Abstract
Zinc pyrithione (ZPT) is widely used as an antimicrobial. Zinc is a necessary trace element of the human whose homeostasis associated with several cancers. However, the anticancer effect of increased Zinc in ovarian cancer is still unclear. This study focussed on the anti-tumour effects of ZPT combined with Zinc in SKOV3 and SKOV3/DDP cells. The cell viability, apoptosis, migration, and invasion assays were detected by CCK-8, flow cytometry, wound healing and transwell assay, respectively. The distribution of Zinc in cells was monitored by staining of Zinc fluorescent dye and lysosome tracker. The changes in lysosomal membrane stability were reflected by acridine orange fluorescence and cathepsin D reposition. Expression of the proteins about invasion and apoptosis was evaluated by western blot. The results indicated that ZPT combined with Zinc could notably reduce cell viability, inhibit migration and invasion in SKOV3 and SKOV3/DDP cells. Besides, ZPT performed as a Zinc carrier targeted lysosomes, caused the increase of its membrane permeability and the release of cathepsin D accompanied by mitochondrial apoptosis in SKOV3/DDP cells. In conclusion, our work suggests that ZPT combined with Zinc could inhibit proliferation, migration, invasion, and promote apoptosis by trigger the lysosome-mitochondrial apoptosis pathway in ovarian carcinoma.
Introduction
Ovarian cancer is one of the most common cancers in women [Citation1], and many patients are diagnosed at an advanced disease stage. The main strategy for treatment includes debulking surgery and platinum-based chemotherapy [Citation2]. However, the primary and the acquired drug-resistance pose bafflement to clinical therapy, and most patients would suffer treatment failure and unfavourable prognosis [Citation3]. Therefore, it is necessary to find an effective strategy for improving the treatment of ovarian cancer.
Zinc is essential for humans, as it has three leading biological roles: structural, regulatory, and catalyst. Zinc homeostasis also links with cancer development [Citation4]. Increasing evidence has implicated that abnormal Zinc homeostasis plays a vital role in the event of multiple cancers, including prostate [Citation5], pancreatic [Citation6], ovarian cancer [Citation7] and some other cancers [Citation8–10]. For example, some researchers have proved that Zinc level decreased in prostate cancer tissues, which could be detected in the development of prostate cancer [Citation5]. Additionally, complementing Zinc shows an anticancer effect of prostate cancer cells [Citation11] and tissues [Citation12]. Therefore, increasing the concentration of Zinc can be a promising strategy to inhibit the development of cancer cells, especially in the carcinoma of abnormal Zinc deficiency. Therefore, an appropriate and effective Zinc carrier is needed to help this method carry out.
Zinc pyrithione (ZPT) is a 1:2 compound of a Zinc atom and bonding the membrane-permeable ionophore pyrithione. The Food and Drug Administration (FDA) regards it as a worldwide microbicidal agent and generally applied in medicine and cosmetology, including anti-dandruff shampoos because of its antibacterial, antifungal, or antiseborrheic activity [Citation13–15]. Besides, ZPT has been found that it can act as a Zinc ionophore to help transport Zinc across biological membranes and increase the intracellular Zinc ion concentration [Citation16].
Our previous studies have proved that clioquinol could transfer Zinc into cancer cells focussing on lysosome [Citation17,Citation18]. Then we further demonstrated that clioquinol combined with Zinc could inhibit NF-kB activity and leading cancer cells apoptosis [Citation19]. The recent studies found that transferring Zinc into cells could also increase the radiosensitivity of nasopharyngeal cancer stem-like cells [Citation20,Citation21]. However, there were rarely studies to explore the influence of overloaded Zinc on ovarian cancer and the drug-resistant cells. Therefore, we researched the anti-cancer effects of ZPT combined with Zinc in this study. We emphasised the inhibiting effect of overloaded Zinc on the malignancy tumour behaviours and provided a considerable treatment strategy for ovarian cancer.
Materials and methods
Cell lines and cell culture
The human ovarian cancer cell line SKOV3 and the cisplatin-resistant human ovarian cancer subline SKOV3/DDP respectively remained in DMEM, and IMDM media (Hyclone) supplemented with 10% foetal bovine serum (Wisent, Canada) at 37 °C and 5% CO2, cisplatin (0.5 μg/ml; Qilu Pharm., Jinan, China) was added into the medium to maintain chemoresistance. Cells were transferred into cisplatin-free medium two days before experiments.
SKOV3 was obtained from the Shanghai Life Science Institute Cell Library (Shanghai, China), SKOV3/DDP was obtained from the Chinese Academy of Medical Sciences and Peking Union Medical College, Beijing, China. SKOV3/DDP was sub-cloned by exposing SKOV3 to cisplatin with gradually elevated concentration, and last had a resistant index of 4.4 referred to the ratio of the half-maximal inhibitory concentrations (IC50). All cell lines now maintained in the Key Laboratory of Tumour Biological Behaviour of Hubei Province.
