Abstract
The advent of nanoparticles revolutionised the drug delivery systems in human diseases; however, their prominent role was highlighted in the cancer-based therapies, where this technology could specifically target cancer cells. Herein, we decided to combine two nanoparticles Fe3O4 and ZnO to fabricate a new anti-cancer nanocomposite. Noteworthy, hydroxylated carbon nanotube (CNT) was used to increase the water-solubility of the compound, improving its uptake by malignant cells. This study was designed to evaluate the anticancer property as well as the molecular mechanisms of ZnO/CNT@Fe3O4 nanocomposite cytotoxicity in CML-derived K562 cells. Our results outlined that ZnO/CNT@Fe3O4 decreased the proliferative capacity of K562 cells through induction of G1 arrest and induced apoptosis probably via ROS-dependent upregulation of FOXO3a and SIRT1. The results of qRT-PCR analysis also demonstrated that while ZnO/CNT@Fe3O4 significantly increased the expression of pro-apoptotic genes in K562 cells, it had no significant inhibitory effect on the expression levels of anti-apoptotic target genes of NF-κB; proposing an attenuating role of NF-κB signalling pathway in K562 cell response to ZnO/CNT@Fe3O4. Synergistic experiment showed that ZnO/CNT@Fe3O4 could enhance the cytotoxic effects of imatinib on K562 cells. Overall, it seems that pharmaceutical application of nanocomposites possesses novel promising potential for leukaemia treatment strategies.
Introduction
Over the last decades and in the era of tyrosine kinase inhibitors (TKI), major advances have been allocated to the management of chronic myeloid leukaemia (CML), as one of the most prevalent haematologic malignancy defined by the BCR–ABL fusion oncogene [Citation1]. Although this novel targeted therapy has brought lifelong advantages in most of CML patients [Citation2], a proportion of patients have not well responded to TKI, leading to disease re-progression [Citation3,Citation4]. This resistance event has founded a greater extent of substantial efforts to identify the optimal therapy for this malignancy. Once nanotechnology-based therapeutic has been approved for clinical application in a number of patients [Citation5], intense interest has been devoted into the identification of specific diseases which this modern technology could exert beneficial influence on their treatment strategies. Owing to their unique physical and biological properties, soon enough, the nanotechnology-based drugs found their ways into cancer treatment and revolutionized the paradigm of conventional approaches [Citation6]. Among synthesized nanomaterials, metal nanoparticles (NP) have been explored as biomedical and therapeutic agents for a variety of disorders because of their unique properties together with negligible side effects [Citation7].
In the last decade, ZnO quantum dots (QD) and Fe3O4 magnetic NPs have attracted significant consideration among metal NPs and they have been applied in a wide range of biomedical areas. Noteworthy, cytotoxic effects of these compounds have also been well described in a wide array of solid and haematologic malignant cell lines [Citation8,Citation9]. Bai et al. has indicated that ZnO NPs exerted cytotoxic effect on human ovarian cancer cells (SKOV3) through generation of reactive oxygen species (ROS) [Citation10]. Moreover, in another study, it has been suggested that Fe3O4 NPs could be a promising nanomaterial to manipulate CD56+ natural killer (NK) cells, thereby inhibiting lung cancer growth [Citation11]. The most prominent property of these metal nanoparticles is their selective cytotoxicity against cancer cells, which is guaranteed by their selective uptake due to the high expression of membrane anionic phospholipids on cancer cells [Citation9]. This selective activity coupled with minimal cytotoxic effect on the normal cells have been reported in several reports for both ZnO [Citation12,Citation13] and Fe3O4 NPs [Citation14]. Given to the low toxicity profile and the promising efficacy on malignant cells, it was interesting to combine Fe3O4 and ZnO in the form of nanocomposites and to use the anti-cancer property of these two nanoparticles simultaneously. Based on low solubility of metal NPs in the liquid medium [Citation15], we decided to use hydroxylated carbon nanotubes (CNT) as a soluble compound to make our nanocomposite completely water-soluble [Citation16]. Of note, successful formation of ZnO/CNT@Fe3O4 nanocomposite was further characterized using UV-spectroscopy, FTIR, SEM, and XRD. Afterwards, to evaluate the anti-leukemic property of our new-designed nanocomposite, CML-derived K562 cells were subjected to the different concentrations of this product. The present study showed that ZnO/CNT@Fe3O4 nanocomposite exerted a concentration-dependent growth suppressive effect in K562 cells, either as a single agent or in a combination with imatinib. As far as we are aware, to date, no study has reported the anti-leukemic activity of ZnO/CNT@Fe3O4 in CML cell lines, and our data depicted for the first time that ZnO/CNT@Fe3O4 induced a ROS-dependent apoptotic cells death presumably through upregulation of FOXO3a and SIRT1 in CML-derived K562 cells.
Material and methods
Synthesis and formulation of hydrophilic ZnO/CNT@Fe3O4 nanocomposite
For this new and active nanoformulation, we used 1–3 gr multiwall CNT (8–15 nm) which had been modified with iron oxide nanoparticles (NPs) and 0.5–2 gr ZnO NPs which coated with hydrophilic CNT@fatty acid in 20 ml pure ethanol. This nanofluid was dissolved in 1–2 gr zinc oxide NPs powder as synergic material in nanosolution. They were mixed together at room temperature. Then, 1–2 g polyethylene glycol 6000 (PEG 6000) as binding agent in distilled water were added and the mixture was stirred at 40–50 °C for 1–2 h. Afterwards, 2–3 ml Span 80 (Sorbitane monooleate, Merck company) as an active water solution, non-ionic surfactant, stabilizer and emulsifier oil/water was added with continued vigorous stirring, under reflux processing about 2 h duration of reaction.
