Abstract
Background
Hypoxic-ischaemic encephalopathy (HIE) is a prevailing severe brain damage disease in newborns, and caused by perinatal asphyxia cerebral ischaemia and reperfusion. Here, we investigated the role of cZNF292 in oxygen-glucose deprivation/reperfusion (OGD/R)-induced neural stem cells (NSCs) injury, and explored the underlying molecular mechanism.
Methods
Before NSCs were subjected to OGD/R treatment, NSCs were transfected with or without overexpressing cZNF292, si-cZNF292 or miR-22 inhibitor. Viability, apoptosis and potential molecular mechanism were examined. Cell viability and apoptotic rate were evaluated utilizing cell counting kit-8 (CCK-8) and flow cytometry. The cZNF292 and miR-22 expression was determined utilizing quantitative reverse transcription-PCR (qRT-PCR). Moreover, apoptosis and Wnt/β-catenin and PKC/ERK pathways-associated proteins were quantified applying western blot.
Results
OGD/R repressed viability and promoted apoptosis of NSCs. Also, cZNF292 expression was promoted by OGD/R treatment. Moreover, cZNF292 overexpression further caused OGD/R-stimulated damage. Inversely, silencing cZNF292 alleviated OGD/R-stimulated damage in NSCs. In addition, miR-22 expression was negatively regulated by cZNF292. It was confirmed that silencing cZNF292 attenuated OGD/R-induced NSCs injury and promoted the activation of Wnt/β-catenin and PKC/ERK pathways via the up-regulation of miR-22.
Conclusions
The cZNF292 silence alleviated OGD/R-induced injury through the up-regulation of miR-22 in NSCs, and which furnished the theoretical basis for further research on HIE progression.
Introduction
Hypoxic-ischaemic encephalopathy (HIE) may take place before or during childbirth. Neurological injuries associated with HIE will bring serious consequences, such as acute death and long-term disability (like blindness, epilepsy and cerebral palsy) [Citation1]. Despite advances in technology, the incidence of cerebral palsy caused by perinatal hypoxic is still higher than 2 per 11,000 newborns [Citation2]. The exhaustion of brain cell energy production, the reduction of tissue glucose metabolism as well as the occurrence and development of cell damage are closely related to HIE [Citation3,Citation4]. Since the time and reason of brain damage caused by HIE are difficult to determine, there are no signals and methods for the effective treatment of HIE currently. The treatment of HIE is still a momentous clinical problem. Therefore, it is pressing to investigate a novel and effective way for HIE treatment.
Circular RNA (circRNA) is an innovative RNA taken shape by covalent loop closure and widely discovered in eukaryotes. CircRNA is derived from a gene exon or intron region and is extensive in mammalian cells. Researches have shown that circRNAs are preserved in various species [Citation5,Citation6]. In addition, because of circRNAs’ unique structure of ring and the characteristic of non-degradation by RNase R, circRNA is fairly stable. With its specific expression, regulatory complexity and critical role in diseases, circRNA has received an increasing number of public attention [Citation7]. The cZNF292 is one of circRNAs family and silencing cZNF292 was found to be involved in suppressing the tube formation in vitro under hypoxic conditions, which might be related to the pathogenesis, development and prognosis of tumour [Citation8]. However, the effect of cZNF292 on HIE is still widely unknown.
Accumulating evidence has released that microRNAs (miRNAs) participate in a variety of diseases including ischaemic stroke [Citation9,Citation10]. The mature miRNAs consisted of approximately 21–22 nucleotides, and usually suppress the expression of related gene by combining the 3′ untranslated region (3′ UTR) of targeted mRNAs [Citation11,Citation12]. Abundant evidence showed that miRNAs participated in HIE in vivo and in vitro. For example, Yang et al. demonstrated that the up-regulation of miR-325-3p repressed pineal aralkylamine N-acetyltransferase of neonatal HIE brain damage in rats [Citation13]. In addition, Yan et al. indicated that miR-208 was involved in the protection of Ginsenoside Rb1 of hypoxia/ischaemia damage in cardiomyocytes [Citation14]. Furthermore, miR-22 presented cardioprotective effect against hypoxia-induced damage in vitro [Citation15,Citation16].
In the current study, we constructed oxygen-glucose deprivation/reperfusion (OGD/R)-induced injury model in neural stem cells (NSCs) to mimic HIE, and the regulatory effects of cZNF292 and miR-22 on OGD/R-treated NSCs were investigated. Furthermore, the molecular mechanism of cZNF292 on OGD/R-treated NSCs was further explored.