Analysis of cell viability
SKOV3 and SKOV3/DDP were all seeded in 96-well plates (7000 cells per well) with 100 μl of the medium, which ensured 40–60% confluence after 24 h of growth. Then cells were treated with ZPT (Sigma Chemical Co, St. Louis, MO) or added with Zinc chloride (Sigma Chemical Co: St. Louis, MO) at various concentrations and growing for designated periods. After that, adding 10 μl CCK-8 (Dojindo, Tokyo, Japan) to incubate for not more than 4 h at 37 °C. The optical density (OD) at 450 nm was measured with a Microplate Reader (SpectraMax Absorbance Reader, America). Cells in the control group were exposed to vehicle (DMSO in DMEM or IMDM supplemented with 10% FBS).
Apoptosis assay
The cells were treated with ZPT, Zinc, or a combination of them for 24 h after overnight attachment. Then collecting all cells of each well for double staining with Annexin V-FITC/propidium iodide (PI) (Annexin V-FITC/PI Apoptosis Kit, Bestbio, China). The percentage of apoptotic cells was measured by flow cytometry (Beckman, USA).
Wound-healing assay
The cells were seeded into 6-well plates, whose density reached about 95% confluence after 24 h growth. The monolayer was lightly scratched by a new 200 μl pipette tip across the well. After scratching, the well was washed with PBS to remove the detached cells and then replenished with serum-free medium with different combinations of drugs for 24 h. Photos were taken at different five views for a well by Shunyu ICX41 inverted microscope (Zhejiang, China). The gap distance was quantitatively evaluated by the software of Image J.
Cell migration and invasion assays
The cells in 200 μl serum-free media were placed into the upper chamber with an eight μm pore membrane (Costar, Corning, NY, USA) for migration assays and invasion assays with Matrigel (Sigma-Aldrich, USA). Media containing 10% FBS (600 μL) was added to the lower chamber. After 24 h incubating, the cells were fixed in 4% paraformaldehyde and stained with crystal violet for 30 min. Then using a cotton swab to remove the cells on the upper surface of the membrane, and counting five random fields at 100 magnifications by using Shunyu ICX41 inverted microscope (Zhejiang, China) in each membrane. Finally, using Image J software (National Institutes of Health) to count cells.
Zinc fluorescence staining and tracking experiment
SKOV3/DDP cells were plated in a 12-well plate with 1 × 105 cells per well. After 24 h incubating, cells were treated with 0.35 μM ZPT or added with 20 μM Zinc for one hour, then stained with 2.5 μM FluoZin-3, AM (Invitrogen) for 30 min and diluted Hoechst33342 (Invitrogen) for 5–10 min in minimum essential medium (IMDM) at room temperature. Next, using the HBSS (Hanks balanced salt solution) to wash cells three times and taking photos by fluorescence microscopy.
For Zinc track and location assay, SKOV3/DDP cells were seeded in Lab-Tek glass-bottom chamber slides (Nunc, Denmark), expect the above treatments, which also dyed with 60 nM lysosome tracker (Invitrogen) for half an hour. After washing with HBSS, Confocal images were obtained by an LSM710 Confocal Live-Cell Imaging System (Leica-LCS-SP8-STED Carl Zeiss, Oberkochen, Germany). FluoZin™-3 was excited at 494 nm, and emission was detected at 516 nm. Lysotracker Red was excited at 543 nm, and emission was detected at 590 nm. Hoechst33342 was excited at 350 nm, and emission resistant at 461 nm. Quantifying of Fluorescent intensities was determined by using the NIS-Elements AR software (Nikon Instruments).
Lysosomal membrane assay
Acridine orange (AO; Sigma-Aldrich, USA) seems as a metachromatic fluorescent dye. It emits red fluorescence when in the intact lysosomes and emits green fluorescence when in the cytosol and the nucleus. Therefore, in the AO stained cells, decreased red fluorescence and elevated green fluorescence could prove the damage of Lysosomal membrane [Citation22]. SKOV3/DDP cells were incubated in the presence of ZPT, Zinc, or both of them for about 4 h, then stained with acridine orange(5 μM, 15 min, 37 °C) to detect the lysosomal integrity. After washed them in the HBSS three times, the cells were observed by an LSM710 Confocal Live-Cell Imaging System with the corresponding filter, 470–490 nm excitation and 515 nm emission wavelengths.
Immunofluorescence staining
The relocated Cathepsin D was examined using immunofluorescence staining. SKOV3/DDP cells were seeded on coverslips and then treated with ZPT, Zinc, or both of them for 8 h. Cells were washed with PBS and fixed in 4% paraformaldehyde at room temperature for 30 min. Next, these cells were permeabilized for 10 min with 0.4% TritonX-100 and blocked by 2% bovine serum albumin (BSA) for one hour. After washed three times with PBS, the cells were incubated in anti-cathepsin D antibody (Proteintech, China) for overnight at 4 °C. Then following fluorescein-conjugated secondary antibody (1:100, Proteintech, China) for 1 h. Hoechst33342 (Invitrogen) was used for staining cell nucleus for 10 min. Finally, the cells were observed by confocal laser scanning microscopy.