Cell culture and drug treatment
To investigate the effects of ZnO/CNT@Fe3O4 on chronic myeloid leukaemia cells, CML-derived K562 cells were cultured in RPMI 1640 medium supplemented with 10% heat inactivated foetal bovine serum, antibiotics and 2 mmol/l L-glutamine in a humidified incubator with 5% CO2 at 37 °C. The relevant amount of ZnO/CNT@Fe3O4 drug was dissolved in sterile PBS to make a stock solution. Moreover, proteasome inhibitor Bortezomib (BTZ) (Selleckchem, Munich, Germany), imatinib (Sigma) and autophagy inhibitor chloroquine (CQ) (Sigma, Taufkirchen, Germany) were prepared, divided to aliquots, and stored at −20 °C until use. K562 cells were treated with the relevant amounts of the nanocomposite, either alone or in combination with other inhibitors.
Trypan blue assay test of cell count and viability
To assess the suppressive effect of ZnO/CNT@Fe3O4 on the viability and growth kinetics, K562 cells were seeded at 2 × 105 cells/ml and incubated with designated concentrations of ZnO/CNT@Fe3O4, either alone or in combination with imatinib and other inhibitors. After indicated treatment intervals, drug-treated cells were mixed with 0.4% trypan blue solution (Invitrogen) in a 1:1 ratio and then the mixture was allowed to incubate for 1–2 min at room temperature and loaded onto the chamber of Neubauer haemocytometer. The total number of unstained (viable) and stained (non-viable) cells were manually counted and the percentage of viable cells was calculated.
Detection of metabolic activity by microculture tetrazolium test
To assess whether treatment of the cells with ZnO/CNT@Fe3O4, as a single agent or in a combined modality, could reduce the metabolic activity, K562 cells were treated with different concentrations of the nanocomposite in the presence or the absence of imatinib. Moreover, to investigate whether inhibition of NF-κB signalling pathway using BTZ could potentiate the cytotoxic effect of ZnO/CNT@Fe3O4, MTT assay was applied. After treatment of K562 cells with different agents, we incubated the cells with 100 μl of MTT solution for a further 3 h in a humidified incubator. The optical densitometry of a resulting formazan solubilized with DMSO was measured in an enzyme-linked immunosorbent assay (ELISA) reader at the wavelength of 570 nm. The percentage of metabolic activity was calculated as (%) = (ODexp/ODcon) ×100; where ODexp and ODcon are the optical densities of exposed and control cells, respectively.
Median-effect analysis of drug combinations
To evaluate the interaction between ZnO/CNT@Fe3O4 and imatinib, the combination index (CI) was computed using the method developed by Chou and Talalay and the computer software CalcuSyn according to the classic isobologram equation. The CI values of <1, =1, and >1 indicate synergism, additive effect, and antagonism of drugs, respectively. The dose which may be reduced in a combination for a given level of effect as compared to the concentration of individual drug alone defined as dose reduction index (DRI) and calculated as follow: (DRI)1 = (Dx)1/(D)1 and (DRI)2 = (Dx)2/(D)2.
BrdU cell proliferation assay
The suppressive effect of ZnO/CNT@Fe3O4 on the growth and proliferation of K562 cells was assessed by measuring DNA synthesis rate of the cells using a colorimetric bromodeoxyuridine (BrdU)-based cell proliferation ELISA kit (Roche, Penzberg, Germany) as per manufacturer’s recommendations. Initially, K562 cells were seeded into 96-well plate at a density of 5000 cells/well and treated with the indicated concentrations of ZnO/CNT@Fe3O4. Afterwards, 10 μl/well of BrdU labelling solution was added, and the cells were re-incubated at 37 °C for 12 h. Then, cells were fixed and DNA was denatured using 200 μl of FixDenat solution provided with the kit. After 30 min and discarding Fixodent, 100 μl peroxidase-conjugated anti-BrdU (antiBrdU-POD) antibody was added to each well. Finally, the cells were incubated with tetramethylbenzidine (TMB) for 3 min at room temperature and the reaction product was quantified by measuring the absorbance at 450 nm.
Cell cycle distribution analysis
The impact of ZnO/CNT@Fe3O4 on the distribution of the cells in the different phases of cell cycle were ascertained by flow cytometric analysis after 24 h incubation of K562 cells with different concentrations of ZnO/CNT@Fe3O4. In brief, 1 × 106 cells were harvested, washed twice with cold PBS, and then fixed in 70% ethanol. Next, propidium iodide (PI) and RNase were used to stain DNA and degrade RNA, respectively. DNA content of the cells was quantified from the peak analysis of flowcytometric histograms, and the data were interpreted using the Windows FlowJo V10 software.
Assessment of apoptosis using flow cytometry
To investigate ZnO/CNT@Fe3O4 effect on the induction of programmed cell death, CML cells were subjected to apoptosis analysis. Briefly, K562 cells were harvested after 24 h of treatment with the designated concentrations of the ZnO/CNT@Fe3O4, washed with PBS, and re-suspended in a total volume of 100 μl of the incubation buffer at a concentration of 1 × 106 cells/ml. After that, Annexin-V-Flous (2 μl per sample) was added, and cell suspensions were incubated for 20 min in the dark. After incubation, Fluorescence was quantified using flow cytometry.
RNA extraction and cDNA synthesis
Total RNA from ZnO/CNT@Fe3O4-treated CML cells was extracted using RNA Isolation Kit (Roche, Mannheim, Germany) and quantified by Nanodrop instrument. The reverse transcription reaction was performed using Complementary DNA (cDNA) Synthesis Kit (Takara Bio, Shiga, Japan). Adapted times and temperature profiles for the reverse transcription were used: incubation for 5 min at 65 °C, 5 min at 25 °C, followed by 60 min at 42 °C. The reaction was terminated by heating for 5 min at 70 °C.