Materials and methods
Cell culture
NSCs were isolated from the hippocampus of E14 rats, and the methods of acquisition and cultivation of NSCs referred to previous study [Citation17]. The steps were as follows: the rats were weighted and injected (2 ml/kg) with chloral hydrate. After anaesthesia, the hippocampus was dissected and digested into single-cell suspension with 0.125% trypsin. Then, NSCs (at a density of 1 × 104 cells/ml) were cultured in flasks with NSCs complete culture medium (DMEM/F12) added by 2% B27, 10 ng/ml EGF, 10 ng/ml bFGF and 100 U/ml penicillin/streptomycin in a chamber with 5% CO2 and the temperature of 37 °C. After the cultivation of five days, neurospheres were dissociated and seeded at a density of 1–2 cells/well in 96-well plates. Then, subclonal neurospheres were passaged three times to obtain neurospheres originating from a single primary cell to perform following experiments.
Cell treatment
After culture, glucose-free DMEM was used to culture NSCs. Next, NSCs were hatched in an incubator including 5% CO2 and 95% N2 for 2 h. After treatment, cell media was reverted to complete media before finishing OGD treatment. Then, cells were cultivated in normal incubator for 24 h.
Cell counting kit-8 (CCK-8) assay
NSCs at a density of 5 × 103 were hatched in a 96-well plate. After the treatment, viability was assessed utilizing CCK-8 (Donjindo Molecular Technologies, Kumamoto, Japan). In brief, after culture, 10 μl CCK-8 solution was added. After the hatch of 1 h with CCK-8 reagent, absorbance was assessed at 450 nm applying a Microplate Reader (Bio-Rad, Hercules, CA).
Apoptosis assay
Apoptosis of OGD/R-induced and transfected NSCs were assessed utilizing Annexin V/FITC/PI apoptosis detection kit (Biosea Biotechnology, Beijing, China). To be specific, treated cells were rinsed twice with PBS (Solarbio, Beijing, China) and re-suspended in buffer. Five microlitres Annexin V-FITC and 5 μl PI were added into the suspension and cells were stained for 15 min in the dark at room temperature. Then, the fluorescence intensities of stained cells were determined by utilizing flow cytometry assay (Beckman Coulter, Fullerton, CA). All data were measured by FlowJo software (Tree Star, San Carlos, CA).
Transfection
Overexpressing cZNF292 with pc-DNA3.1 plasmids (cZNF292), small interfering RNA oligonucleotides targeting cZNF292 (si-cZNF292), miR-22 inhibitor and their own negative control (pc-DNA3.1 plasmids (Vehicle), si-NC and NC inhibitor) were produced and purchased by Santa Cruz Biotechnology (Santa Cruz, CA). NSCs were transfected with cZNF292, Vehicle, si-cZNF292, si-NC, miR-22 inhibitor and NC inhibitor by utilizing Lipofectamine 3000 reagent (Invitrogen, Carlsbad, CA) according to producer’s manual. After transfection for 48 h, all cells were harvested for follow-up experiments.
Quantitative reverse transcription PCR (qRT-PCR)
Total RNA was separated from NSCs on basis of producer’s protocols of Trizol reagent (Thermo Fisher Scientific, Waltham, MA). MiRNA reverse transcription was applied by the Multiscribe RT kit (Biosystems, Barcelona, Spain). The cZNF292 and miR-22 expression was detected utilizing QuantiNova SYBR Green PCR Kit (TaKaRa, Tokyo, Japan). The PCR was performed by the SYBR® Green Master Mix (TaKaRa, Tokyo, Japan) according to user’s manual. The relative expression was estimated by the 2−△△Ct method.
Western blot assay
NSCs at a density of 3 × 105 were seeded in six-well plates. After treatment, cells and lysed by using RIPA lysis buffer (Millipore, Billerica, MA) and collected in (Ep) Eppendorf tubes, and then centrifuged at 12,000×g at 4 °C for 10 min. After centrifugation, the supernatant were evaluated and the concentration of all proteins was obtained by BCA kit (Sigma Aldrich, St. Louis, MO). Then, 100 μl samples were isolated in a 10% SDS-PAGE gel and moved onto a PVDF membrane (Millipore, Billerica, MA). After the membrane was incubated with 10% BSA at room temperature, the expression of extracted proteins was cultivated with primary antibodies of Bax (Cell Signaling Technology, Danvers, MA), cleaved-PARP (Cell Signaling Technology, Danvers, MA), cleaved-caspase-3 (Cell Signaling Technology, Danvers, MA), Wtn3a (Cell Signaling Technology, Danvers, MA), β-catenin (Cell Signaling Technology, Danvers, MA), t-PKC (Cell Signaling Technology, Danvers, MA), p-PKC (Cell Signaling Technology, Danvers, MA), t-ERK (Cell Signaling Technology, Danvers, MA), p-ERK (Cell Signaling Technology, Danvers, MA) and β-actin (Cell Signaling Technology, Danvers, MA) at 4 °C overnight. After rinsing, the treated membranes were conjuncted with secondary antibodies for 1 h. Subsequently, the membranes with blots were transferred to the Bio-Rad ChemiDoc™ XRS system (Bio-Rad, Hercules, CA). Ultimately, every protein band was determined utilizing Image Lab™ Software (Bio-Rad, Hercules, CA).
