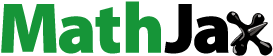
Abstract
Background
Protocatechuic aldehyde (PA) extracts from S. miltiorrhiza, which anti-oxidative and anti-inflammatory functions have been certified in diverse diseases. Nonetheless, the influence of PA in spinal cord injury (SCI) is still hazy. The research probed the function of PA in hydrogen peroxide (H2O2)-damaged PC12 cells.
Methods
The disparate dosages of H2O2 (0–400 µM) or PA (0–2 µM) were applied for stimulating PC12 cells, and subsequently cell viability, apoptosis, apoptosis- and autophagy-correlative factors were evaluated. After pc-MEG3 transfection, functions of MEG3 overexpression in H2O2 and/or PA-managed PC12 cells were reassessed. Western blot was conducted to determine Wnt/β-catenin and PTEN/PI3K/AKT pathways.
Results
H2O2 stimulation clearly triggered PC12 cell damage via prohibiting cell viability and accelerating apoptosis and autophagy. But, PA management mitigated H2O2-triggered PC12 cells damage. Down-regulated MEG3 triggered by PA was presented in H2O2-managed cells. What’s more, overexpressed MEG3 dramatically overturned the influences of PA in H2O2-damaged PC12 cells. Beyond that, PA activated Wnt/β-catenin and PTEN/PI3K/AKT via repression of MEG3 in H2O2-managed PC12 cells.
Conclusions
The results disclosed the protective impacts of PA on PC12 cells to resist H2O2-provoked damage. MEG3, Wnt/β-catenin and PTEN/PI3K/AKT pathways joined in adjusting the activity of PA in H2O2-damaged PC12 cells.
Introduction
Spinal cord injury (SCI) is a sort of familiar trauma, which is given rise to spinal cord lateral bending, overextension, rotation and axial load due to external forces [Citation1,Citation2]. SCI is able to cause fearful dysfunction of lower limbs, which may involve respiratory and urinary systems, and even endanger life [Citation3]. SCI is accompanied by extensive complications, such as pressure sore, urinary tract infection, spasm, paraplegia, neuralgia and pneumonia [Citation4–7]. At present, the principle of treatment for SCI is executed drugs and hyperbaric oxygen on the basis of spinal decompression [Citation8]. The therapeutic strategies may affect rehabilitation care and functional recovery of the patients with SCI. However, SCI has a poor prognosis invariably, which often leaves behind different degrees of sequelae, and requires long-term rehabilitation treatment. Hence, further in-depth research on the molecular mechanism of SCI may offer a theoretical basis for better seeking the neoteric treatment methods for SCI.
Protocatechuic aldehyde (PA) is a water-soluble traditional Chinese medicine (TCM) monomer, which extracts from the root of Salvia miltiorrhiza Bunge (S. miltiorrhiza) [Citation9]. PA has been corroborated to join in affecting vascular smooth muscle cell migration, proliferation and intravascular thrombosis [Citation10]. Moreover, one research from Xing et al. declared that PA could restrain lipopolysaccharide (LPS)-triggered human umbilical vein endothelial cell apoptosis through mediation of Caspase-3 [Citation11]. Additionally, the protective function of PA in SH-SY5Y cells against oxidative stress was testified by Gao et al. [Citation12]. Further, the anti-inflammatory impact of PA on myocardial ischaemia/reperfusion (MI/R) injury was revealed in vivo and in vitro experiments [Citation13]. In line with these researches, we understood that PA might exhibit the protective activity in diverse diseases. Nonetheless, whether PA exerts the functions in SCI is still ambiguous.
Hereof, we attempted to investigate the regulatory mechanism of PA in SCI. Hydrogen peroxide (H2O2) was utilized for the establishment of PC12 cell injury model. Subsequently, the roles of PA in PC12 cells impaired by H2O2 were probed. In this process, whether maternally expressed gene 3 (MEG3) participates in mediating the functions of PA is also investigated. The possible regulatory mechanism was disclosed through monitoring Wnt/β-catenin and PTEN/PI3K/AKT pathways. This research showcased the protective function of PA in H2O2-caused PC12 cells damage, which hinted that PA might be a potent therapeutic drug for remedying SCI.
