Netherlands), X’Pert Pro model. 1H NMR and 13C NMR spectra of ligand were recorded on Varian, UNITYInova 500 MHz. Elemental analysis of ligand was considered by CHSNO, ECS4010, Italy (University of Medical Science, Kerman, Iran).
Experimental
Synthesis of ligand (L)
Schiff base ligand was obtained by addition of a solution of 2-hydroxynaphthalene-1-carbaldehyde (1.7 g) in ethanol (40 ml) to a solution of 2-aminopyridine (5 mmol, 0.94 g) in ethanol (40 ml) and the reaction mixture was stirred for 24 h at room temperature. The obtained precipitate was filtered and dried in air. Finally, the product was characterized by IR, 1H NMR and 13C NMR spectroscopy.
C16H12N2 O: anal. Found: C, 78.69; H, 4.43; N, 12.50; Calc.: C, 77.40; H, 4.87; N, 11.28, IR (KBr, cm−1): νO–H, 3365; νC=N, 1472; νCH=N, 1608; 1H NMR (301 MHz, DMSO-d6) δ 15.18 (d, J = 7.8 Hz, 1H), 9.81 (d, J = 7.7 Hz, 1H), 8.51 (dd, J = 5.0, 1.9 Hz, 1H), 8.22 (d, J = 8.4 Hz, 1H), 8.04–7.82 (m, 2H), 7.71 (dd, J = 7.9, 1.4 Hz, 1H), 7.59 (d, J = 8.1 Hz, 1H), 7.52 (ddd, J = 8.4, 7.0, 1.5 Hz, 1H), 7.37–7.25 (m, 2H), 6.84 (d, J = 9.4 Hz, 1H). 13C NMR (76 MHz, DMSO) δ 177.2, 152.8, 150.6, 148.9, 139.2, 139.2, 133.6, 129.3, 128.7, 126.4, 124.2, 123.9, 121.6, 119.61, 115.2 and 108.0.
Synthesis of copper nanocomplex (CuL)
A solution of L ligand (3 mmol or 0.74 g in 15 ml THF) was poured dropwise under ultrasonic irradiation into THF solution of Cu(NO3)2 (2 mmol or 0.56 g in 10 ml THF). After 12 h, the obtained precipitate was filtered, washed by THF, and dried in the air.
Synthesis of Mn3O4/CuL bio-nanocolloid (Mn3O4/CuL)
Four grams of AS to 200 ml of Milli-Q water and heated at 80 °C. After settling for 1 h, the extract was vacuum filtered.
Two hundred millilitres of manganese (II) nitrate tetrahydrate (0.05 M) was added to the extract solution of AS (170 ml) and water (130 ml) at pH = 9 within 10 min at room temperature under ultrasonic irradiation and Mn3O4 nanoparticles was obtained. Then, 200 μl of aqueous solution of CuL (0.01 M) was injected in it and stirred. After 20 min, Mn3O4/CuL bio-nanocolloid was obtained.
Green oxidation of alcohols using Mn3O4/CuL bio-nanocolloid
To a mixture of alcohol (2 mmol) and Mn3O4/CuL bio-nanocolloid (0.014 g) under solvent free conditions, 0.8 mmol H2O2 (16 ml) was added and the reaction mixture was stirred at 80 °C for 5 h.
Recycling procedure of Mn3O4/CuL bio-nanocolloid on the oxidation of benzyl alcohol
To a mixture of benzyl alcohol (1 mmol, 0.1 ml) and Mn3O4/CuL bio-nanocolloid (0.007 g) under solvent free conditions, H2O2 was added (0.4 mmol, 8 ml) and the reaction mixture was stirred at 80 °C for 5 h. After completion of the reaction, 1 ml ethyl acetate was added and catalyst was separated by centrifugation. Mn3O4/CuL bionanocomposite as the isolated solid phase was dried under air and reused for next runs.