Quantitative real-time PCR
Changes in mRNA expression level of the desired genes were assessed by real-time PCR that was performed with a light cycler instrument (Roche Diagnostics, Germany) using SYBR Premix Ex Taq technology (Takara Bio, Inc). For this purpose, PCR assay was performed in an ultimate volume of 20 μl of reaction mixture containing 10 μl of SYBR Green master mix, 2 μl of cDNA product, 0.5 μl of each forward and reverse primers (10 pmol) and 7 μl of nuclease-free water (Qiagen, Hilden, Germany). Thermal cycling conditions included an initial activation step for 30 s at 95 °C followed by 40 cycles including a denaturation step for 5 s at 95 °C and a combined annealing/extension step for 20 s at 60 °C. Melting curves were analysed to verify single PCR product of each primer, and fold change in the expression of each target mRNA relative to HPRT as an internal control was calculated on the basis of a comparative on 2−ΔΔct relative expression formula.
Intracellular reactive oxygen species detection
To determine the effect of ZnO/CNT@Fe3O4 on the amount of intra-cellular reactive oxygen species (ROS) in CML-derived K562 cells, we used a fluorogenic dye DCFH-DA for measuring hydroxyl, peroxyl and other ROS activity within the cell. After incubation with the desired concentrations of the nanocomposite, the cells were incubated with DCFH-DA at 37 °C for 30 min. Finally, fluorescence intensities of the samples were detected by fluorescence spectrophotometer (Cary Eclipse, USA) with excitation at 485 nm and emission at 530 nm.
Detection of autophagy by acridine orange staining
CML cells were treated with different concentrations of autophagy inhibitor CQ (Sigma) for 24 h and washed with PBS for three times. Afterward, the cells were stained with 1 µg/ml acridine orange (Merck, Darmstadt, Germany) for 15 min in the dark and visualised under a fluorescence microscope (Labomed, Los Angeles, USA). Owing to differences in acidity, autophagic lysosomes appeared as orange/red fluorescent cytoplasmic vesicles, while the cytoplasm and nucleolus were green.
Statistical analysis
Experimental data were evaluated in triplicate against untreated control cells and collected from three independent experiments. The significance of differences between experimental variables was determined by the use of two-tailed student’s test. In order to compare between the control group and the treated ones, the Dunnett’s multiple comparison test was used. All data are presented as mean ± standard deviation (SD) and a probability level of p ≤ .05 was considered statistically significant.
Results
Characterisations of the synthesised ZnO/CNT@Fe3O4 nanofluid product
After synthesising the desired nanofluid product, it was of particular interest to verify and characterize its structure and special functional groups. shows FTIR spectrum of ZnO/CNT@Fe3O4 from 760 and 3800 cm−1. The presence of ZnO and Fe3O4 nanoparticles was confirmed by the symmetrical stretching vibration of Zn–O and iron oxide (Fe–O) magnetic nanoparticles (NPs) groups which were appeared in 721 cm−1 and 838 cm−1, respectively. Moreover, the presence of polyethylene glycol (PEG) in the nanofluid was established by two peaks located at 1462 cm−1 and 1399 cm−1 which are related to the symmetric and asymmetric –CH3. Three absorption bands corresponding for C–O–C [Citation17,Citation18], C=O [Citation19,Citation20] could be assigned to the symmetrical stretching vibration of COO– and C=C bond in carbon sp2 hybridization (carbon–carbon bonds of CNT nanotubes), which were observed at 1110 cm−1, 1735 cm−1 and 1645 cm−1, respectively (). To more precisely characterize the structure of the fabricated ZnO/CNT@Fe3O4 nanoproduct, we further used UV- spectroscopy, XRD, transmission electron microscopy (TEM) and scanning electron microscopy (SEM) images. The results obtained from the UV-Vis absorbance spectrum of nanoparticles in nanoproduct revealed that the mean size of these nanoparticles are very small and maximum peaks at 190 and 204 nm wavelengths showed that this nanofluid contains very fine nanoparticles and high band gaps energy (). This finding was further confirmed by XRD analysis which showed that the analyzed x-ray diffraction peaks were matched well with the standard XRD patterns of zinc oxide (JCPDS no.: 017-751377) and iron oxide magnetic nanoparticles (JCPDS no: 00-003-0863) in this new system. The main dominant peaks for CNT were detected at 2θ = 24.5 that could be indexed as (002) planes of CNT (). The small size of the newly synthesized nanoparticle was also validated by TEM analysis. The interesting new morphology of the ZnO/CNT@Fe3O4 nanocomposites in nanoproduct () was indicated by the scanning electron microscopy (SEM) images. As presented in , Q-dot ZnO NPs and hydrophilic CNT nanoparticles are attached on the surface of magnetic iron oxide nanoparticles (Fe3O4). Spherical fine Q-dot ZnO NPs, as activator material, and Fe3O4 NPs, as a spherical suitable substrate, are seen with carbon nanotube (CNT) NPs, which play as a mediator between Fe3O4 NPs and fatty acid reagent in nanocomposites product.
Figure 1. Characteristic of ZnO/CNT@Fe3O4 nanofluid product. (A) FTIR spectrum of ZnO/CNT@Fe3O4 nanocomposite. (B) ZnO/CNT@Fe3O4 exhibited high absorption peaks at 190 and 204 nm wavelengths and a few low ladder shaped peaks in UV visible. (C) XRD analysis confirmed the structural characteristics of ZnO/CNT@Fe3O4. (D) Scanning electron microscopy (SEM) and transmission electron microscopy (TEM) of the nanoparticle. SEM illustrated the morphology of the ZnO/CNT@Fe3O4 nanocomposites in nano-product. Spherical fine Q-dot ZnO NPs and Fe3O4 NPs are combined together with CNT NPs. The results of TEM also validated the small size of this nanocomposite at 75 nm.