Materials and methods
Cell cultivation and stimulation
PC12 cells purchased from American Type Culture Collection (ATCC, Rockville, MD, USA) were cultured in the recommended RPMI-1640 medium (ATCC® 30-2001™). For configuration of the complete medium, RPMI-1640 medium was mixed with 10% horse serum and 5% FBS (ATCC). An incubator setting at 37 °C with 5% CO2 and 95% air was exploited to culture PC12 cells. The reagent of 3% H2O2 was obtained from Millipore (Billerica, MA), which was configured as the diverse dosages (50, 100, 200, 300 and 400 µM) for the disposition of PC12 cells for 24 h. PA purchased from Meilun Biology Technology (Dalian, China) was configured to diverse concentrations as 0.1, 0.5, 1 and 2 µM. Subsequently, PA was exploited to pre-process PC12 cells before receiving H2O2 management. After 24 h treatment, these managed PC12 cells were gathered and applied to the follow-up experiments.
Cell transfection
The whole-length MEG3 sequence was inserted into pcDNA3.1 vector to establish the overexpressed vector of MEG3 (pc-MEG3). Meanwhile, the empty pcDNA3.1 was utilized as a negative control in the correlative experiment. These expression vectors were composited by Sangon (Shanghai, China), simultaneously these composited vectors were employed for transient transfection via conducting the Lipofectamine 3000 (Invitrogen, Carlsbad, CA, USA). The cell transfection was conducted for 48 h, and subsequently, cells were collected for the next researches.
Cell viability assay
The vectors of pc-MEG3 and pcDNA3.1 were capitalized for cell transfection, and these transfected cells were received H2O2 and/or PA management. Cell Counting Kit-8 (CCK-8, APExBIO, Houston, TX, USA) was used to monitor cell viability. These above-involved cells were cultivated with 10 µL CCK-8 solution for 1 h, and subsequently, a Microplate Reader instrument (Dynatech, Chantilly, VA, USA) was manipulated to detect the absorbance at 450 nm.
Cell apoptosis assay
After pc-MEG3 and pcDNA3.1 transfection, these cells were received H2O2 and/or PA stimulation. After this, PC12 cells were gathered and laundered with PBS, as well as re-suspended in 250 µL 1× binding buffer. Afterwards, 100 µL cell suspensions were supplemented into the 5 ml flow tubes; meanwhile, they were dyed with 5 µL Annexin V-FITC (Solarbio, Beijing, China) and 10 µL PI (Solarbio) for 15 min incubation under the darkness circumstances. Subsequently, the flow tubes were added 400 µL PBS, and cell apoptosis was immediately analysed by utilizing a flow cytometry (FACSAria II, BD, USA).
Real-time quantitative PCR (RT-qPCR)
After transfection with pc-MEG3/pcDNA3.1 and stimulation with H2O2/PA, total RNA from these cells was elicited via applying TRIzol reagent (TaKaRa, Dalian, China). HiFi-MMLV cDNA kit (Cwbio, Beijing, China) was utilized for the composition of cDNA conforming to the specification. Expression level of MEG3 was determined via exploiting SYBR® Green PCR Kit (Takara), and RT-qPCR experiment was conducted on ABI Prism 7500 Real-Time PCR instrument (ABI, Foster, CA, USA). Primers sequences employed in RT-qPCR were listed in the following: MEG3, Forward, 5′-AGA GAG GAC ATG AGG ACC CC-3′; Reverse, 5′-CCT ACC TCA CAG GGC TGT TG-3′. β-actin, Forward, 5′-ACA TCT GCT GGA AGG TGG AC-3′; Reverse 5′-GGT ACC ACC ATG TAC CCA GG-3′. For the standardization of MEG3, β-actin was chosen as the internal reference. Method of analysis was applied for analysing the correlative data in the experiment [Citation14].
Western blot assay
After pc-MEG3 and pcDNA3.1 transfection, PC12 cells received H2O2 and/or PA stimulation. Total protein from above-managed cells was elicited in RIPA lysis buffer (Beyotime), which contained the correlative protease inhibitor (Upstate Group, Lake Placid, NY). Modified BCA kit (Sangon) was utilized to determine the protein concentrations. The SDS-PAGE was then conducted to separate protein samples, and these separated proteins were shifted to PVDF membranes (Invitrogen) on the basis of wet transfer method. After this, the membranes were sealed with 5% BSA for 1 h at 37 °C, and then were co-cultivated with the related primary antibodies of Bax (#ab182733), Pro-Caspase-3 (#ab32150), Cleaved-Caspase-3 (#ab49822), Caspase-9 (#ab32539), Beclin-1 (#ab210498), p62 (#ab109012), LC3-II (#ab51520), Wnt3a (#ab28472), β-catenin (#ab32572), Wnt5a (#ab174963), PTEN (#ab170941) and β-actin (#ab227387, Abcam, Cambridge, UK), and t-PI3K (#4292), p-PI3K (#4228), t-AKT (#4691), p-AKT (#4060, CST, Boston, MA, USA) for all night at 4 °C. After co-cultivation with the corresponding secondary antibody (#ab205718, Abcam) for 1 h, and subsequently, the enhanced chemiluminescence (ECL) reagent (Pierce) was then exploited for the acquisition of the western blots, and the signals were analysed via application of ImageJ software (NIH, Bethesda, MD, USA).