Results and discussion
Green Mn3O4 nanoparticles were performed by adding manganese salts to extraction solution of AS under ultrasonic irradiation. It should be noted that at pH = 7 of AS, the mixture of MnO2, Mn3O4 and Mn2O3 was obtained () [Citation36]. While, Mn3O4 nanoparticles were gained at pH = 9 as a sole product (). shows XRD of Mn3O4 exhibiting (211) predominant orientation at 2θ = 36.1° corresponding to tetragonal structure of Mn3O4 with I41/amd (141) space group and an estimated crystallite size of 40 nm. Other different characterization peaks (112), (200), (103), (004), (220), (105), (321), (224) and (400) were at 28.9, 31, 32.3, 38.11, 44.54, 50.6, 58.78, 59.66 and 64.71°, respectively. The estimated lattice parameters are a=b = 5.7621 Å and c = 9.4696 Å which are good agreement with previous literature [Citation37]. The same set of characteristic peaks was observed in the XRD pattern of Mn3O4/CuL bio-nanocolloid, which indicates the stability of the crystalline phase of nanoparticles during the synthesis of bio nanocomposite. The average crystallite size Mn3O4/CuL bio-nanocolloid (45 nm) was estimated using the Debye–Scherrer formula. Other peaks were related to CuL nanocomplex.
Figure 1. XRD of synthesized product from manganese salt in the presence of plant at pH = 7 (a), at pH = 9 (b) and XRD of Mn3O4/CuL bio-nanocolloid (c).
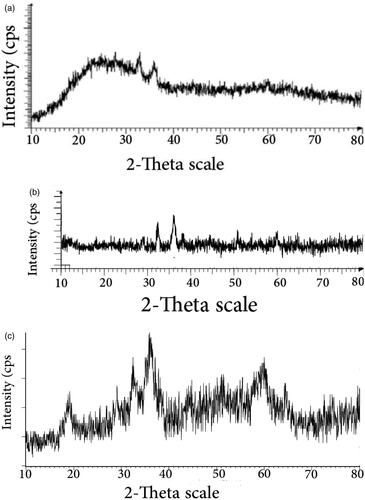
On the other hand, L ligand was obtained by condensation of 2-hydroxy 1-naphthaldehyde and 2-aminopyridine. By adding copper salt to L ligand under ultrasonic irradiation, water-soluble CuL nanocomplex was achieved. The mixture of CuL nanocomplex and Mn3O4 in the presence of AS, Mn3O4/CuL bio-nanocolloid was obtained (Scheme 1).
Proton NMR spectrum of the free ligand has been recorded in DMSO at room temperature (, top). The ligand exhibits hydroxyl proton (Ha) appearing at δ 15.203 ppm, the aromatic pyridine proton (Hb) appearing at δ 9.832 ppm, Hc appearing at δ 8.30 ppm, Hd appearing at δ 6.857 ppm, and aromatic and heteroaromatic proton signals appearing at δ 7.25–8.539 ppm. The chemical shift of hydroxyl proton is very high (15.203 ppm) indicating intramolecular hydrogen bond. 13C NMR spectrum (, down) showed similar diagnostic features for the free ligand. Hydroxyl carbon (C-9) was found at 177.642 ppm, pyridine carbon (C-1) at 151.059 ppm and imine carbon (C-7) at 149.4526 ppm, and aromatic carbons were found at 108.474–149.426 ppm.
FT-IR spectra of L ligand, CuL nanocomplex and Mn3O4/CuL bio-nanocolloid are shown at . In the free ligand (L), the band of 3365 cm−1 was observed and assigned to ν (O–H) absorption. The strong band at 1608 cm−1 was related to stretching vibrations of C=N functional group, which was confirmed by the presence of Schiff base. C=C was assigned at 1545 cm−1 [Citation38]. Copper nanocomplex, ν C=N (1608 cm−1) was shifted to 1596 cm−1 (lower frequencies), when complex was obtained [Citation39]. The small bands at 495 and 522 cm−1 are related to Cu–N and Cu–O bonds in nanocomplex. FT-IR spectrum of Mn3O4/CuL bio-nanocolloid exhibits one strong band at 507 cm−1, which can be attributed to the vibrations of metal-oxygen confirming the presence of Mn3O4 NPs [Citation40].