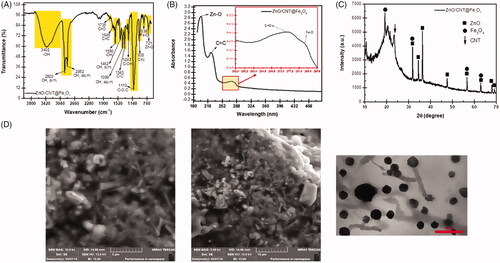
Time- and concentration-dependent inhibitory effects of ZnO/CNT@Fe3O4 on K562 cell survival
Cytotoxic effects of ZnO/CNT@Fe3O4 nanocomposite against K562 cells were evaluated by the investigation of its inhibitory effect on viability and metabolic activity using both the trypan blue exclusion and MTT assays. Our results showed that ZnO/CNT@Fe3O4 significantly inhibited cell viability of K562 in a concentration-dependent manner as compared to the untreated cells. As presented in , 24 h treatment with ZnO/CNT@Fe3O4 nanocomposite reduced the viability to 76% and 58% at the concentration of 15 and 20 μg/ml, respectively. Accordingly, time-dependent experiments showed that the metabolic activity was considerably hindered upon exposure of K562 cells to the agent with maximal repression observed at 20 μg/ml.
Figure 2. Treatment of K562 cells with ZnO/CNT@Fe3O4 significantly inhibited cell viability and metabolic activity of cells in both time- and concentration-dependent manner. Values are given as mean ± standard deviation of three independent experiments. *p ≤ .05 represents significant changes from untreated control.
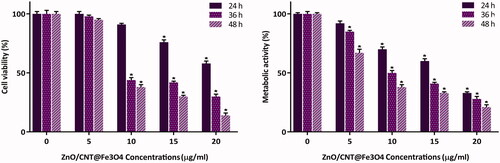
Zno/CNT@Fe3O4 inhibited proliferation through accumulation of K562 cells in G1 phase
To investigate whether ZnO/CNT@Fe3O4 cytotoxicity against K562 was coupled with the alteration of cell distribution in different phases of cell cycle, PI staining was applied. Of particular interest, we found that the antiproliferative effect of the agent, as evident by the decreased number of viable cells (), was accompanied with a significant reduction of cell population in S phase (). Moreover, DNA content analysis and results collected from cell cycle experiments showed that 24 h exposure to ZnO/CNT@Fe3O4 increased the percentage of cell populations in G1 phase from 33.2% in the control group to 50.8% in 20 μg/ml-treated cells; indicating that the growth suppressive effect of ZnO/CNT@Fe3O4 was mediated, at least partly, through the induction of G1 arrest (). Our results were further strengthened by analyzing DNA replication of the cells using the BrdU incorporation assay. As presented in , 24 h treatment resulted in a significant decrease in DNA synthesis nearly by 60 and 67% at the concentrations of 15 and 20 μg/ml, respectively. Molecular analysis of the critical genes responsible for the transition from G1 to the S phase further confirmed our results, where we found that while the mRNA expressions of both cyclin-dependent kinase inhibitors p21 and p27 were upregulated, the expression level of c-Myc as a master regulator of cell proliferation was significantly reduced in K562-treated cells ().
Figure 3. ZnO/CNT@Fe3O4 suppressed the proliferative capacity of K562 cells through alteration of cell distribution in cell cycle. (A) Treatment of cells with increasing concentrations of the nanocomposite not only significantly reduced the number of K562 cells but also halted the transition of cells from G1 phase of cell cycle. (B) The results of BrdU assay showed that ZnO/CNT@Fe3O4 could hamper the ability of CML cells to replicate DNA in a concentrations-dependent manner. (C) Results of RQ-PCR analysis demonstrated that treatment of K562 cells with ZnO/CNT@Fe3O4 robustly altered the expression levels of cyclin-dependent kinase inhibitors and c-Myc. Values are given as mean ± standard deviation of three independent experiments. * p ≤.05 represents significant changes from untreated control.
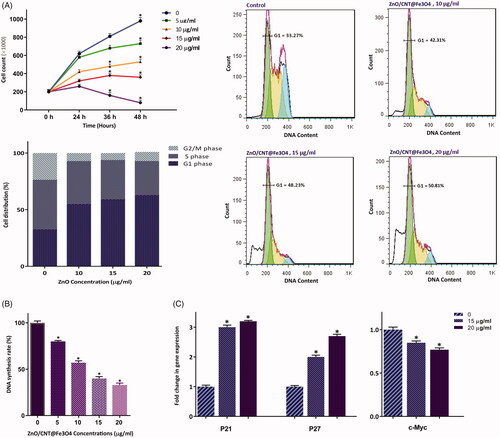
Zno/CNT@Fe3O4 induced apoptosis and increased cell population in Sub-G1 phase
K562 cells were treated with the designated concentrations of the agent and the modulation of phosphatidylserine externalization was examined using FACS analysis. The resulting data showed that ZnO/CNT@Fe3O4 led to a concentration-dependent increase in apoptotic K562 cells as compared with control group, which was in agreement with the increased cell population in Sub-G1 phase (). As a compensatory mechanisms in response to anti-cancer agents, mounting body of evidence has declared that autophagy could be frequently activated in cancer cells during drug treatment [Citation21]. Given this, it was reasonable to evaluate whether inhibition of autophagy using a well-known autophagy inhibitor chloroquine (CQ) could potentiate ZnO/CNT@Fe3O4-induced cytotoxicity in K562 cells. Notably, we found that the inhibition of autophagy, as revealed by the increased acridine fluorescence (), not only resulted in a concentration-dependent cytotoxicity in K562 (), but also exerted a superior cytotoxicity in ZnO/CNT@Fe3O4-treated cells (); indicating that the activation of autophagy could decrease the antileukemic effects of the agent in CML cells.