Statistical analysis
The data in this research were calculated via adopting SPSS 19.0 (IBM, Armonk, NY), and were depicted as mean ± SD. The Student’s t-test was utilized to analyse the data from two groups, as well as ANOVA with Tukey’s post-hoc test was employed to analyse the data from multiple subgroups. p-value < .05 was deemed as a significantly different verdict.
Results
H2O2 evoked PC12 cells damage
The diverse dosages of H2O2 (0–400 µM) were exploited to deal with PC12 cells, and cell viability was subsequently detected through CCK-8 assay. The vigour of PC12 cells was clearly crippled by H2O2 at the dosages of 50, 100 (p < .05), 200, 300 (p < .01) and 400 µM (p < .001, ). In consideration of cell viability was half-suppressed by 200 µM H2O2, the subsequent trials were utilized this dosage to stimulate PC12 cells. The next trial revealed that cell apoptosis was distinctly expedited by H2O2 (p < .001, ). In the meantime, the expression levels of apoptosis-relevant factors (Bax, and Cleaved-Caspase-3/-9) were all upgraded by H2O2 (p < .001, ). Data from these trials testified that H2O2 stimulation resoundingly triggered PC12 cells damage.
Figure 1. H2O2 caused PC12 cells damage. (A) PC12 cells were received the diverse dosages of H2O2 (0–400 µM) management, CCK-8 experiment was conducted to detect cell viability. The dosage of 200 µM H2O2 was selected and utilized to manage PC12 cells. CCK-8, flow cytometry and western blot experiments were implemented to examine (B) cell viability, (C) apoptosis and (D,E) Bax, Pro/Cleaved-Caspase-3 and Pro/Cleaved-Caspase-9 expression. *p < .05, **p < .01, ***p < .001.
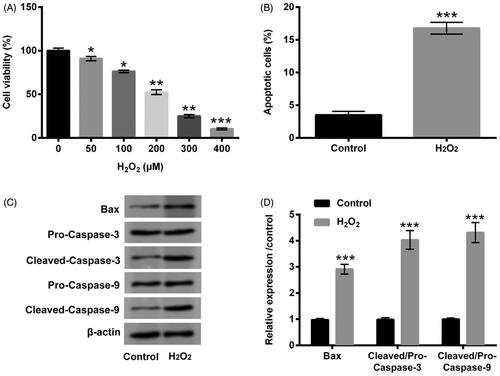
PA eased H2O2-triggered PC12 cell damage
The disparate dosages of PA (0–2 µM) were then applied to manage PC12 cells, meanwhile we discovered the suppressed cell viability (p < .05) was appeared in PA-disposed group at the dosage of 2 µM (). Interestingly, there was no appreciable influence in cell viability in PA-managed group at the dosages of 0.1, 0.5 and 1 µM (). Hence, 1 µM PA was elected for the stimulation of PC12 cells in the follow-up experiments. We then observed that H2O2-crippled cell viability was obviously relieved by PA management (0.5 µM, p < .05; 1 µM p < .01; ). Additionally, H2O2-accelerated cell apoptosis was also eased by PA management (p < .01, ). The up-regulations of Bax and Cleaved-Caspase-3/-9 evoked by H2O2 were likewise declined by PA administration (p < .01, ). After H2O2 and PA stimulation, the protein levels of cell autophagy-correlated factors (Beclin-1, p62 and LC3-II/LC3-I) were then probed in PC12 cells. In , we discovered that H2O2 stimulation enhanced Beclin-1 and LC3-II expression (p < .001), however, abated p62 expression (p < .01). Nevertheless, PA administration clearly overturned H2O2-affected the expression of cell autophagy-correlated factors (p < .01, ). Data from above trials attested that PA could assuage H2O2-triggered PC12 cell damage.