Western blotting
After treated for 24 h, the total cellular protein of SKOV3/DDP was extracted with RIPA lysis buffer (P0013B, Beyotime Biotechnology, Shanghai, China) supplemented with 1 mM PMSF (ST506, Beyotime Biotechnology, Shanghai, China). Then, protein extracts were degenerated by boiling with 5 × SDS sample buffer at 100 °C for 10 min. Next, these protein samples were separated by 10% SDS-polyacrylamide gel electrophoresis (SDS-PAGE), transferred to NC membranes (#58060-U, Sigma, America), and incubated with anti-Rabbit antibody against MMP2 (1:300 dilution, #10373-2-AP, Proteintech, Wuhan, China), Vimentin (1:1000 dilution, #10366-1-AP, Proteintech, Wuhan, China), Bcl-2 (1:1000 dilution, #12789-1-AP, Proteintech, Wuhan, China), Bax (1:2000 dilution, #50599-2-lg, Proteintech, Wuhan, China), Caspase-9 (1:2000 dilution, #ab202068, abcam, Shanghai, China), Caspase-3 (1:20,00 dilution, #ab184787, abcam, Shanghai, China), and anti-Mouse antibody against GAPDH (1:50,00 dilution, #60004-1-lg, Proteintech, Wuhan, China), Beta Tubulin (1:20,000 dilution, #10094-1-AP, Proteintech, Wuhan, China) at 4 °C overnight. Subsequently, the membranes were hatched in HRP Goat anti Rabbit IgG(1:10,000 dilution, #ANT020, AntGene, Wuhan, China) or HRP Goat anti Mouse IgG(1:10000 dilution, #ANT019, AntGene, Wuhan, China) for 2 h and visualised with ECL (Advansta, USA) blotting detection reagents in ChemiDoc XRS + system (Bio-Rad, Hercules, CA, USA). The grey value of bands is calculated by Image J and presented as a bar chart by using GraphPad Prism 8 software.
Statistical analysis
Experiments were performed in triplicate unless otherwise stated. Results are expressed as mean ± standard deviation (SD). Experimental comparisons between treatments were made by Student’s t-test or one-way ANOVA. The analysis was made by GraphPad Prism 8 software (San Diego, CA, USA).
Results
The effects of ZPT combined with Zinc on the proliferation of ovarian cancer cells
First, we investigated the effects of ZPT, Zinc, or ZPT combined with Zinc on the proliferation of the ovarian cancer cell lines by CCK8 kit. SKOV3/DDP cells exhibited considerable resistance to cisplatin compared with SKOV3 cells (). The cell lines had dose-dependent effects on the proliferation when treated with various ZPT concentrations (). Moreover, ZPT showed slightly proliferating inhibition under 0.40 μM level. The IC50 values of ZPT on SKOV3 and SKOV3/DDP cells were 0.65 μM and 0.97 μM, respectively. Zinc at the concentration of 50 μM or less exhibited low cytotoxic effects on cells in 48 h from our previous studies and this experiment (data not shown). However, as shown in , After exposure to 40 μM or 20 μM Zinc, the ZPT IC50 value of SKOV3 and SKOV3/DDP cells decreased to 0.35 μM and 0.40 μM levels, respectively. We also found that there was a time-dependent manner when treating with 0.35 μM ZPT and 40 μM Zinc for SKOV3 cells, or 0.35 μM ZPT and 20 μM Zinc for SKOV3/DDP cells ().
Figure 1. Effects of ZPT and Zinc on the proliferation of ovarian cancer cells. (A) Cells were treated with DDP at the concentration increases proportionally for 48 h. (B) Cells were treated with increasing concentrations of ZPT for 48 h. (C) Cells were exposed to a diverse concentration of ZPT and 40 μM Zinc (SKOV3) or 20 μM Zinc (SKOV3/DDP) for 24 h. (D) SKOV3 cells and SKOV3/DDP cultured in 0.35 μM ZPT and 40 μM Zinc (SKOV3) or 20 μM Zinc (SKOV3/DDP) for three days, and the daily changes of cell viability was shown. Results represent the mean ± SD from three independent experiments.
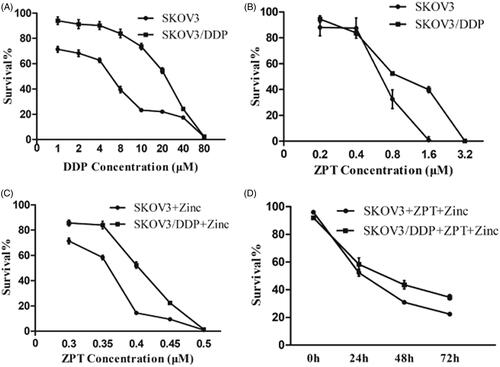
The effects of ZPT combined withZinc on migration and invasion of SKOV3 and SKOV3/DDP cells
We detected the migration and invasion of ovarian cancer cell lines after treated with ZPT combined with Zinc by wound healing and transwell migration and invasion assays. The results of wound healing experiment is shown in (A and B for SKOV3 cells, C and D for SKOV3/DDP cells), which testified that ZPT or Zinc had a slight influence on cell healing distance, while ZPT combined with Zinc dramatically inhibit the distance of wound healing in two cell lines. In addition, the cell numbers of migration and invasion were significantly decreased in the ZPT combined with Zinc group when compared with the other three groups in SKOV3 cell line and SKOV3/DDP cell line in the transwell migration and invasion assays (). To get further insight into the effects of ZPT and Zinc on migration and invasion, we detected the expression of Vimentin and MMP2 in the SKOV3/DDP cell line (). These proteins did not change significantly in the group treated with ZPT or Zinc, but markedly decreased in the group treated with ZPT combined with Zinc when compared to the control group, which suggested that ZPT combined Zinc may inhibit the expression of Vimentin and MMP2.