Figure 4. Evaluation of ZnO/CNT@Fe3O4 effect on induction of apoptosis in K562 cells. ZnO/CNT@Fe3O4 increased cell population in sub-G1 in CML-derived cells. Values are given as mean ± standard deviation of three independent experiments. *p ≤ .05 represents significant changes from untreated control. The percentage of apoptotic cells in nanocomposite-treated group was increased in response to drug treatment in a concentration-dependent manner.
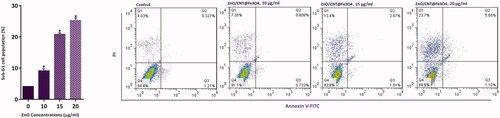
Figure 5. Evaluating the effect of autophagy inhibition on ZnO/CNT@Fe3O4-induced anti-leukemic effect. (A) Treatment of K562 cells with autophagy inhibitor chloroquine (CQ) at the concentration of 40 μM led to a conspicuous reduction in the red-to-green fluorescence intensity ratio. (B) Combinational treatment of CQ (40 μM) with ZnO/CNT@Fe3O4 resulted in superior cytotoxicity as compared with either agent alone. Values are given as mean ± standard deviation of three independent experiments. *p ≤ .05 represents significant changes from untreated control.
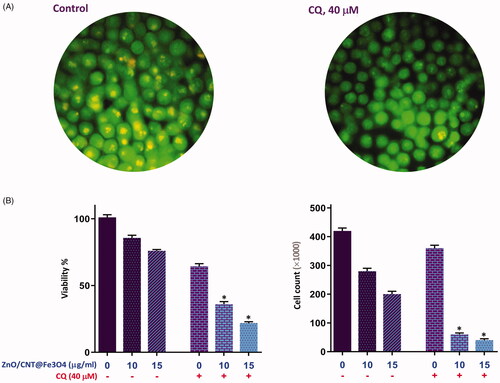
Zno/CNT@Fe3O4 cytotoxicity was coupled with the alteration of apoptosis-related genes
In the light of apoptotic property of the agent, we were tempted to delve into the molecular mechanisms through which ZnO/CNT@Fe3O4 induce its apoptotic effect. In this respect, we evaluated the mRNA expression levels of apoptosis-related genes using qRT-PCR. Our results showed that ZnO/CNT@Fe3O4 induced apoptosis through the alteration in the expressions of both death promoters and death repressors genes, mainly via increasing Bax and Bcl-2 ratio (). Notably, we could find no significant alteration in the mRNA levels of antiapoptotic target genes of the NF-κB pathway such as XIAP and Survivin (). As shown in , suppression of the NF-κB signalling using a well-known proteasome inhibitor (Bortezomib) resulted in an enhanced decrease in cell viability of ZnO/CNT@Fe3O4-treated K562 as compared to either agent alone; indicating that ZnO/CNT@Fe3O4-induced apoptosis is merely mediated through the modulation of the NF-κB pathway.
Figure 6. The effect of ZnO/CNT@Fe3O4 on the transcriptional activity of apoptosis-related genes. (A) After treatment of K562 cells with designated concentrations of the drug and harvesting the RNA, the expression of the indicated genes was measured using Rq-PCR after normalising the cycle threshold (Ct) of each triplicate against their corresponding HPRT. (B) The results of RQ-PCR analysis revealed that single agent of ZnO/CNT@Fe3O4 was unable to alter the expression of the anti-apoptotic members of IAP family, survivin and XIAP, which their expression regulate via NF-κB signalling pathway. Moreover, treatment of K562 cells with a proteasome inhibitor, Bortezomib (BTZ), increased the sensitivity of cells to ZnO/CNT@Fe3O4; suggestive of a contributory role of NF-κB signalling pathway in the cell response to nanocomposite. Values are given as mean ± standard deviation of three independent experiments. *p ≤ .05 represents significant changes from untreated control.
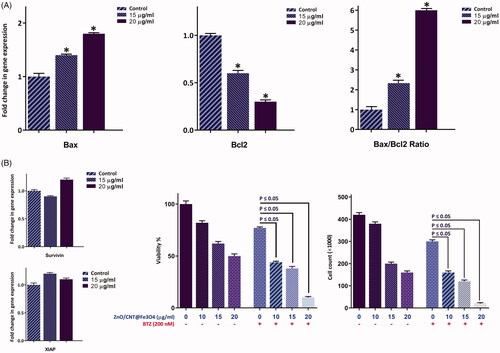
Zno/CNT@Fe3O4-induced apoptosis was coupled with increased ROS and SIRT1 expression
Being a vital product of mitochondria, ROS participates in the regulation of physiological cell signalling; however, excessive production might lead to cell death [Citation22]. It has also been reported that in response to the anti-cancer agents, the production of ROS as well as its associated regulatory genes are increased in malignant cells, which in turn trigger apoptotic cell death [Citation23]. As shown in , the percentage of ROS level in K562 cells was raised in a concentration-dependent manner up to 4.1-fold at the concentration of 20 μg/ml of ZnO/CNT@Fe3O4. In agreement with the up-regulated ROS, we also found that ZnO/CNT@Fe3O4 increased the mRNA expressions of SIRT1 and FOXO3a genes, two potent ROS regulatory genes, as well (). Taken together, it is postulated that ZnO/CNT@Fe3O4-induced apoptosis is mediated, at least partially, through augmentative production of ROS in CML-derived K562 cells.