The presences of all elements in Mn3O4/CuL bio-nanocolloid were confirmed at EDAX of it and elemental analysis of this compound (). Copper, manganese, carbon and oxygen were observed as elements in this nanocolloid. 34.49% of C, 52.45% of O, 12.86% of Mn and 0.19% of Cu were in this material. The morphology, size, and shape of this bio-material were investigated by TEM and SEM (). Aggregation of particles was observed as TEM image. SEM image shows nanocube morphology of prepared nanocolloid, with width of 80 nm in diameter and 3 mm in length.
Catalytic activities of Mn3O4/CuL bio-nanocolloid on the oxidation primary and secondary alcohols
The catalytic activity of Mn3O4/CuL bio-nanocolloid was investigated on the oxidation of alcohols (Scheme 2). The catalyst-free condition does not have the ability to oxidize the benzyl alcohol under different conditions. In this regards, catalytic experiments were initiated with the oxidation of benzyl alcohol (1 mmol) under air in the presence of H2O2 to have the standard conditions of the reaction. In this hence, the nature of solvent and oxidant, temperature, reaction time and the quantity of catalyst were considered on the oxidation of benzyl alcohol as a blank reaction in this green system ().
Scheme 2. The oxidation of primary and secondary alcohols catalysed by Mn3O4/CuL bio-nanocolloid using H2O2 to have O2 and water as byproducts under ultrasonic irradiation.
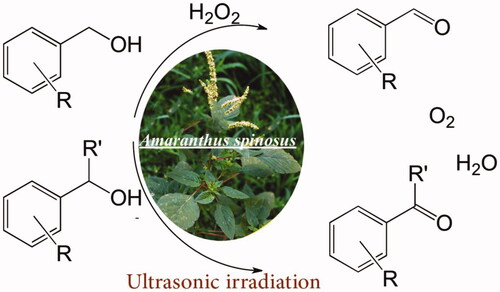
About 0.1 mmol concentration of alcohol and 80 °C are the best conditions for this oxidation reaction as the further evaluation demonstrated. The green oxidation of benzaldehyde was completed after 5 h (). Also, a survey of the results shown in revealed that a sufficient amount of catalyst loading of 0.007 g in the presence of 0.4 mmol oxidant led to higher conversion, but yield of product decreased with higher concentrations of catalyst. The effect of solvent on the catalytic performance is shown in . The reaction did not proceed in aprotic and protic solvents such as ethanol (EtOH), methanol (MeOH), acetonitrile (CH3CN), water and n-hexane. While, the solvent-free condition under air at 80 C, benzyl alcohol was converted to benzaldehyde completely. So, solventless was selected as a safe and green media for industrial applications. Based on the data in , common oxidants such as O2, TBAO (tetrabutyl ammonium oxone) and Oxone@ were weaker oxidants than H2O2 and TBHP for this transformation. However, H2O2 was selected as an ideal oxidant for further investigation. The ability synthesized Mn3O4, manganese salt, ligand (L) and CuL nanocomplex to promote the oxidation of benzyl alcohol which was then probed under the obtained optimized condition (). These compounds exhibited poor activity under this condition and the preferable catalytic performance of Mn3O4/CuL bio-nanocolloid was confirmed.
As described in documents [Citation41], Lewis acid sites were believed as the active sites in catalytic oxidation of benzyl alcohol with H2O2 [Citation42]. In this case, aqueous phase of H2O2 (30%) and benzyl alcohol from alcohol phase may interact with the catalyst on the interphase to generate the product benzaldehyde. This species attacks benzyl alcohol to form peroxycarboximidic acid intermediate (), which is then converted into benzyl alcohol with concomitant regeneration of the catalyst. In view of the proposed mechanistic pathways for the catalytic oxidation of benzyl alcohol, it can be concluded that both the phenyl ring and OH group of benzyl alcohol may interact with the metal centre of Cu, whereas the inner active sites remain intact. However, the interaction of the phenyl ring and OH group with the outer metal ions of Cu is also possible via adsorption of the phenyl ring on Cu, as reported in reference [Citation43].