Statistical analysis
Total data of various experiments were represented as a way of the mean ± SD and computed by SPSS 19.0 statistical software (IBM, New York, NY). p Value was computed with a one-way analysis of variance (ANOVA) or Student’s t-test. p < .05 denotes statistically significant results.
Results
OGD/R stimulated the apoptosis of NSCs
After NSCs were subjected to the treatment of OGD/R, viability, apoptotic rate and apoptosis-related protein expressions in NSCs were investigated. Compared with the control group, cell viability was markedly diminished in the OGD/R-treated group (p < .01, ). Oppositely, numbers of apoptotic cells were dramatically increased after OGD/R treatment (p < .001, ). The expression of Bax, cleaved-PARP and cleaved-caspase-3 was all significantly raised when suffering from OGD/R treatment (p < .001, ). All the above data indicated that OGD/R could promote the damage of NSCs. This implied that the establishment of in vitro injury model was successful.
Figure 1. Effect of OGD/R on the viability and apoptosis of NSCs. When NSCs were treated by OGD/R, viability and apoptosis were measured by CCK-8, apoptosis assay and western blot. (A) Viability was declined. (B) Apoptosis was escalated. (C, D) Expression of Bax, cleaved-PARP and cleaved-caspase-3 was elevated. **p < .01 or ***p < .001 compared to labelled groups.
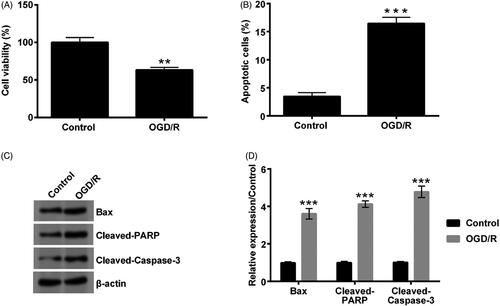
OGD/R promoted cZNF292 expression in NSCs
After NSCs were subjected to OGD/R treatment, we also determined cZNF292 expression. The result stated that cZNF292 expression was strongly up-regulated by OGD/R in NSCs cells (p < .01, ).
The silence of cZNF292 prevented OGD/R-induced damage in NSCs
Next, to determine the function of cZNF292 in OGD/R-induced NSCs damage, we performed the transfection of cZNF292 and si-cZNF292. We found that cZNF292 expression was strongly enhanced after overexpressing plasmid was transfected into NSCs (p < .01, ), this was the mark of our successful transfection and laid the foundation for the following experiments. Compared with the group of OGD/R plus vehicle, viability was further reduced by overexpressing cZNF292 before OGD/R treatment (p < .05, ). Furthermore, apoptotic cells number and Bax, cleaved-PARP and cleaved-caspase-3 expression also further elevated when overexpressing cZNF292 before the treatment of OGD/R (p < .05 or p < .01, ). Sequentially, si-cZNF292 was transfected into NSCs, and cZNF292 expression was remarkably declined (p < .01, ). Then, we discovered that the transfection of si-cZNF292 before OGD/R treatment could attenuate NSCs viability induced by OGD/R (p < .01, ). Furthermore, the number of apoptotic cells and the expression of Bax, cleaved-PARP and cleaved-caspase-3 were similarly alleviated when silencing cZNF292 before OGD/R treatment (p < .01 or p < .001, ). Hence, it was concluded that the silence of cZNF292 could protect NSCs against OGD/R-brought damage.
Figure 3. Effect of cZNF292 on OGD/R-treated NSCs viability and apoptosis. After the transfection of cZNF292 or si-cZNF292, NSCs were/not treated by OGD/R, and then cZNF292, viability and apoptosis were detected by qRT-PCR, CCK-8, apoptosis assay and western blot. (A) cZNF292 expression was escalated. (B) Viability was declined by overexpressing cZNF292. (C) Numbers of apoptotic cells were raised in NSCs. (D, E) Expression of Bax, cleaved-PARP and cleaved-caspase-3 was collectively improved when overexpressing cZNF292. (F) cZNF292 was repressed when si-cZNF292 was transfected into NSCs. (G) Viability was alleviated when silencing cZNF292. (H) Numbers of apoptotic cells were repressed when silencing cZNF292. (I, J) Expression of Bax, cleaved-PARP and cleaved-caspase-3 was alleviated in OGD/R-treated NSCs. *p < .05, **p < .01 or ***p < .001 compared to labelled groups.