Figure 7. Treatment of ZnO/CNT@Fe3O4 not only increased the amount of intracellular level of ROS in K562 cells, but also elevated the expression level of SIRT1 and Foxo3a in a concentration-dependent manner. Values are given as mean ± standard deviation of three independent experiments. *p ≤ .05 represents significant changes from untreated control.

Zno/CNT@Fe3O4 enhanced sensitivity of K562 cells to imatinib synergically
Intrigued by the remarkable anti-leukemic effect of ZnO/CNT@Fe3O4, it was of particular interest to determine whether ZnO/CNT@Fe3O4 could potentiate the effectiveness of imatinib, as the standard first-line therapy for CML, in K562 cells. Combinatorial experiments revealed that the combination of ZnO/CNT@Fe3O4 with imatinib was more effective in inhibiting K562 cell survival as compared with imatinib alone (). Next, to investigate whether the interaction between these two agents was synergistic or caused by additive effect, the combination index (CI) was calculated. Determination of both CI and dose reduction index (DRI) values demonstrated that ZnO/CNT@Fe3O4 has the ability to enhance the cytotoxic effect of imatinib ().
Figure 8. Synergistic effect of ZnO/CNT@Fe3O4 with imatinib. After simultaneous treatment of K562 cells with ZnO/CNT@Fe3O4 and imatinib viability and metabolic activity were evaluated using trypan blue exclusion and MTT assays. Combination index (CI) was calculated according to the classic isobologram equation; (Dx)1 and (Dx)2 indicate the individual concentrations of ZnO/CNT@Fe3O4 and imatinib required to inhibit a given level of viability index, and (D)1 and (D)2 are the concentrations of ZnO/CNT@Fe3O4 and imatinib necessary to produce the same effect in combination, respectively. Points above and below the isoeffect line reflect antagonism and synergy, respectively.
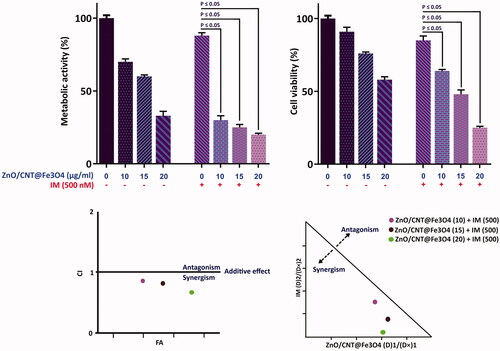
4. Discussion
Engineered nanocomposites are currently under intense investigations due to their vast applicability in various fields of biomedical approaches such as drug and gene delivery, biosensors, and cancer studies [Citation7]. Although multiple preclinical and clinical researches showed extensive potential of nanoparticles (NP) in cancer treatment, further studies are now underway to more precisely examine their molecular mechanisms of action. One major foreseen difficulty with the development and implementation of NPs is their low solubility in water in liquid media [Citation15]. It has therefore been suggested that the best way to increase metal nanoparticle’s solubility is to combine them with water-soluble compounds such as polymers, liposomes, proteins, fatty acids, and inorganic acids [Citation15]. In the present study, we used ZnO QD in combination with hydroxylated carbon nanotubes and Fe3O4 in the form of ZnO/CNT@Fe3O4 nanocomposite to increase water-solubility of our product. Previous studies have declared that owing to their excellent surface properties, CNT are smart nano-carries for the delivery of drugs, genes and other biomedically essential materials into the cells with high biocompatibility [Citation16]. Apart from its inimitable cell penetration aptitude, CNTs have proven to be suitable in specific and targeted drug delivery to desired cancerous tissues [Citation24]. It is worth to mention that the successful formation of ZnO/CNT@Fe3O4 nanocomposite was further characterized using UV-spectroscopy, FTIR, SEM, and XRD.
Our data showed that ZnO/CNT@Fe3O4 significantly decreased both the viability and metabolic activity of CML-derived K562 cells. In consistent, Bisht et al. provided evidence that high doses of ZnO-Fe3O4 magnetic composite nanoparticle induced a cytotoxic effect in human breast cancer cell line (MDA-MB-231) but not in normal mouse fibroblast (NIH 3T3) [Citation25]. Previous studies have demonstrated that the cytotoxicity of metal NPs may be as a result of free radicals and reactive oxygen species (ROS) generation [Citation26,Citation27]. It has also been reported that the excessive ROS can trigger apoptosis through the activation of FOXO3a, which can promote apoptosis signalling by inducing the expression of pro-apoptotic members of the Bcl2 family of mitochondria-targeting proteins [Citation28]. Interestingly, the results of ROS assay revealed that ZnO/CNT@Fe3O4 dramatically increased ROS generation in K562 cell line. Accordingly, we found that not only ZnO/CNT@Fe3O4 increased the mRNA expression level of FOXO3a, but also shifted the ratio of the death promoter to death repressor genes via alteration of Bax and Bcl2 expression level. However, we found no significant changes in the mRNA levels of antiapoptotic target genes of the NF-κB pathway such as Survivin and XIAP; indicating that ZnO/CNT@Fe3O4-induced apoptosis is probably mediated through NF-κB-independent mechanisms. Notably, supplementary investigations revealed that the suppression of NF-κB pathway by using a well-known proteasome inhibitor bortozomib sensitized K562 cells to the cytotoxic effect of ZnO/CNT@Fe3O4; substantiating the fact that the sensitivity of K562 cells to ZnO/CNT@Fe3O4 is probably attenuated by the activation of nuclear factor-κB axis.