Figure 2. The functions of ZPT combined with Zinc on the migration and invasion assays in SKOV3 and SKOV3/DDP cells. (A, C) Representative wound closure images of SKOV3 cells (A) and SKOV3/DDP cells (C) after treating of normal medium, 0.35 μM ZPT, 40 μM Zinc (SKOV3) or 20 μM (SKOV3/DDP) Zinc, and 0.35 μM ZPT combined with 40 μM or 20 μM Zinc for 24 h. (B, D) Quantification of the wound width of four groups in SKOV3 (B) and SKOV3/DDP (D) cells. (E) Representative images of SKOV3 migration and invasion behind different treatments for 24 h. (F) Quantitative analysis of migration and invasion cells in SKOV3 cell line. (G) Representative images of migration and invasion assays in SKOV3/DDP cells after planned treatments for 24 h. (H) Quantitative analysis of migration and invasion cells in SKOV3/DDP cell line. (I, J) The representative image and quantified expressions of Vimentin and MMP2 were shown after corresponding treatments for 24 h in SKOV3/DDP cells. Data are expressed as the mean ± SD. **p < .01, ***p < .001. Bar = 40 μm.
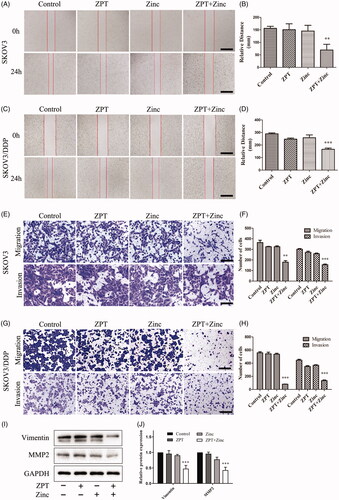
ZPT acted as a Zinc ionophore and introduced Zinc into the lysosomes
The Zinc levels in cells were revealed by staining FluoZin-3-AM (). The intracellular labile Zinc did not significantly increase when SKOV3/DDP cells treated with 0.35 μM ZPT. Likewise, when treated with 20 μM Zinc, the intracellular labile Zinc was slightly increased. However, adding 0.35 μM ZPT combined with 20 μM Zinc into SKOV3/DDP cells induced a significant increase of the intracellular labile Zinc, which suggested that ZPT showed a good ability of Zinc transportation. Next, we examined the subcellular localization of labile Zinc (). We stained SKOV3/DDP cells with FluoZin-3-AM and LysoTracker Red, and found that most of the intracellular labile Zinc colocalized into lysosome with anyone treatment in our groups. These results indicated that ZPT might act as a Zinc ionophore and increased the free Zinc levels, which mostly located in lysosomes.
Figure 3. ZPT induced Zinc accumulating in lysosomes. (A) Fluorescent photomicrographs of FluoZin-3-stained SKOV3/DDP cells were taken after different treatments. From left to right rows: Cells were cultivated in normal medium or treated with 0.35 μM Zinc pyrithione, 20 μM Zinc, 0.35 μM Zinc pyrithione combined with 20 μM Zinc for 1 h in media. Treatment with ZPT combined with Zinc significantly increased intracellular Zinc levels compared with other conditions. (B) Quantification of Zinc fluorescent in four groups of SKOV3/DDP cells. (C) Fluorescent confocal micrographs of FluoZin-3-AM and LysoTracker Red stained SKOV3/DDP cells after treatments. Most intracellular labile Zinc showed apparent overlap with LysoTracker fluorescence. The typical pictures were shown from three independent experiments. ***p < .001. Bar = 40 μm.