Figure 6. Proposed mechanism for the catalytic oxidation of benzyl alcohol by Mn3O4/CuL bio-nanocolloid.
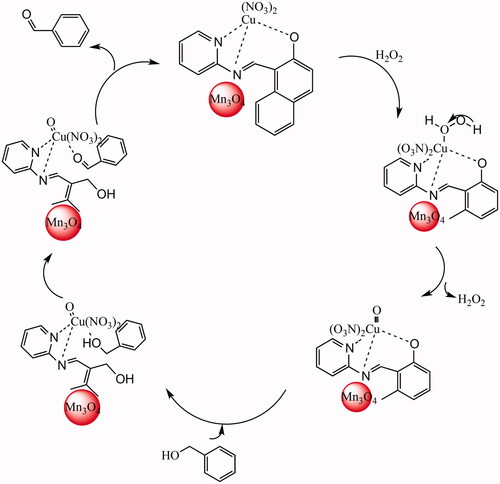
Thereafter, we focussed on the consideration of the substrate scope under stirring. In this regards, a set of structurally and electronically primary and secondary alcohols were selected for this safe and cost-efficient strategy (). As shown in , good to excellent yields were obtained for different primary and secondary benzyl alcohols, except for those with the aryl ring substituted with a nitro group. It should be noted that the corresponding carboxylic acids and ester were not observed in this green system and over-oxidation did not happen.
Table 1. Oxidation of alcohols catalysed by Mn3O4/CuL bio-nanocolloid using hydrogen peroxide.
Finally, we compared the conditions of oxidation of benzyl alcohol in this clean and cheap system with the results of other reports using hydrogen peroxide in different conditions (). The results showed that this Mn3O4/CuL bio-nanocolloid bionanocomposite could be used as a robust, reusable and active heterogeneous catalyst in the oxidation of primary and secondary alcohols with H2O2.
Table 2. Comparison of the result of this study with that of related references.
Reusability of catalyst
Recovery and the catalytic activity are imperative options for the industrial application of this biocatalyst. For this aim, the structure of catalyst must be unchanged. A comparison of the FT-IR spectra of the used Mn3O4/CuL bio nanocolloid (six recovery cycles) with those of the fresh catalyst showed that the structure of the catalyst remained almost completely intact (). On the other hand, the reusability of the Mn3O4/CuL bio nanocolloid was explored in the oxidation of benzyl alcohol. When the reactions were completed, the nanocolloid was separated by centrifugation after addition of the ethyl acetate. Recovered catalyst in dry state was used for the next runs. Stability as well as the activity of it was investigated and Mn3O4/CuL bionanocolloid was recycled for six times. Remarkable results (100–90%) of benzaldehyde were gained showing excellent catalytic activity of nanocolloid without noticeable loss ().
Figure 7. (a) The FT-IR of fresh catalyst (top) and reused catalyst after six times (down) and (b) recycling of catalyst.
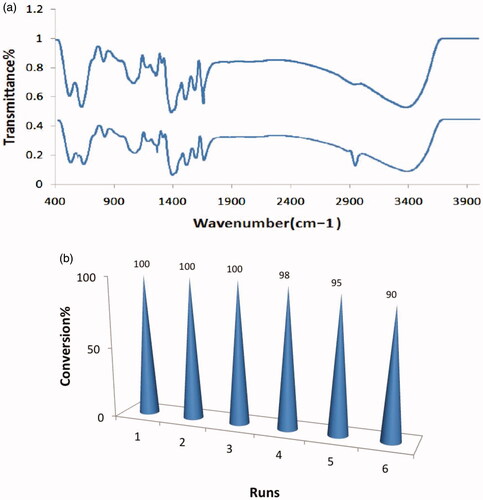
The features of our clean strategy on recycling of catalyst are the easy work up and convenient separation of product using green solvent (ethyl acetate or ethanol) from reactions. This method is not only eco-friendly but also less time consuming, cost effective, stable operation, efficient and safer. On the other hand, natural starting material for catalyst preparation, a long-term stability of catalyst, using ideal oxidant, and solventless show the great potential in scalability for actual industries applications. These features as concepts in economical and sustainable modern oxidation systems along with good reusability of the bio-catalyst render a practical strategy to address the environmental and industrial applications.