It was reported that ROS production could provide a signal that upregulates SIRT1, a member of the NAD+-dependent class III histone deacetylases [Citation29], which in turn regulates cell cycle progression through the induction of G1 or G2/M cell cycle arrest [Citation30]. In agreement with the up-regulated ROS, ZnO/CNT@Fe3O4 increased the expression of SIRT1 and blocked the transition of the cells from G1 phase of the cell cycle by increasing the expression level of p21 and p27 cyclin-dependent kinase inhibitors; indicating that the growth suppressive effect of ZnO/CNT@Fe3O4 was mediated, at least partly, through the induction of G1 arrest. Apart from regulation of cell cycle progression, some reports showed that the expression of SIRT1 in cancer cells may lead to the induction of autophagy [Citation31]. Playing as a double edged sword, induction of autophagy could either trigger cell death or induce a resistance phenotype, according to the cancer cell type [Citation21,Citation32,Citation33]. In a study conducted by Helgason et al., it was reported that the activation of autophagy attenuate the efficacy of imatinib in K562 cells [Citation34]. Consistently, investigating the effects of ZnO/CNT@Fe3O4 in combination with a well-known autophagy inhibitor chloroquine (CQ) revealed that the inhibition of autophagy not only resulted in a concentration-dependent cytotoxicity in K562, but also exerted a superior cytotoxicity in ZnO/CNT@Fe3O4-treated cells; indicating that the antileukemic effects of the agent could be overshadowed, at least partially, through the activation of autophagy in K562 cells.
Intrigued by the remarkable anti-leukemic effect of ZnO/CNT@Fe3O4, we were tempted to investigate whether ZnO/CNT@Fe3O4 could potentiate the effectiveness of Imatinib, as the standard first-line therapy for CML. Combinatorial experiments revealed that the drugs combination produced a synergistic effect and was more effective in inhibiting K562 cell survival as compared with Imatinib alone. Taken together, the present study showed that ZnO/CNT@Fe3O4 had a significant anti-tumor activity against CML-derived K562 cells, either as a single agent or in a combined-modal strategy. We also provided a mechanistic pathway by which ZnO/CNT@Fe3O4 could augment apoptotic cell death probably through a ROS-dependent upregulation of FOXO3a and SIRT1 (); casting new light on the potent application of ZnO/CNT@Fe3O4 in chronic myeloid leukaemia.
Video abstract of the results was provided as a supplementary file.
Figure 9. Schematic representation proposed for the plausible mechanisms of action of ZnO/CNT@Fe3O4 in K562 cells. Treatment of CML-derived cells with metal nanoparticle ZnO/CNT@Fe3O4 augmented the intracellular level of ROS, which in turn induced apoptotic cell death via upregulation of SIRT1 and FOXO3a. However, our results suggest that the effectiveness of this nanoparticle could be diminished, at least partially, through over-activated nuclear factor-κB in K562 cells. More interestingly, we found that Suppression of NF-κB signalling pathway in K562 cells using a well-known proteasome inhibitor, Bortezomib, enhanced the cytotoxic effect of ZnO/CNT@Fe3O4 probably through suppression of the expression level of anti-apoptotic members of IAP family.
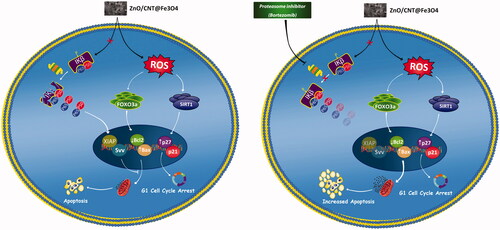
Video_abstract.mp4
Download MP4 Video (3.5 MB)Acknowledgements
Authors would like to express their gratitude to Shahid Beheshti University of Medical Sciences (Tehran, Iran) for supporting this study.
Disclosure statement
No potential conflict of interest was reported by the author(s).
References
- Hughes T. Monitoring CML patients responding to treatment with tyrosine kinase inhibitors: review and recommendations for harmonizing current methodology for detecting BCR-ABL transcripts and kinase domain mutations and for expressing results. Blood. 2006;108(1):28–37.
- Druker BJ, Talpaz M, Resta DJ, et al. Efficacy and safety of a specific inhibitor of the BCR-ABL tyrosine kinase in chronic myeloid leukemia. N Engl J Med. 2001;344(14):1031–1037.
- Zhang X, Tu H, Yang Y, et al. Bone marrow-derived mesenchymal stromal cells promote resistance to tyrosine kinase inhibitors in chronic myeloid leukemia via the IL-7/JAK1/STAT5 pathway. J Biol Chem. 2019;294(32):12167–12179.
- Soverini S, Mancini M, Bavaro L, et al. Chronic myeloid leukemia: the paradigm of targeting oncogenic tyrosine kinase signaling and counteracting resistance for successful cancer therapy. Mol Cancer. 2018;17(1):49.
- Parhi P, Mohanty C, Sahoo SK. Nanotechnology-based combinational drug delivery: an emerging approach for cancer therapy. Drug Discovery Today. 2012;17(17–18):1044–1052.
- Jain K. Nanotechnology-based drug delivery for cancer. Technol Cancer Res Treat. 2005;4(4):407–416.
- Gao Y, Xie J, Chen H, et al. Nanotechnology-based intelligent drug design for cancer metastasis treatment. Biotechnol Adv. 2014;32(4):761–777.
- Cai X, Luo Y, Zhang W, et al. pH-Sensitive ZnO quantum dots–doxorubicin nanoparticles for lung cancer targeted drug delivery. ACS Appl Mater Interfaces. 2016;8(34):22442–22450.
- Vangijzegem T, Stanicki D, Laurent S. Magnetic iron oxide nanoparticles for drug delivery: applications and characteristics. Expert Opin Drug Deliv. 2019;16(1):69–78.