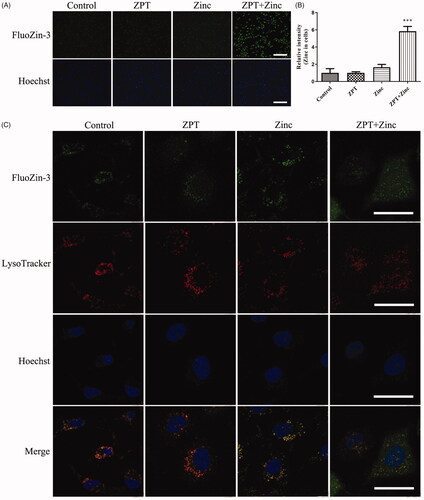
ZPT combined with Zinc increased the lysosomal membrane permeability and the release of cathepsin D
In the above studies, we found that Zinc was transported and accumulated in lysosomes of SKOV3/DDP cells. To investigate the possible influence caused by Zinc on lysosomes, we used acidic organelles to detect lysosomal membrane permeability. After treatment for 4 h, we discovered that the group of ZPT combined with Zinc showed stronger green fluorescence intensity but feebler red fluorescence intensity compared with the untreated group, which may lead to the loss of the pH gradient over lysosomal membranes destabilised (). In addition, in the ZPT combined with Zinc group the lysosomal enzyme cathepsin D displaced from lysosomes to the cytoplasm in SKOV3/DDP cells (). These results indicated the damage of lysosomal membrane integrity caused by ZPT combined with Zinc in SKOV3/DDP cells.
Figure 4. The effects of ZPT combined with Zinc on lysosomal membrane permeability and cathepsin D. (A) Representative images of acridine orange translocation to illustrate the change of lysosomal membrane stability after exposing in 0.35 μM ZPT and 20 μM Zinc for 4 h in SKOV3/DDP cells. (B) Representative images of cathepsin D translocation from lysosomes into the cytoplasm after 8 h treatment of 0.35 μM ZPT and 20 μM Zinc in SKOV3/DDP. Bar = 40 μm.
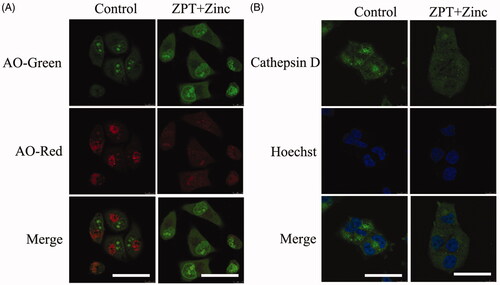
ZPT combined with Zinc promoted the apoptosis of SKOV3 and SKOV3/DDP synergistically
Next, for a much clearer understanding of the joint effect of ZPT and Zinc on apoptosis. we detected the cell apoptotic rate by using Annexin V-FITC/PI Apoptosis Kit and the expression of mitochondrial apoptosis-related proteins. The total apoptosis rate was regarded as the summation of early and late apoptosis rates. As shown in , the rate of apoptosis was 12.08% and 11.47% in SKOV3 cells after treated with ZPT or Zinc for 24 h, respectively. Whereas the apoptosis rate was considerably increased to 29.95% when cultured with ZPT combined with Zinc. Similarly, we detected the combination of ZPT and Zinc could induce a higher apoptosis rate of 26.20% compared with treating with ZPT (15.92%) or Zinc (16.63%) in SKOV3/DDP cells (). The change of the apoptosis rates of SKOV3 and SKOV3/DDP cells could be seen clearly in .
Figure 5. Effects of ZPT and Zinc on the mitochondrial apoptosis of ovarian cancer cells. (A) SKOV3 cells were treated by normal medium, 0.35 μM ZPT, 40 μM Zinc, 0.35 μM ZPT combined with 40um Zinc for 24 h. (B) SKOV3/DDP cells were dealt with normal medium, 0.35 μM ZPT, 20 μM Zinc, 0.35 μM ZPT combined with 20 μM Zinc for 24 h. Representative data of apoptosis analyses labelling by Annexin V-FITC and PI were shown. (C) The apoptosis rates in SKOV3 and SKOV3/DDP cells were shown in the bar chart. (D, E) The representative image and quantified expression of the mitochondrial apoptosis related proteins were displayed after different treatments in SKOV3/DDP cells. *p < .05, **p < .01, ***p < .001.
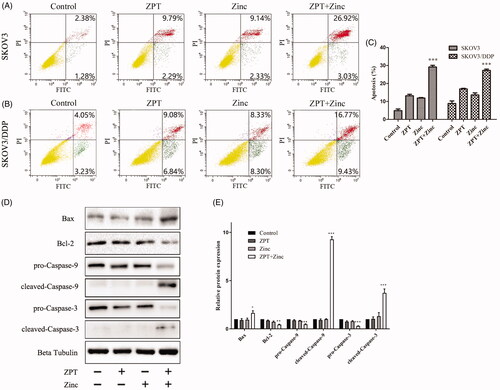
The Bcl-2 family proteins are considered closely relate to mitochondrial apoptosis, contain both pro-apoptosis and anti-apoptosis proteins whose unbalance would lead the activation of caspase. To investigate the mitochondrial apoptosis induced by the combination of ZPT and Zinc in SKOV3/DDP cells, the proapoptotic protein Bax, the antiapoptotic protein Bcl-2, the apoptosis initiator caspase-9 and the effector caspase-3 were detected. As shown in . The results indicated that ZPT combined with Zinc could dramatically promote the Bax level and downregulate the Bcl-2 level, and the caspase-9 and caspase-3 were activated shown as an elevation of cleaved caspase-9 and caspase-3 contrasted with the other groups. Therefore, these results suggested that mitochondrial pathway played a vital role in the apoptosis induced by ZPT combined with Zinc in SKOV3/DDP cells.