Conclusions
In summary, the first bio-nanocomposite based on weeds was synthesized. We prepared a novel of bionanocolloid successfully, by incorporating copper nanocomplex as a biocompatible molecule with Mn3O4 nanoparticles under ultrasonic agitation in the presence of Amaranthus spinosus. As a heterogeneous catalyst, the Mn3O4/CuL nanocatalyst efficiently oxidized a wide range of primary and secondary alcohols to the corresponding carbonyl compounds using H2O2 and under solvent free conditions as ideal oxidant and reaction media, respectively. Using cheap and natural starting materials, reusability, and recyclability of the catalyst are salient features of this green strategy from an economic and environmental point of view.
Disclosure statement
The authors declare that they have no known competing financial interests or personal relationships that could have appeared to influence the work reported in this paper.
Additional information
Funding
References
- Codou A, Guigo N, Heux L, et al. Partial periodate oxidation and thermal cross-linking for the processing of thermoset all-cellulose composites. Compos Sci Technol. 2015;117:54–61.
- Hassan HB, Tammam RH. Preparation of Ni-metal oxide nanocomposites and their role in enhancing the electro-catalytic activity towards methanol and ethanol. Solid State Ionics. 2018;320:325–338.
- Hatami M. Production of polyimide ceria nanocomposites by development of molecular hook technology in nano-sonochemistry. Ultrason Sonochem. 2018;44:261–271.
- Maleki A, Jafari AA, Yousefi S. Green cellulose-based nanocomposite catalyst: design and facile performance in aqueous synthesis of pyranopyrimidines and pyrazolopyranopyrimidines. Carbohydr Polym. 2017;175:409–416.
- Aygün A, Özdemir S, Gülcan M, et al. Synthesis and characterization of reishi mushroom-mediated green synthesis of silver nanoparticles for the biochemical applications. J Pharm Biomed Anal. 2020;178:112970.
- Cheng Y, Wei Z, Du Q, et al. The shape effect manganese (II,III) oxide nanoparticles on the performance of electrochemical capacitors. Elactrochim Acta. 2018;284:408–417.
- Mohammadiyan E, Ghafuri H, Kakanejadifard A. Synthesis and a magnetic Fe3O4 @CeO2 nanocomposite decorated with Ag nanoparticle and investigation of synergistic effects of Ag on photocatalytic activity. Optik. 2018;166:39–48.
- RabiulAwual M, Khraisheh M, Alharthi N, et al. Efficient detection and adsorption of cadmium(II) ions using innovative nano-composite materials. Chem Eng J. 2018;343:118–127.
- Saad R, AboElazayem O, Kellici S, et al. Greener synthesis of dimethyl carbonate using a novel tin-zirconia/grapheme nanocomposite catalyst. Appl Catal B: Environ. 2018;226:451–462.
- Şahin B, Aygün A, Gündüz H, et al. Cytotoxic effects of platinum nanoparticles obtained from pomegranate extract by the green synthesis method on the MCF-7 cell line. Colloids Surf B Biointerfaces. 2018;163:119–124.
- Yuan Y, Yu B, Shi Y, et al. Highly efficient catalysts for reducing toxic gases generation change with temperature of rigid polyurethane foam nanocomposites: a comparative investigation. Compos A Appl Sci Manuf. 2018;112:142–154.
- Chemat F, Rombaut N, Sicaire A-G, et al. Ultrasound assisted extraction of food and natural products. Mechanisms, techniques, combinations, protocols and applications. A review. Ultrason Sonochem. 2017;34:540–560.
- Liu C, Li Y, Zhang Y, et al. Synthesis of Ni-CeO2 nanocatalyst by the microemulsion-gas method in a rotor-stator reactor. Chem Eng Process. 2018;130:93–100.