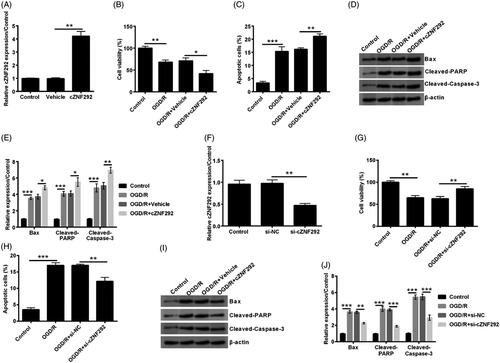
cZNF292 negatively regulated miR-22 expression in NSCs
Studies have reported that miR-22 presents cardioprotective effect against hypoxia-induced damage in vitro [Citation15,Citation16]. Afterwards, the relationship between miR-22 and cZNF292 was analysed. MiR-22 expression was down-regulated by overexpressing cZNF292 (p < .01, ). This indicated that miR-22 expression was negatively regulated by cZNF292.
Silencing cZNF292 attenuated OGD/R-induced NSCs damage through up-regulating miR-22
To determine the function of miR-22 on OGD/R-induced NSCs injury attenuated by silencing cZNF292, as a next step, NC inhibitor and miR-22 inhibitor were transfected into NSCs. In the first place, we conducted the measure of transfection efficiency and observed that miR-22 level was notably declined in NSCs when transfecting miR-22 inhibitor (p < .01, ). The result suggested that miR-22 inhibitor was successfully transfected into NSCs to suppress miR-22 expression level. In the subsequent experiments, compared with the co-transfection of si-cZNF292 and NC inhibitor before OGD/R treatment, viability was observed to be clearly declined by transfecting si-cZNF292 and miR-22 inhibitor before OGD/R treatment (p < .05, ). Apoptotic cell number and Bax, cleaved-PARP and cleaved-caspase-3 expression were clearly elevated when down-regulating miR-22 in NSCs (p < .05 or p < .001, ). Above results implied that silencing cZNF292 weakened OGD/R-caused NSCs damage through elevating miR-22 expression.
Figure 5. Effect of miR-22 on viability and apoptosis in OGD/R and si-cZNF292 co-treated NSCs. When NSCs were transfected by miR-22 inhibitor or NC inhibitor, the level of miR-22 was assessed by qRT-PCR. (A) MiR-22 expression was repressed in miR-22 inhibitor-transfected group. After the transfection of si-cZNF292 or miR-22 inhibitor or both, NSCs were treated by OGD/R, and then viability and apoptosis were detected by CCK-8, apoptosis assay and western blot. (B) Viability was restrained by down-regulating of miR-22. (C) Numbers of apoptotic cells were alleviated by down-regulating of miR-22. (D, E) Expression of Bax, cleaved-PARP and cleaved-caspase-3 was enhanced by down-regulating miR-22. *p < .05, **p < .01 or ***p < .001 compared to labelled groups.
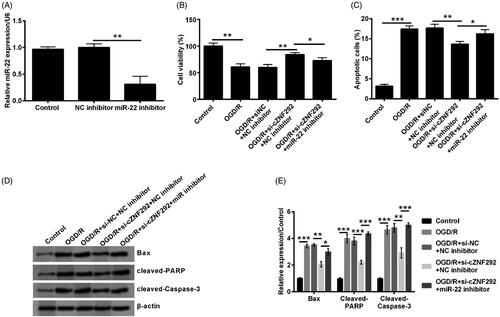
Silencing cZNF292 promoted the activation of Wnt/β-catenin and PKC/ERK pathways via up-regulation of miR-22
The activation and suppression of Wnt/β-catenin and PKC/ERK pathways regulated the occurrence of growth-associated pathological and physiological processes and development, including managing homeostasis under the conditions of damage/stress [Citation18,Citation19]. Finally, the main pathways of Wnt/β-catenin and PKC/ERK were investigated to reveal the potential mechanism of cZNF292 affected on OGD/R-evoked damage in NSCs. Results are represented in . Wnt3a and β-catenin expression was dramatically declined by OGD/R treatment (p < .001). In addition, we observed that silencing cZNF292 attenuated the above decline in NSCs (p < .01). Sequentially, the Wnt3a and β-catenin appeared notably reduction when down-regulating miR-22 (p < .05 or p < .01). Meanwhile, OGD/R treatment decreased the ratio of p/t-PKC and p/t-ERK (p < .001). Silencing cZNF292 increased the ratio of p/t-PKC and p/t-ERK (p < .001). Then, miR-22 down-regulation decreased the ratio of p/t-PKC and p/t-ERK in NSCs (p < .001, ). All the above data illustrated silencing cZNF292 promoted the activation of Wnt/β-catenin and PKC/ERK pathways via up-regulation of miR-22.