Figure 2. PA assuaged H2O2-triggered PC12 cell damage. (A) PC12 cells received the different dosages of PA (0–2 µM) administration, CCK-8 experiment was executed to test cell viability. (B) H2O2 (200 µM) and PA (0.1, 0.5 and 1 µM) were exploited to co-manage PC12 cells, CCK-8 experiment was carried out again to examine cell viability. H2O2 (200 µM) and PA (1 µM) were utilized to dispose PC12 cells, flow cytometry and western blot were conducted to evaluate (C) apoptosis and (D,E) Bax, Pro/Cleaved-Caspase-3 and Pro/Cleaved-Caspase-9 expression. *p < .05, **p < .01, ***p < .001.
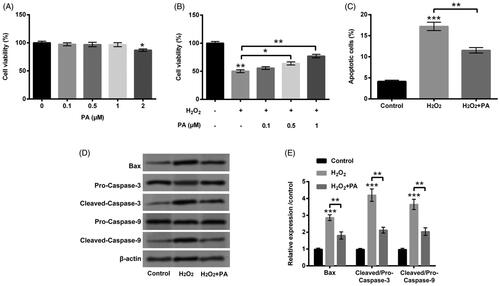
PA repressed MEG3 expression in H2O2-damaged PC12 cells
We subsequently explored the correlation between PA and MEG3 in H2O2-impaired PC12 cells. Primordially, we only capitalized PA to stimulate PC12 cells, and the consequence revealed that MEG3 expression was reduced by PA management alone (p < .05, ). Intriguingly, in H2O2-managed PC12 cells, the elevation of MEG3 expression (p < .01) was discovered (). But, the above phenomenon was visibly abrogated by PA administration (p < .01, ). For purpose of whether MEG3 takes part in adjusting the influence of PA in H2O2-impaired PC12 cells, pc-MEG3 vector was utilized for the alteration of MEG3 expression. After executing cell transfection, the raised MEG3 expression was discovered in pc-MEG3-transfected PC12 cells (p < .01, ). These successfully transfected PC12 cells were then employed in the subsequent experiments.
Figure 4. PA caused MEG3 down-regulation in H2O2-damaged PC12 cells. (A) PA (1 µM) was utilized to manage PC12 cells, RT-qPCR was performed to detect MEG3 expression in these disposed PC12 cells. (B) H2O2 (200 µM) and PA (1 µM) were exploited to manage PC12 cells, RT-qPCR was conducted to determine MEG3 expression in these disposed PC12 cells. (C) Expression vectors of pc-MEG3 and pcDNA3.1 were applied for PC12 cells transient transfection, and then RT-qPCR was performed again to determine MEG3 expression in these transfected PC12 cells. *p < .05, **p < .01.
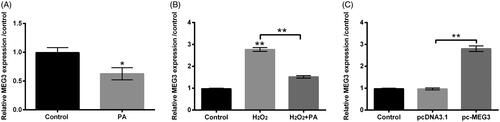
PA assuaged H2O2-triggered PC12 cells damage through declining MEG3 expression
After overexpression of MEG3, the involvement in PA-adjusted H2O2-triggered PC12 cell damage was probed. We firstly discovered that overexpressed MEG3 restrained cell vitality (p < .05, Supplementary Figure S1(A)) as well as induced apoptosis in PC12 cells (p < .01, Supplementary Figure S1(B)). Nevertheless, PA accelerated cell viability, and restrained apoptosis in H2O2-treated PC12 cells were both reversed by overexpressed MEG3 (p < .05 or p < .01, ). On the side, PA declined apoptosis-associated factors levels in H2O2-managed cells were also reversed by overexpressed MEG3 (p < .01 or p < .001, ). Beyond that, PA repressed Beclin-1 and LC3-II expression meanwhile elevated p62 expression were all overturned by overexpressed MEG3 (p < .05 or p < .01, ). These results showcased that PA assuaged H2O2-triggered PC12 cell damage via suppression of MEG3 expression.
Figure 5. PA allayed H2O2-triggered PC12 cells damage through prohibiting MEG3 expression. PC12 cells received pc-MEG3 and pcDNA3.1 transfection and then were managed with H2O2 (200 µM) and PA (1 µM), CCK-8, flow cytometry and western blot were executed to test (A) cell viability, (B) apoptosis, (C,D) Bax, Pro/Cleaved-Caspase-3 and Pro/Cleaved-Caspase-9 expression and (E,F) cell autophagy-associated factors (Beclin-1, p62 and LC3-II/LC3-I) expression. *p < .05, **p < .01, ***p < .001.