Discussion
Ovarian cancer is one of the dreadful threatens to women. Platinum-based chemotherapy is the mainstream treatment for advanced ovarian cancer. However, the 5-year survival rate still climbs slightly, one unavoidable reason is platinum chemotherapy resistance [Citation23,Citation24]. Therefore, a new treatment strategy to help eliminate ovarian cancer should be brought to the forefront. In this study, we first explored the influence caused by Zinc and its carrier-ZPT on ovarian cancer cells and ovarian cisplatin resistance cells. We found that transporting Zinc into cells through ZPT resulted in suppressing several malignant phenotypes of SKOV3 and SKOV3/DDP cells, including proliferation, migration, invasion, and inducing lysosomes-mitochondria apoptosis.
Zinc is an integrant element and a necessary structural composition of various proteins, such as cytokines, enzymes, and transcription factors [Citation25]. Therefore, if the balance of Zinc homeostasis is broken, many kinds of diseases including malignant tumour would occur [Citation26,Citation27]. It also has been known that Zinc level of serum and tissues is notably lower in ovarian tumour patients than in controls [Citation7,Citation28–30]. However, to our knowledge, there was rare article to explore if an increased Zinc level in ovarian cancer can affect tumour cell survival. In our study, we observed that ZPT combined with Zinc in an appropriate dosage exerted cytotoxicity on SKOV3 and SKOV3/DDP cells. These results indicate that ZPT combined Zinc may be a promising treatment to block the survival of ovarian cancer cells.
The ability of tumour cells to invade and migrate is related to its aggressiveness and metastasis. The change of Zinc level in cells can have an influence on MMPs expression and activation, which considered relevant to tumour metastasis [Citation31]. It has been reported that elevated Zinc levels could inhibit prostate cancer cell invasion and metastasis by restraining the proteolytic activity of PSA [Citation32]. In another study, Zinc was made of nanofibers, which have a negative function on inflammation nuclear factor-kappa B signalling, and finally can suppress migration and invasion of tumour cells [Citation33]. In our study, the date indicated that the combination of ZPT with Zinc resulted in a decrease of cancer cell migration and invasion efficiencies, compared with the untreated group and single drug groups in SKOV3 cells and SKOV3/DDP cells. Furthermore, the analysis of the expression of Vimentin and MMP2 supported the above hypothesis because these indexes are closely related to the migratory and invasive phenotype [Citation34,Citation35]. Therefore, these results indicated that ZPT combined with Zinc could be a considerable choice to inhibit migration and invasion during tumour progression.
The activation of the antiapoptotic defense plays a vital role in drug resistance [Citation36,Citation37]. Lysosomal destabilization is considered to relate to cell apoptosis in a mitochondrion-dependent way [Citation38,Citation39]. Some researches reported that Zinc could induce mitochondrial apoptosis in several kinds of cancer [Citation40–42]. However, there is no consistent conclusion about the subcellular localization of supplementary Zinc in the cells. Some studies suggested that Zinc targeted in mitochondria [Citation43,Citation44], while other researches advocated that Zinc could trigger apoptosis by accumulating Zinc into the lysosome [Citation45,Citation46]. In our study, in accordance, we observed that ZPT transported Zinc mostly into lysosomes, accumulated Zinc could cause the lysosomal membrane permeabilization (LMP) and consequent cathepsin D distribution. Besides, the elevated apoptosis rate, increased ratio of Bax/Bcl2 and the activation of caspase-9 and caspase-3 in the ZPT combined with Zinc group compared with the other three groups, all of which could suggest the operation of mitochondrial apoptosis in ovarian cancer cells. Therefore, these results illustrate that ZPT combined with Zinc could sequester the increased Zinc into lysosomes and cause lysosome membrane destabilization, following the mitochondrial apoptosis, which suggest a lysosome-mitochondrial apoptosis pathway may be involved in the treatment of ZPT combined with Zinc in ovarian cancer cells.
One critical aspect of our study is that SKOV3/DDP needs lesser Zinc than SKOV3 when combined with 0.35 μM ZPT to obtain similar effects. The majority of studies are dedicated to the use of Zinc complexes or combined Zinc to reverse chemoresistance [Citation47,Citation48]. And a gradually clear association between metal and antibiotic that metal (including Zinc) promotes the metal–antibiotic coresistance because it could select resistance genes for these two agents [Citation18]. However, it is still unclear whether Zinc resistance is consistent with chemotherapeutics resistance in cancer cells. In this article, our study found that the sensibility of Zinc on SKOV3/DDP is much higher than SKOV3, indicates the resistibility of Zinc and chemotherapeutic drugs may be contrary or have no relation. Certainly, it still needs more research about the status of other resistant cells and the possible mechanisms involved to know whether Zinc is related to chemotherapy resistance, if there is a correlation, is it consistent or vice versa? We suppose this might provide a novel strategy to reverse the chemoresistance of cancer.