Figure 6. Effect of cZNF292 and miR-22 on Wnt/β-catenin and PKC/ERK pathways in OGD/R-treated NSCs. After the transfection of si-cZNF292 or miR-22 inhibitor or both, NSCs were treated by OGD/R, and then Wnt/β-catenin and PKC/ERK pathways-related proteins were evaluated by western blot. (A, B) Protein expression of Wnt3a andβ-catenin was suppressed by OGD/R treatment and then elevated by silencing cZNF292. Down-regulating miR-22 partially declined the expression in NSCs. (C, D) The ratio of p/t-PKC and p/t-ERK was diminished by OGD/R treatment and silencing cZNF292 reversed the effect of OGD/R. Subsequently, the ratio of p/t-PKC and p/t-ERK was further attenuated by down-regulating miR-22. *p < .05, **p < .01 or ***p < .001 compared to labelled groups.
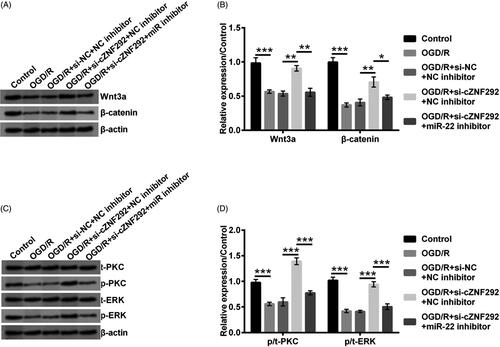
Discussion
HIE, caused by perinatal asphyxia cerebral ischaemia and reperfusion (I/R), is prevailing severe brain damage disease in newborns [Citation20]. HIE pathogenesis is complicated and affected neuro-inflammation, oxidative stress and various growth factors [Citation21,Citation22]. The optimal treatments for HIE, including drug therapy and hypothermia, have been identified to be promising novel methods of HIE treatment [Citation23]. However, perinatal HIE is still a long-standing and difficult to solve the public health problem [Citation24]. In our research, we established OGD/R-induced cell damage model in NSCs to mimic HIE in vitro. As the results presented, OGD/R significantly induced the damage of NSCs and the up-regulation of cZNF292. In addition, we found that the silence of cZNF292 could alleviate OGD/R-induced NSCs injury through the up-regulation of miR-22. Besides, it was stated that silence of cZNF292 induced the activation of Wnt/β-catenin and PKC/ERK pathways via up-regulation of miR-22. These results were fairly crucial for research on the pathogenesis of HIE.
Researches have indicated that the formation of circRNA is accomplished by reverse splicing, which is different from standard linear RNA slicing. In addition, it may be formed by the combination of endogenous miRNA. Some intron-retained circRNAs are located in the nucleus of eukaryotic cells, when it may modulate gene expression, indicating that it may be used as the therapeutic target or biomarker of disease diagnosis [Citation25,Citation26]. The cZNF292 is a kind of circRNA that conducts biological activities in both endothelial cells and tumour cell [Citation8,Citation27]. Limited studies investigated the function of cZNF292 of never cells and tissues for now. In a precious study, cZNF292 was found to participate in the proliferation and tube formation in glioma cells. Further, silencing cZNF292 repressed tube formation through the mobilization of Wnt/β-catenin pathway [Citation27]. Another research elucidated that cZNF292 knockdown repressed proliferation, radioresistance and vasculogenic mimicry (VM) of hypoxic human hepatoma cells [Citation28]. In addition, it was unclosed that hypoxia caused the reduction of cZNF292. The cZNF292 silence restrained the expression of cZNF292 and the formation of tube in vitro endothelial cells [Citation29]. However, whether cZNF292 exerts the neuro-protective effect in OGD/R-treated NSCs remains widely unclear. Similarly, it was herein reported that the silence of cZNF292 exerted a positive role in OGD/R-induced NSCs damage. However, the silence of cZNF292 promoted NSCs growth and inhibited apoptosis in our study, and which was different from previous studies. As the circRNAs–miRNAs–mRNA network was reported, we boldly speculated the above difference from previous reports was resulted by combining different miRNAs to regulate related gene expression.