- Bai D-P, Zhang X-F, Zhang G-L, et al. Zinc oxide nanoparticles induce apoptosis and autophagy in human ovarian cancer cells. IJN. 2017;12:6521–6535.
- Wu L, Zhang F, Wei Z, et al. Magnetic delivery of Fe3O4@ polydopamine nanoparticle-loaded natural killer cells suggest a promising anticancer treatment. Biomater Sci. 2018;6(10):2714–2725.
- Hanley C, Layne J, Punnoose A, et al. Preferential killing of cancer cells and activated human T cells using ZnO nanoparticles. Nanotechnology. 2008;19(29):295103.
- Ostrovsky S, Kazimirsky G, Gedanken A, et al. Selective cytotoxic effect of ZnO nanoparticles on glioma cells. Nano Res. 2009;2(11):882–890.
- Khan MI, Mohammad A, Patil G, et al. Induction of ROS, mitochondrial damage and autophagy in lung epithelial cancer cells by iron oxide nanoparticles. Biomaterials. 2012;33(5):1477–1488.
- Ma Y-Y, Ding H, Xiong H-M. Folic acid functionalized ZnO quantum dots for targeted cancer cell imaging. Nanotechnology. 2015;26(30):305702.
- Beg, S, Rahman, M, Jain, A, et al. Emergence in the functionalized carbon nanotubes as smart nanocarriers for drug delivery applications. In Fullerens, Graphenes and Nanotubes. 2018; 105-133. Edited by: Alexandru Mihai Grumezescu. William Andrew Publishing.
- Jmiai A, El Ibrahimi B, Tara A, et al. Application of Zizyphus Lotuse-pulp of Jujube extract as green and promising corrosion inhibitor for copper in acidic medium. J Mol Liq. 2018;268:102–113.
- Wan S, He F, Wu J, et al. Rapid and highly selective removal of lead from water using graphene oxide-hydrated manganese oxide nanocomposites. J Hazard Mater. 2016;314:32–40.
- Cui M, Ren S, Zhao H, et al. Polydopamine coated graphene oxide for anticorrosive reinforcement of water-borne epoxy coating. Chem Eng J. 2018;335:255–266.
- Li M, Liu Q, Jia Z, et al. Electrophoretic deposition and electrochemical behavior of novel graphene oxide-hyaluronic acid-hydroxyapatite nanocomposite coatings. Appl Surf Sci. 2013;284:804–810.
- Sui X, Chen R, Wang Z, et al. Autophagy and chemotherapy resistance: a promising therapeutic target for cancer treatment. Cell Death Dis. 2013;4(10):e838–e838.
- Essers MAG, Weijzen S, de Vries-Smits AMM, et al. FOXO transcription factor activation by oxidative stress mediated by the small GTPase Ral and JNK. Embo J. 2004;23(24):4802–4812.
- Hori YS, Kuno A, Hosoda R, et al. Regulation of FOXOs and p53 by SIRT1 modulators under oxidative stress. PLoS One. 2013;8(9):e73875.
- Mahajan S, Patharkar A, Kuche K, et al. Functionalized carbon nanotubes as emerging delivery system for the treatment of cancer. Int J Pharm. 2018;548(1):540–558.
- Bisht G, Rayamajhi S, Kc B, et al. Synthesis, characterization, and study of in vitro cytotoxicity of ZnO-Fe3O4 magnetic composite nanoparticles in human breast cancer cell line (MDA-MB-231) and mouse fibroblast (NIH 3T3). Nanoscale Res Lett. 2016;11(1):537.
- Zhu X, Zhou J, Cai Z. The toxicity and oxidative stress of TiO2 nanoparticles in marine abalone (Haliotis diversicolor supertexta). Mar Pollut Bull. 2011;63(5–12):334–338.
- Wahab R, Dwivedi S, Umar A, et al. ZnO nanoparticles induce oxidative stress in Cloudman S91 melanoma cancer cells. J Biomed Nanotechnol. 2013;9(3):441–449.
- Hagenbuchner J, Kuznetsov A, Hermann M, et al. FOXO3-induced reactive oxygen species are regulated by BCL2L11 (Bim) and SESN3. J Cell Sci. 2012;125(5):1191–1203.
- Salminen A, Kaarniranta K, Kauppinen A. Crosstalk between oxidative stress and SIRT1: impact on the aging process. IJMS. 2013;14(2):3834–3859.
- He X, Maimaiti M, Jiao Y, et al. Sinomenine induces G1-phase cell cycle arrest and apoptosis in malignant glioma cells via downregulation of sirtuin 1 and induction of p53 acetylation. Technol Cancer Res Treat. 2018;17:153303461877030.
- Lee IH, Cao L, Mostoslavsky R, et al. A role for the NAD-dependent deacetylase Sirt1 in the regulation of autophagy. Proc Natl Acad Sci. 2008;105(9):3374–3379.
- Sheikh‐Zeineddini N, Bashash D, Safaroghli‐Azar A, et al. Suppression of c‐Myc using 10058‐F4 exerts caspase‐3‐dependent apoptosis and intensifies the antileukemic effect of vincristine in pre‐B acute lymphoblastic leukemia cells. J Cell Biochem. 2019;120(8):14004–14016.
- Bashash D, Sayyadi M, Safaroghli-Azar A, et al. Small molecule inhibitor of c-Myc 10058-F4 inhibits proliferation and induces apoptosis in acute leukemia cells, irrespective of PTEN status. Int J Biochem Cell Biol. 2019;108:7–16.
- Helgason G, Mukhopadhyay A, Karvela M, et al. Autophagy in chronic myeloid leukaemia: stem cell survival and implication in therapy. CCDT. 2013;13(7):724–734.