Conclusion
In summary, our study suggests that increased Zinc levels in cells may be an appropriate strategy for the treatment of ovarian cancer. Our results revealed that ZPT combined with Zinc has a remarkable inhibiting effect on proliferation, migration, invasion, and inducing lysosome-mitochondrial apoptosis. Therefore, increasing Zinc concentration may be a promising way to help to treat ovarian cancer, which warrants further validation in prospective studies.
Disclosure statement
No potential conflict of interest was reported by the author(s).
Additional information
Funding
References
- Bowtell DD. The genesis and evolution of high-grade serous ovarian cancer. Nat Rev Cancer. 2010;10(11):803–808.
- Cheng X, Wei L, Huang X, et al. Solute carrier family 39 member 6 gene promotes aggressiveness of esophageal carcinoma cells by increasing intracellular levels of zinc, activating phosphatidylinositol 3-kinase signaling, and up-regulating genes that regulate metastasis. Gastroenterology. 2017;152(8):1985–1997.e1912.
- Matulonis UA, Sood AK, Fallowfield L, et al. Ovarian cancer. Nat Rev Dis Primers. 2016;2:16061.
- Costello LC, Franklin RB. Decreased zinc in the development and progression of malignancy: an important common relationship and potential for prevention and treatment of carcinomas. Expert Opin Ther Targets. 2017;21(1):51–66.
- Clavijo Jordan MV, Lo ST, Chen S, et al. Zinc-sensitive MRI contrast agent detects differential release of Zn(II) ions from the healthy vs. malignant mouse prostate. Proc Natl Acad Sci USA. 2016;113(37):E5464–5471.
- Li M, Zhang Y, Liu Z, et al. Aberrant expression of zinc transporter ZIP4 (SLC39A4) significantly contributes to human pancreatic cancer pathogenesis and progression. Proc Natl Acad Sci USA. 2007;104(47):18636–18641.
- Gal D, Lischinsky S, Friedman M, et al. Prediction of the presence of ovarian cancer at surgery by an immunochemical panel: CA 125 and copper-to-zinc ratio. Gynecol Oncol. 1989;35(2):246–250.
- Ressnerova A, Raudenska M, Holubova M, et al. Zinc and copper homeostasis in head and neck cancer: review and meta-analysis. Curr Med Chem. 2016;23(13):1304–1330.
- Fong LY, Jiang Y, Farber JL. Zinc deficiency potentiates induction and progression of lingual and esophageal tumors in p53-deficient mice. Carcinogenesis. 2006;27(7):1489–1496.
- Liaw KY, Lee PH, Wu FC, et al. Zinc, copper, and superoxide dismutase in hepatocellular carcinoma. Am J Gastroenterol. 1997;92(12):2260–2263.
- Uzzo RG, Crispen PL, Golovine K, et al. Diverse effects of zinc on NF-kappaB and AP-1 transcription factors: implications for prostate cancer progression. Carcinogenesis. 2006;27(10):1980–1990.
- Tao M, Xu K, He S, et al. Zinc-ion-mediated self-assembly of forky peptides for prostate cancer-specific drug delivery. Chem Commun (Camb). 2018;54(37):4673–4676.
- Bailey P, Arrowsmith C, Darling K, et al. A double-blind randomized vehicle-controlled clinical trial investigating the effect of ZnPTO dose on the scalp vs. antidandruff efficacy and antimycotic activity. Int J Cosmet Sci. 2003;25(4):183–188.
- Mo J, Lin D, Wang J, et al. Apoptosis in HepG2 cells induced by zinc pyrithione via mitochondrial dysfunction pathway: involvement of zinc accumulation and oxidative stress. Ecotoxicol Environ Saf. 2018;161:515–525.
- Mills KJ, Hu P, Henry J, et al. Dandruff/seborrhoeic dermatitis is characterized by an inflammatory genomic signature and possible immune dysfunction: transcriptional analysis of the condition and treatment effects of zinc pyrithione. Br J Dermatol. 2012;166 Suppl 2(Suppl 2):33–40.
- Ding WQ, Lind SE. Metal ionophores – an emerging class of anticancer drugs. IUBMB Life. 2009;61(11):1013–1018.
- Yu H, Zhou Y, Lind SE, et al. Clioquinol targets zinc to lysosomes in human cancer cells. Biochem J. 2009;417(1):133–139.
- Poole K. At the nexus of antibiotics and metals: the impact of Cu and Zn on antibiotic activity and resistance. Trends Microbiol. 2017;25(10):820–832.
- Yu H, Lou JR, Ding WQ. Clioquinol independently targets NF-kappaB and lysosome pathways in human cancer cells. Anticancer Res. 2010;30(6):2087–2092.
- Lu S, Ke Y, Wu C, et al. Radiosensitization of clioquinol and zinc in human cancer cell lines. BMC Cancer. 2018;18(1):448.
- Ke Y, Li Y, Zeng Y, et al. Radiosensitization of clioquinol combined with zinc in the nasopharyngeal cancer stem-like cells by inhibiting autophagy in vitro and in vivo. Int J Radiat Oncol Biol Phys. 2018;102(3):e160.
- Boya P, Kroemer G. Lysosomal membrane permeabilization in cell death. Oncogene. 2008; 27(50):6434–6451.