MiRNAs are also a glass non-coding RNAs and play their function by targeting specific mRNAs. Moreover, circRNAs acted the sponges of miRNAs. Multiple miRNAs are abundant in the nervous system and serve key functions in brain developmental plasticity [Citation30]. Currently, accumulating evidence has uncovered the different functions of miRNAs in various neurological diseases, such as Alzheimer’s disease (AD), Parkinson’s disease (PD), Huntington’s disease (HD) and I/R [Citation16,Citation31–34]. A similar research explored the neuro-protective effect of miR-22 overexpression in vitro and in vivo experiments [Citation33]. Yu et al. also implied that miR-22 overexpression prevented cells against ischaemia/reperfusion-stimulated damage. Moreover, miR-22 was found to be involved in repressing apoptosis in cortical neurons [Citation33]. In the current study, consequences showed that cZNF292 remarkably down-regulated miR-22 expression in NSCs. Further interesting research stated that the protective effect of silencing cZNF292 on OGD/R-induced NSCs damage was abolished by the down-regulation of miR-22. These findings implied that the overexpression of miR-22 might be participated in modulating the protective effect of silencing cZNF292 in OGD/R-treated NSCs.
Wnt pathway is involved in a variety of processes during embryonic development and modulates proliferation, migration, morphology and cell fate at the cellular levels [Citation35]. Wnt/β-catenin pathway was reported to be blocked after hypoxia treatment and enhanced the growth of NSCs [Citation36,Citation37]. Moreover, previous literature demonstrated that HIF-1α fortified Wnt signalling pathway was not fully understood in brain development [Citation38]. Another literature elucidated that β-catenin stimulated hippocampal proliferation partially through CyclinD1 after hypoxia treatment in NSCs [Citation35]. Recent reports implied that HIF-1α mobilized Wnt/β-catenin pathway in NSCs under hypoxic condition [Citation35]. This evidence demonstrated that Wnt/β-catenin pathway was involved in the development and biological progression in NSCs. Moreover, PKC is a key pathway that mediates the permeability of brain microvascular endothelial cells [Citation39]. After brain damage, the blood–brain barrier is open, permeability enhances, and cerebral oedema occurs and develops [Citation40]. Thus, the expression of PKC was determined in the current assay. In addition, in different kinds of neuronal cells and under various different conditions, ERK pathway represented to be modulated survival effects [Citation41]. For instance, ERK pathway was reported by the participation in the protection of dexamethasone on neonatal HIE in vivo [Citation41]. Based on these previous researches, we explored the function of Wnt/β-catenin and PKC/ERK pathways on OGD/R-induced NSCs and found the inactivation of two signalling pathways by OGD/R in NSCs. A study has reported that cZNF292 knockdown facilitated SRY (sex-determining region Y)-box 9 (SOX9) nuclear translocation, and weakened the activity of Wnt/β-catenin pathway, which suppressed hypoxic hepatoma cell proliferation, VM and radioresistance in vitro and in vivo [Citation28]. It has also been reported that miR-22/Wnt1/β-catenin axis administrates the development of oral squamous cell carcinoma (OSCC) [Citation42]. The Wnt/β-catenin was regulated by miR-22/Sirt1 in hypoxia-induced cardiomyocytes [Citation43]. However, our study unclosed that the silence of cZNF292 activated the Wnt/β-catenin pathway through increasing miR-22 in OGD/R-treated NSCs. The study regarding the function of cZNF292 and miR-22 on PKC/ERK pathway was limited. A literature has uncovered that differentiation-associated miR-22 represses max expression and inhibits cell cycle progression through the regulation of PKC/ERK pathway. Nevertheless, we explained that the silence of cZNF292 activated the PKC/ERK pathway through increasing miR-22 in OGD/R-treated NSCs. Above results illustrated that the effect of cZNF292 on Wnt/β-catenin and PKC/ERK pathways by down-regulating miR-22 expression in OGD/R-treated NSCs for the first time. The evidence hinted about the potential molecular mechanism of silencing cZNF292 protecting NSCs against OGD/R-induced damage.
Our research demonstrated that silencing cZNF292 could protect NSCs against OGD/R-induced injury by up-regulating miR-22. The underlying molecular mechanism might be through the mobilization of Wnt/β-catenin and PKC/ERK pathways. These discoveries implied that cZNF292 silence might be a novel therapy target for the treatment of HIE. Further researches are still essential to investigate the wider application of cZNF292 silence in clinic.
Disclosure statement
No potential conflict of interest was reported by the author(s).
References
- Lu Q, Harris VA, Kumar S, et al. Autophagy in neonatal hypoxia ischemic brain is associated with oxidative stress. Redox Biol. 2015;6:516–523.
- Odding E, Roebroeck ME, Stam HJ. The epidemiology of cerebral palsy: incidence, impairments and risk factors. Disabil Rehabil. 2006;28(4):183–191.
- Blumberg RM, Cady EB, Wigglesworth JS, et al. Relation between delayed impairment of cerebral energy metabolism and infarction following transient focal hypoxia-ischaemia in the developing brain. Exp Brain Res. 1997;113(1):130–137.