- Lumina Sonia M, Anand S, Blessi S, et al. Effect of surfactants (PVB/EDTA/CTAB) assisted sol–gel synthesis on structural, magnetic and dielectric properties of NiFe2O4 nanoparticles. Ceram Int. 2018;44:272–842.
- Al-Asfar A, Zaheer Z, Aazam ES. Ecofriendly green synthesis of Ag@febimetallic nanoparticles: antioxidant, antimicrobial and photocatalytic degradation of bromothymol blue. J Photochem Photobiol B. 2018;185:143–152.
- Bhattarai B, Zaker Y, Bigioni TP. Green synthesis of gold and silver nanoparticles: challenges and opportunities. Curr Opin Green Sustain Chem. 2018;12:91–100.
- Şahin B, Demir E, Aygün A, et al. Investigation of the effect of pomegranate extract and monodisperse silver nanoparticle combination on MCF-7 cell line. J Biotechnol. 2017;260:79–83.
- Aygun A, Gülbagca F, Yildiz L, et al. Biogenic platinum nanoparticles using black cumin seed and their potential usage as antimicrobial and anticancer agent. J Pharm Biomed Anal. 2020;179:112961.
- Naeimi A, Amiri A, Ghasemi Z. A novel strategy for Green synthesis of colloidal porphyrins/silver nanocomposites by Sesbania sesban plant and their catalytic application in the clean oxidation of alcohols. J Taiwan Inst Chem Eng. 2017;80:107–113.
- Nagar N, Devra V. Green synthesis and characterization of copper nanoparticles using Azadirachta indica leaves. Mater Chem Phys. 2018;213:44–51.
- Rivera-Rangel RD, González-Muñoz MP, Rodriguez MA, et al. Green synthesis of silver nanoparticles in oil-in-water microemulsion and nano-emulsion using geranium leaf aqueous extract as a reducing agent. Colloids Surf A Physicochem Eng Aspects. 2018;536:60–67.
- Saha B, Yadav SK, Sengupta S. Synthesis of nano-Hap prepared through green route and its application in oxidative desulfurization. Fuel. 2018;222:743–752.
- Kaur M, Kumar M, Sachdeva S, et al. Aquatic weeds as the next generation feedstock for sustainable bioenergy production. Bioresour Technol. 2018;251:390–402.
- Boreh AJ, Agarwal M, Goyal A, et al. Physical insights of ultrasound-assisted ethanol production from composite feedstock of invasive weeds. Ultrason Sonochem. 2019;51:378–385.
- Shanker U, Jassal V, Rani M. Green synthesis of iron hexacyanoferrate nanoparticles: potential candidate for the degradation of toxic PAHs. J Environ Chem Eng. 2017;5(4):4108–4120.
- Chemat F, Khan ZHMK. Applications of ultrasound in food technology: processing, preservation and extraction. Ultrason Sonochem. 2011;18:813–835.
- Zhao ZX, Cheng LP, Pang W. Green synthesis of ethyl oxalate benzylidinylhydrazides. Tetrahedron Lett. 2018;59(21):2079–2081.
- Nikbakht E, Yadollahi B, Farsani MR. Green oxidation of alcohols in water by a polyoxometalate nanocapsule as catalyst. Inorg Chem Commun. 2015;55:135–138.
- Caron S, Dugger RW, Ruggeri SG, et al. Large scale oxidation in pharmaceutical industry. Chem Rev. 2006;106(7):2943–2989.
- Tian C, Chu G, Feng Y, et al. Quantitatively identify and understand the interphase of SiO2/rubber nanocomposites by using nanomechanical mapping technique of AFM. Compos Sci Technol. 2019;170:1–6.
- Papiya F, Pattanayak P, Kumar P, et al. Development of highly efficient bimetallic nanocomposite cathode catalyst, composed of Ni:Co supported sulfonated polyaniline for application in microbial fuel cells. Electrochim Acta. 2018;20:931–945.