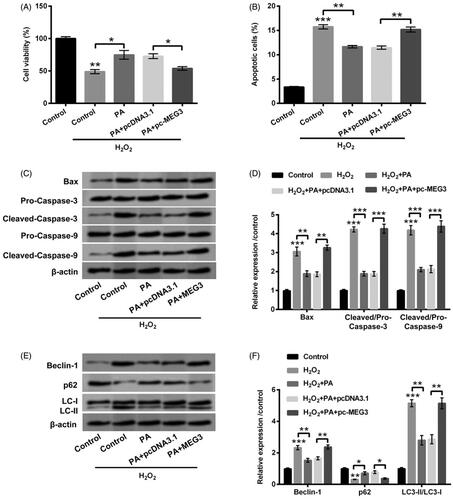
PA accelerated Wnt/β-catenin and PTEN/PI3K/AKT activations in H2O2-managed PC12 cells via decreasing MEG3 expression
Functions of PA in Wnt/β-catenin and PTEN/PI3K/AKT pathways were eventually delved in H2O2-managed PC12 cells. The decrease of Wnt3a, β-catenin and Wnt5a (all p < .01) were discovered in H2O2-managed PC12 cells (). But, PA obviously elevated Wnt3a, β-catenin and Wnt5a (all p < .01) in PC12 cells administrated by H2O2 (). After overexpression of MEG3, the enhanced Wnt3a, β-catenin and Wnt5a protein levels were crippled in H2O2-administrated PC12 cells (p < .01, ). In the meantime, H2O2 management enhanced PTEN expression (p < .001) as well as restrained p-PI3K (p < .01) and p-AKT (p < .05) expression (). Moreover, PA distinctly repressed PTEN expression (p < .01), meanwhile elevated p-PI3K and p-AKT (p < .05) expression in H2O2-managed PC12 cells (). Nonetheless, overexpressed MEG3 also overturned the mediatory functions of PA in PTEN (p < .01), p-PI3K and p-AKT expression (p < .05, ). Data from these trials represented that PA expedited Wnt/β-catenin and PTEN/PI3K/AKT activations in H2O2-disposed PC12 cells through mediation of MEG3.
Figure 6. PA expedited Wnt/β-catenin and PTEN/PI3K/AKT activations in H2O2-managed PC12 cells through adjusting MEG3 expression. PC12 cells were received pc-MEG3 and pcDNA3.1 transfection meanwhile were managed with H2O2 (200 µM) and PA (1 µM), western blot was conducted to estimate the protein levels of (A,B) Wnt3a, β-catenin and Wnt5a as well as (C,D) PTEN, p/t-PI3K and p/t-AKT in above-managed PC12 cells. *p < .05, **p < .01, ***p < .001.
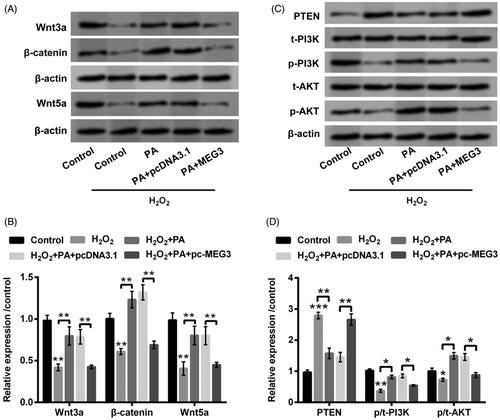
Discussion
Herein, the roles of PA in H2O2-triggered PC12 cell impairment were uncovered. The results showcased the protective ability of PA in PC12 cells against H2O2-evoked impairment, which was presented as accelerating cell viability, repressing apoptosis, meanwhile restraining autophagy-correlative factors expression. Additionally, PA stimulation triggered the decrease of MEG3 in H2O2-disposed PC12 cells. More importantly, MEG3 overexpression signally reversed the influences of PA in PC12 cell impairment evoked by H2O2. Despite these, the activations of Wnt/β-catenin and PTEN/PI3K/AKT were aggrandized by PA administration through prohibiting MEG3 expression in H2O2-managed PC12 cells.