- Sizemore G, Lucke-Wold B, Rosen C, et al. Temporal lobe epilepsy, stroke, and traumatic brain injury: mechanisms of hyperpolarized, depolarized, and flow-through ion channels utilized as tri-coordinate biomarkers of electrophysiologic dysfunction. OBM Neurobiol. 2018;2(2):1–4.
- Lasda E, Parker R. Circular RNAs co-precipitate with extracellular vesicles: a possible mechanism for circRNA clearance. PLOS One. 2016;11(2):e0148407.
- Lin SP, Ye S, Long Y, et al. Circular RNA expression alterations are involved in OGD/R-induced neuron injury. Biochem Biophys Res Commun. 2016;471(1):52–56.
- Memczak S, Papavasileiou P, Peters O, et al. Identification and characterization of circular RNAs as a new class of putative biomarkers in human blood. PLoS One. 2015;10(10):e0141214.
- Boeckel JN, Jae N, Heumuller AW, et al. Identification and characterization of hypoxia-regulated endothelial circular RNA. Circ Res. 2015;117(10):884–890.
- Irmady K, Jackman KA, Padow VA, et al. Mir-592 regulates the induction and cell death-promoting activity of p75NTR in neuronal ischemic injury. J Neurosci. 2014;34(9):3419–3428.
- Moon JM, Xu L, Giffard RG. Inhibition of microRNA-181 reduces forebrain ischemia-induced neuronal loss. J Cereb Blood Flow Metab. 2013;33(12):1976–1982.
- Klein ME, Impey S, Goodman RH. Role reversal: the regulation of neuronal gene expression by microRNAs. Curr Opin Neurobiol. 2005;15(5):507–513.
- Ma Q, Dasgupta C, Li Y, et al. MicroRNA-210 suppresses junction proteins and disrupts blood–brain barrier integrity in neonatal rat hypoxic-ischemic brain injury. Int J Mol Sci. 2017;18(7).
- Yang Y, Sun B, Huang J, et al. Up-regulation of miR-325-3p suppresses pineal aralkylamine N-acetyltransferase (Aanat) after neonatal hypoxia-ischemia brain injury in rats. Brain Res. 2017;1668:28–35.
- Yan X, Liu J, Wu H, et al. Impact of miR-208 and its target gene nemo-like kinase on the protective effect of ginsenoside Rb1 in hypoxia/ischemia injured cardiomyocytes. Cell Physiol Biochem. 2016;39(3):1187–1195.
- Yang J, Chen L, Ding J, et al. Cardioprotective effect of miRNA-22 on hypoxia/reoxygenation induced cardiomyocyte injury in neonatal rats. Gene. 2016;579(1):17–22.
- Chen Z, Qi Y, Gao C. Cardiac myocyte-protective effect of microRNA-22 during ischemia and reperfusion through disrupting the caveolin-3/eNOS signaling. Int J Clin Exp Pathol. 2015;8(5):4614–4626.
- Zhang X, Zhang L, Cheng X, et al. IGF-1 promotes Brn-4 expression and neuronal differentiation of neural stem cells via the PI3K/Akt pathway. PLoS One. 2014;9(12):e113801.
- L’Episcopo F, Serapide MF, Tirolo C, et al. A Wnt1 regulated frizzled-1/beta-catenin signaling pathway as a candidate regulatory circuit controlling mesencephalic dopaminergic neuron-astrocyte crosstalk: therapeutical relevance for neuron survival and neuroprotection. Mol Neurodegener. 2011;6:49.
- Xu BY, Tang XD, Chen J, et al. Rifampicin induces clathrin-dependent endocytosis and ubiquitin-proteasome degradation of MRP2 via oxidative stress-activated PKC-ERK/JNK/p38 and PI3K signaling pathways in HepG2 cells. Acta Pharmacol Sin. 2019;41(1):56–64.
- Vasiljevic B, Maglajlic-Djukic S, Gojnic M, et al. New insights into the pathogenesis of perinatal hypoxic-ischemic brain injury. Pediatr Int. 2011;53(4):454–462.
- Vasiljevic B, Maglajlic-Djukic S, Gojnic M, et al. The role of oxidative stress in perinatal hypoxic-ischemic brain injury. Srp Arh Celok Lek. 2012;140(1–2):35–41.
- Yuan Y, Zheng Z. Geniposide protects PC-12 cells against oxygen and glucose deprivation-induced injury by up-regulation of long-noncoding RNA H19. Life Sci. 2019;216:176–182.
- Chalak LF, Sanchez PJ, Adams-Huet B, et al. Biomarkers for severity of neonatal hypoxic-ischemic encephalopathy and outcomes in newborns receiving hypothermia therapy. J Pediatr. 2014;164(3):468–474.e1.