- Selvarajan S, Suganthi A, Rajarajan M. A facile synthesis of ZnO/manganese hexacyanoferrate nanocomposite modified electrode for the electrocatalytic sensing of riboflavin. J Phys Chem Solids. 2018;121:350–359.
- Dasgupta S, Chakraborty A, Chatterjee S, et al. Synthesis and characterization of magnetically separable Fe3O4@AHBA@Ni(O) [AHBA = 3-amino-4-hydroxybenzoicacid] nanocatalyst: applications for carbonyl hydrogenation and alcohol oxidation. Inorg Chim Acta. 2018;474:1–10.
- Duman S, Ozkar S. Ceria supported manganese(O) nanoparticle catalysts for hydrogen generation from the hydrolysis of sodium borohydride. Int J Hydrogen Energy. 2018;43(32):15262–15274.
- Ali L, Mathlouthi E, Kajdan M, et al. Multifunctional manganese-doped Prussian blue nanoparticles for two photon photothermal therapy and magnetic. Catal Commun. 2018;8:2037–2042.
- Augustin M, Fenske D, Bardenhagen I, et al. Manganese oxide phases and morphologies: a study on calcination temperature and atmospheric dependence. Beilstein J Nanotechnol. 2015;6:47–59.
- Lee JH, Sa YJ, Kim TK, et al. A transformative route to nanoporous manganese oxides of controlled oxidation states with identical textural properties. J Mater Chem A. 2014;2(27):10435–10443.
- Naeimi A, Honarmand M, Sedri A. Ultrasonic assisted fabrication of first MoO3/copper complex bio-nanocomposite based on Sesbania sesban plant for green oxidation of alcohols. Ultrason Sonochem. 2019;50:331–338.
- Sedri A, Naeimi A, Mohammadi SZ. An innovative synthesis of MoO3/Ag nanocomposite and catalytic application of immobilized molybdenum complex on cellulose extracting from Carthamus tinctorius. Carbohydr Polym. 2018;199:236–243.
- Musić MRS, Popović S, Dragčević Đ, et al. Synthesis and long-term phase stability of Mn3O4 nanoparticles. J Mol Struct. 2013;1044:255–261.
- Endud S, Wong KL. Mesoporous silica MCM-48 molecular sieve modified with SnCl2 in alkaline medium for selective oxidation of alcohol. Micropor Mesopor Mater. 2007;101(1–2):256–263.
- Chaudhari MP, Sawant SB. Kinetics of heterogeneous oxidation of benzyl alcohol with hydrogen peroxide. Chem Eng J. 2005;106(2):111–118.
- Ma CY, Cheng J, Wang HL, et al. Characteristics of Au/HMS catalysts for selective oxidation of benzyl alcohol to benzaldehyde. Catal Today. 2010;158(3–4):246–251.
- Dong X, Wang D, Li K, et al. Vanadium-substituted heteropolyacids immobilized on amine-functionalized mesoporous MCM 41: are cyclable catalyst for selective oxidation of alcohols with H2O2. Mater Res Bull. 2014;57:210–220.
- Venturello C, Gambaro M. Selective oxidation of alcohols and aldehydes with hydrogen peroxide catalyzed by methyltrioctylammonium tetrakis(oxodiperoxotungsto)phosphate(3-) under two-phase conditions. J Org Chem. 1991;56(20):5924–5931.
- Vasylyev MV, Neumann R. New heterogeneous polyoxometalate based mesoporous catalysts for hydrogen peroxide mediated oxidation reactions. J Am Chem Soc. 2004;126(3):884–890.
- Lang X, Li Z, Xia C. [α-PW12O40]3− immobilized on ionic liquid–modified polymer as a heterogeneous catalyst for alcohol oxidation with hydrogen peroxide. Synth Commun. 2008;38:1620–1616.
- Karimi B, Rostami FB, Khorasani M, et al. Selective oxidation of alcohols with hydrogen peroxide catalyzed by tungstate ions (WO4=) supported on periodic mesoporous organosilica with imidazolium frameworks (PMO-IL). Tetrahedron. 2014;70(36):6114–6119.