TCMs have been shown the effective functions in remedying SCI, especially S. miltiorrhiza [Citation15,Citation16]. The existing evidences attest that S. miltiorrhiza emerges a beneficial role in impeding secondary damage after acute SCI, which also can fight cell apoptosis and accelerate nerve repair [Citation17,Citation18]. A rat SCI model experiment disclosed that the extractions from S. miltiorrhiza could effectively relieve SCI [Citation19]. As an important ingredient of S. miltiorrhiza, PA has exhibited the proverbially pharmacological activities in miscellaneous ailments [Citation20,Citation21]. A research disclosed that PA could reduce KIM1 expression at mRNA and protein levels, thereby allaying cisplatin-triggered renal failure [Citation22]. Moreover, this research also certified that PA could ease cisplatin-evoked cell oxidative damage and renal inflammation [Citation22]. But, whether PA could prevent H2O2-triggered PC12 cell damage and the latent mechanism are still uninvestigated. In consonance with the research from Guo et al. [Citation13] and the preliminary experiment, we chose the PA concentrations as 0.1, 0.5, 1 and 2 µM used in this research. We discovered that cell viability was clearly restrained by PA in a dose-dependent manner in PC12 cells. Moreover, PA assuaged H2O2-triggered PC12 cell damage through mediating cell behaviours of proliferation, apoptosis and autophagy. The aforesaid consequences imparted that PA might be a potent agent for the treatment of SCI.
MEG3 is situated at chromosome 14q32.3 in humans, which pertains to the imprinted DLK1-MEG3 locus [Citation23]. Function as a tumour suppressor, MEG3 has been broadly recognized in the existing researches [Citation24,Citation25]. Additionally, an interesting evidence uncovered that MEG3 might participate in controlling the angiopoiesis after SCI [Citation26]. Moreover, other attractive research corroborated that MEG3 might be responsible for the hypoxic-triggered damage in PC12 cells [Citation27]. Because of the research focuses on the functions of MEG3 in SCI is scanty, whether MEG3 joins in adjusting the protective ability of PA in H2O2-damaged PC12 cells is still necessary further explored. After H2O2 and/or PA management, the decrease of MEG3 evoked by PA was observed in H2O2-impaired PC12 cells. For further divulging the influence of MEG3 in PA protecting PC12 cells against H2O2-triggered injury, the overexpressed vector of MEG3 (pc-MEG3) was exploited to transient transfect into PC12 cells. The valuable consequences displayed that overexpressed MEG3 obviously overturned the protective activity of PA in H2O2-damaged PC12 cells, which implied that MEG3 might be a crucial participator in the process of PA protecting PC12 cells against H2O2-triggered cell damage.
Although the neuroprotective activity of PA has been testified in the existing researches [Citation28,Citation29], the regulatory mechanisms are distinct-different in the diverse ailments. In this research, we delved an innovative mediatory network in SCI, which was PA-MEG3 network. The extensive researches about lncRNA-associated ceRNA network (lncRNAs-miRNAs-mRNAs) have been discovered in diverse diseases including SCI. Dysregulations of lncRNAs have been testified to showcase a pivotal role in regulating inflammatory response (LINC00305 and LINC00341) and angiogenesis (HIF1A-AS2 and MEG3) [Citation30]. Abnormal expressed miRNAs, such as miR-21, miR-486, miR-20, were also uncovered to be embroiled in SCI through adjusting inflammation, cellular death, regeneration or gliosis [Citation31]. Moreover, several researches have also reported that MEG3 exerted its functions via targeting the relevant miRNAs or mRNA factors. Evidence revealed that MEG3 mediated ischaemic neuronal death through targeting miR-21/PDCD4 pathway [Citation32]. Moreover, up-regulated MEG3 participated in the protective activity of methylene blue in osteoarthritis via affecting P2X3 expression [Citation33]. Based on the above research foundation, we speculated that MEG3 might modulate the correlative downstream target miRNA or mRNA to affect the functions of PA in H2O2-damaged PC12 cells. Further researches are still necessary for certifying this hypothesis.
The involvement of Wnt/β-catenin pathway in SCI process has been extensively testified in the recent researches. One correlative literature represented that Wnt/β-catenin could expedite regeneration after SCI in adult zebrafish [Citation34]. Additionally, the activation of Wnt/β-catenin has been certified to take part in the mediation of the neuroprotective functions of rapamycin in SCI [Citation35]. PTEN/PI3K/AKT pathway has been also proven to participate in regulation of the progression of SCI. An animal model experiment revealed that PTEN/PI3K/AKT pathway was embroiled in adjusting the functional recovery and apoptosis in rat after SCI [Citation36]. Additionally, Zhang et al. discovered that PI3K/AKT pathway participated in adjusting SCI and the mechanism might be linked to apoptosis [Citation37]. In this research, we observed that the activations of Wnt/β-catenin and PTEN/PI3K/AKT pathways were both elevated after PA administration in H2O2-damaged PC12 cells. More interesting, the accelerative function was adjusted via repression of MEG3. These explorations evinced that PA exhibited the protective functions in PC12 cells to resist H2O2-triggered impairment might be adjusted by Wnt/β-catenin and PTEN/PI3K/AKT pathways.