- Lu Q, Harris VA, Rafikov R, et al. Nitric oxide induces hypoxia ischemic injury in the neonatal brain via the disruption of neuronal iron metabolism. Redox Biol. 2015;6:112–121.
- Warton K, Samimi G. Methylation of cell-free circulating DNA in the diagnosis of cancer. Front Mol Biosci. 2015;2:13.
- Liu Q, Zhang X, Hu X, et al. Circular RNA related to the chondrocyte ECM regulates MMP13 expression by functioning as a MiR-136 ‘Sponge’ in human cartilage degradation. Sci Rep. 2016;6(1):22572.
- Yang P, Qiu Z, Jiang Y, et al. Silencing of cZNF292 circular RNA suppresses human glioma tube formation via the Wnt/beta-catenin signaling pathway. Oncotarget. 2016;7(39):63449–63455.
- Yang W, Liu Y, Gao R, et al. Knockdown of cZNF292 suppressed hypoxic human hepatoma SMMC7721 cell proliferation, vasculogenic mimicry, and radioresistance. Cell Signal. 2019;60:122–135.
- Fiedler J, Baker AH, Dimmeler S, et al. Non-coding RNAs in vascular disease – from basic science to clinical applications: scientific update from the Working Group of Myocardial Function of the European Society of Cardiology. Cardiovasc Res. 2018;114(10):1281–1286.
- Ma Q, Dasgupta C, Li Y, et al. Inhibition of microRNA-210 provides neuroprotection in hypoxic-ischemic brain injury in neonatal rats. Neurobiol Dis. 2016;89:202–212.
- Yang CP, Zhang ZH, Zhang LH, et al. Neuroprotective role of microRNA-22 in a 6-hydroxydopamine-induced cell model of Parkinson’s disease via regulation of its target gene TRPM7. J Mol Neurosci. 2016;60(4):445–452.
- Wang Y, Zhao L, Kan B, et al. miR-22 exerts anti-alzheimic effects via the regulation of apoptosis of hippocampal neurons. Cell Mol Biol (Noisy-le-Grand). 2018;64(15):84–89.
- Yu H, Wu M, Zhao P, et al. Neuroprotective effects of viral overexpression of microRNA-22 in rat and cell models of cerebral ischemia-reperfusion injury. J Cell Biochem. 2015;116(2):233–241.
- Jovicic A, Zaldivar Jolissaint JF, Moser R, et al. MicroRNA-22 (miR-22) overexpression is neuroprotective via general anti-apoptotic effects and may also target specific Huntington’s disease-related mechanisms. PLoS One. 2013;8(1):e54222.
- Qi C, Zhang J, Chen X, et al. Hypoxia stimulates neural stem cell proliferation by increasing HIF1alpha expression and activating Wnt/beta-catenin signaling. Cell Mol Biol (Noisy-le-Grand). 2017;63(7):12–19.
- Duncan AW, Rattis FM, DiMascio LN, et al. Integration of Notch and Wnt signaling in hematopoietic stem cell maintenance. Nat Immunol. 2005;6(3):314–322.
- Kaidi A, Williams AC, Paraskeva C. Interaction between beta-catenin and HIF-1 promotes cellular adaptation to hypoxia. Nat Cell Biol. 2007;9(2):210–217.
- Mazumdar J, O'Brien WT, Johnson RS, et al. O2 regulates stem cells through Wnt/beta-catenin signalling. Nat Cell Biol. 2010;12(10):1007–1013.
- Wu PY, Lai B, Dong Y, et al. Different oxidants and PKC isozymes mediate the opposite effect of inhibition of Q(i) and Q(o) site of mitochondrial complex III on calcium currents in rat cortical neurons. Biochim Biophys Acta. 2010;1803(9):1072–1082.
- Aras MA, Hara H, Hartnett KA, et al. Protein kinase C regulation of neuronal zinc signaling mediates survival during preconditioning. J Neurochem. 2009;110(1):106–117.
- Han BH, Holtzman DM. BDNF protects the neonatal brain from hypoxic-ischemic injury in vivo via the ERK pathway. J Neurosci. 2000;20(15):5775–5781.
- Zhang C, Hao Y, Sun Y, et al. Quercetin suppresses the tumorigenesis of oral squamous cell carcinoma by regulating microRNA-22/WNT1/beta-catenin axis. J Pharmacol Sci. 2019;140(2):128–136.
- Zhang S, Zhao Y. Lentinan protects cardiomyocytes against hypoxia-induced injury by regulation of microRNA-22/Sirt1. Artif Cells Nanomed Biotechnol. 2019;47(1):3938–3946.