Conclusion
In short, this research promulgated that PA expedited Wnt/β-catenin and PTEN/PI3K/AKT pathways via repression of MEG3, thereby exerting the protective action in H2O2-impaired PC12 cells. The above investigations hinted that PA might be a potent agent for the treatment of SCI. These findings will offer a reference for clinical research of PA. Further exploration of the functions of PA in SCI is still necessary.
Supplemental Material
Download MS Word (156.4 KB)Disclosure statement
No potential conflict of interest was reported by the authors.
References
- Burke D, Fullen BM, Stokes D, et al. Neuropathic pain prevalence following spinal cord injury: a systematic review and meta-analysis. Eur J Pain. 2017;21(1):29–44.
- Ruzicka J, Machova-Urdzikova L, Gillick J, et al. A comparative study of three different types of stem cells for treatment of rat spinal cord injury. Cell Transplant. 2017;26(4):585–603.
- Dudley-Javoroski S, Petrie MA, McHenry CL, et al. Bone architecture adaptations after spinal cord injury: impact of long-term vibration of a constrained lower limb. Osteoporos Int. 2016;27(3):1149–1160.
- Citak M, Suero EM, Backhaus M, et al. Risk factors for heterotopic ossification in patients with spinal cord injury: a case-control study of 264 patients. Spine. 2012;37(23):1953–1957.
- Blevins E, Raffini L. Extensive thrombotic complications after inferior vena cava filter placement in 2 adolescents with spinal cord injury: a cautionary tale and review. J Pediatr Hematol Oncol. 2015;37(4):e227–e229.
- Sadeghi Fazel F, Derakhshanrad N, Yekaninejad MS, et al. Predictive value of Braden risk factors in pressure ulcers of outpatients with spinal cord injury. Acta Med Iran. 2018;56(1):56–61.
- Motiei-Langroudi R, Sadeghian H. Traumatic spinal cord injury: long-term motor, sensory, and urinary outcomes. Asian Spine J. 2017;11(3):412–418.
- Ojo OA, Poluyi EO, Owolabi BS, et al. Surgical decompression for traumatic spinal cord injury in a tertiary center. Niger J Clin Pract. 2017;20(11):1455–1460.
- Byun JW, Hwang S, Kang CW, et al. Therapeutic effect of protocatechuic aldehyde in an in vitro model of Graves’ orbitopathy. Invest Ophthalmol Vis Sci. 2016;57(10):4055–4062.
- Moon CY, Ku CR, Cho YH, et al. Protocatechuic aldehyde inhibits migration and proliferation of vascular smooth muscle cells and intravascular thrombosis. Biochem Biophys Res Commun. 2012;423(1):116–121.
- Xing YL, Zhou Z, Agula , et al. Protocatechuic aldehyde inhibits lipopolysaccharide-induced human umbilical vein endothelial cell apoptosis via regulation of caspase-3. Phytother Res. 2012;26(9):1334–1341.
- Gao J-W, Yamane T, Maita H, et al. DJ-1-mediated protective effect of protocatechuic aldehyde against oxidative stress in SH-SY5Y cells. J Pharmacol Sci. 2011;115(1):36–44.
- Wei G, Guan Y, Yin Y, et al. Anti-inflammatory effect of protocatechuic aldehyde on myocardial ischemia/reperfusion injury in vivo and in vitro. Inflammation. 2013;36(3):592–602.
- Livak KJ, Schmittgen TD. Analysis of relative gene expression data using real-time quantitative PCR and the 2−ΔΔCT method. Methods. 2001;25(4):402–408.
- Zhang Q, Yang H, An J, et al. Therapeutic effects of traditional Chinese medicine on spinal cord injury: a promising supplementary treatment in future. Evid Based Complement Alternat Med. 2016;2016:8958721.
- Zhang J, Wei H, Lin M, et al. Curcumin protects against ischemic spinal cord injury: the pathway effect. Neural Regen Res. 2013;8(36):3391–3400.
- Koushki D, Latifi S, Norouzi Javidan A, et al. Efficacy of some non-conventional herbal medications (sulforaphane, tanshinone IIA, and tetramethylpyrazine) in inducing neuroprotection in comparison with interleukin-10 after spinal cord injury: a meta-analysis. J Spinal Cord Med. 2015;38(1):13–22.
- Li M, Wang J, Ding L, et al. Tanshinone IIA attenuates nerve transection injury associated with nerve regeneration promotion in rats. Neurosci Lett. 2017;659:18–25.
- Zhang Q, Liu X, Yan L, et al. Danshen extract (Salvia miltiorrhiza Bunge) attenuate spinal cord injury in a rat model: a metabolomic approach for the mechanism study. Phytomedicine. 2019;62:152966.
- Zhang L, Ji Y, Kang Z, et al. Protocatechuic aldehyde ameliorates experimental pulmonary fibrosis by modulating HMGB1/RAGE pathway. Toxicol Appl Pharmacol. 2015;283(1):50–56.
- Xiuli F, Yajun L, Jing L, et al. Protocatechuic aldehyde protects against isoproterenol-induced cardiac hypertrophy via inhibition of the JAK2/STAT3 signaling pathway. Naunyn Schmiedebergs Arch Pharmacol. 2018;391:1373.
- Gao L, Wu W-F, Dong L, et al. Protocatechuic aldehyde attenuates cisplatin-induced acute kidney injury by suppressing Nox-mediated oxidative stress and renal inflammation. Front Pharmacol. 2016;7:479.
- Wallace C, Smyth DJ, Maisuria-Armer M, et al. The imprinted DLK1-MEG3 gene region on chromosome 14q32.2 alters susceptibility to type 1 diabetes. Nat Genet. 2010;42(1):68–71.
- Zhou Y, Zhang X, Klibanski A. MEG3 noncoding RNA: a tumor suppressor. J Mol Endocrinol. 2012;48(3):R45–R53.
- Chak WP, Tong HM, Lung WM, et al. Abstract 600: maternally expressed gene 3 (MEG3) as a tumor suppressor in nasopharyngeal carcinoma. Cancer Res. 2014;74(19 Supplement):600.
- Shi Z, Pan B, Feng S. The emerging role of long non-coding RNA in spinal cord injury. J Cell Mol Med. 2018;22(4):2055–2061.
- Han L, Dong Z, Liu N, et al. Maternally expressed gene 3 (MEG3) enhances PC12 cell hypoxia injury by targeting MiR-147. Cell Physiol Biochem. 2017;43(6):2457–2469.
- Guo C, Wang S, Duan J, et al. Protocatechualdehyde protects against cerebral ischemia-reperfusion-induced oxidative injury via protein kinase Cε/Nrf2/HO-1 pathway. Mol Neurobiol. 2017;54(2):833–845.
- Zhao X, Zhai S, An M-S, et al. Neuroprotective effects of protocatechuic aldehyde against neurotoxin-induced cellular and animal models of Parkinson’s disease. PLoS One. 2013;8(10):e78220.
- Wang N, He L, Yang Y, et al. Integrated analysis of competing endogenous RNA (ceRNA) networks in subacute stage of spinal cord injury. 2020;726;144171.
- Nieto-Diaz M, Esteban FJ, Reigada D, et al. MicroRNA dysregulation in spinal cord injury: causes, consequences and therapeutics. Front Cell Neurosci. 2014;8:53–53.
- Yan H, Rao J, Yuan J, et al. Long non-coding RNA MEG3 functions as a competing endogenous RNA to regulate ischemic neuronal death by targeting miR-21/PDCD4 signaling pathway. Cell Death Dis. 2017;8(12):3211.
- Li X, Tang C, Wang J, et al. Methylene blue relieves the development of osteoarthritis by upregulating lncRNA MEG3. Exp Ther Med. 2018;15(4):3856–3864.
- Strand NS, Hoi KK, Phan TMT, et al. Wnt/beta-catenin signaling promotes regeneration after adult zebrafish spinal cord injury. Biochem Biophys Res Commun. 2016;477(4):952–956.
- Gao K, Wang Y-S, Yuan Y-J, et al. Neuroprotective effect of rapamycin on spinal cord injury via activation of the Wnt/β-catenin signaling pathway. Neural Regen Res. 2015;10(6):951–957.
- Zhu H, Xie R, Liu X, et al. MicroRNA-494 improves functional recovery and inhibits apoptosis by modulating PTEN/AKT/mTOR pathway in rats after spinal cord injury. Biomed Pharmacother. 2017;92:879–887.
- Zhang P, Zhang L, Zhu L, et al. The change tendency of PI3K/Akt pathway after spinal cord injury. Am J Transl Res. 2015;7(11):2223–2232.