- Holmes D. Ovarian cancer: beyond resistance. Nature. 2015;527(7579):S217.
- The L. UKCTOCS and the evaluation of screening for ovarian cancer. Lancet. 2016;387(10022):918.
- Fukada T, Yamasaki S, Nishida K, et al. Zinc homeostasis and signaling in health and diseases: Zinc signaling. J Biol Inorg Chem. 2011;16(7):1123–1134.
- Kolenko V, Teper E, Kutikov A, et al. Zinc and zinc transporters in prostate carcinogenesis. Nat Rev Urol. 2013;10(4):219–226.
- McQuitty JT, Jr., DeWys WD, Monaco L, et al. Inhibition of tumor growth by dietary zinc deficiency. Cancer Res. 1970;30(5):1387–1390.
- Jiang WG, Sanders AJ, Katoh M, et al. Tissue invasion and metastasis: molecular, biological and clinical perspectives. Semin Cancer Biol. 2015;35 Suppl:S244–s275.
- Lightman A, Brandes JM, Binur N, et al. Use of the serum copper/zinc ratio in the differential diagnosis of ovarian malignancy. Clin Chem. 1986;32(1 Pt 1):101–103.
- Alam S, Kelleher SL. Cellular mechanisms of zinc dysregulation: a perspective on zinc homeostasis as an etiological factor in the development and progression of breast cancer. Nutrients. 2012;4(8):875–903.
- Klein T, Bischoff R. Physiology and pathophysiology of matrix metalloproteases. Amino Acids. 2011;41(2):271–290.
- Ishii K, Otsuka T, Iguchi K, et al. Evidence that the prostate-specific antigen (PSA)/Zn2+ axis may play a role in human prostate cancer cell invasion. Cancer Lett. 2004;207(1):79–87.
- Xin Q, Zhang H, Liu Q, et al. Extracellular biocoordinated zinc nanofibers inhibit malignant characteristics of cancer cell. Nano Lett. 2015;15(10):6490–6493.
- Winerdal ME, Krantz D, Hartana CA, et al. Urinary bladder cancer tregs suppress MMP2 and potentially regulate invasiveness. Cancer Immunol Res. 2018;6(5):528–538.
- Yang D, Sun Y, Hu L, et al. Integrated analyses identify a master microRNA regulatory network for the mesenchymal subtype in serous ovarian cancer. Cancer Cell. 2013;23(2):186–199.
- Pommier Y, Sordet O, Antony S, et al. Apoptosis defects and chemotherapy resistance: molecular interaction maps and networks. Oncogene. 2004;23(16):2934–2949.
- Kartal-Yandim M, Adan-Gokbulut A, Baran Y. Molecular mechanisms of drug resistance and its reversal in cancer. Crit Rev Biotechnol. 2016;36(4):716–726.
- Vandenabeele P, Galluzzi L, Vanden Berghe T, et al. Molecular mechanisms of necroptosis: an ordered cellular explosion. Nat Rev Mol Cell Biol. 2010;11(10):700–714.
- Boya P, Andreau K, Poncet D, et al. Lysosomal membrane permeabilization induces cell death in a mitochondrion-dependent fashion. J Exp Med. 2003;197(10):1323–1334.
- Zhou X, Li Y, Li C. Autophagy plays a positive role in zinc-induced apoptosis in intestinal porcine epithelial cells. Toxicol in Vitro. 2017;44:392–402.
- Kocdor H, Ates H, Aydin S, et al. Zinc supplementation induces apoptosis and enhances antitumor efficacy of docetaxel in non-small-cell lung cancer. Drug Des Devel Ther. 2015;9:3899–3909.
- Eron SJ, MacPherson DJ, Dagbay KB, et al. Multiple mechanisms of zinc-mediated inhibition for the apoptotic caspases-3, -6, -7, and -8. ACS Chem Biol. 2018;13(5):1279–1290.
- Zhang X, Guan T, Yang B, et al. A novel role for zinc transporter 8 in the facilitation of zinc accumulation and regulation of testosterone synthesis in Leydig cells of human and mouse testicles. Metab Clin Exp. 2018;88:40–50.
- Ning P, Jiang J, Li L, et al. A mitochondria-targeted ratiometric two-photon fluorescent probe for biological zinc ions detection. Biosens Bioelectron. 2016;77:921–927.
- Wiggins HL, Wymant JM, Solfa F, et al. Disulfiram-induced cytotoxicity and endo-lysosomal sequestration of zinc in breast cancer cells. Biochem Pharmacol. 2015;93(3):332–342.
- Abuarab N, Munsey TS, Jiang LH, et al. High glucose-induced ROS activates TRPM2 to trigger lysosomal membrane permeabilization and Zn-mediated mitochondrial fission. Sci Signal. 2017;10(490):eaal4161.
- Arriaga JM, Greco A, Mordoh J, et al. Metallothionein 1G and zinc sensitize human colorectal cancer cells to chemotherapy. Mol Cancer Ther. 2014;13(5):1369–1381.
- Wu N, Zhang C, Wang C, et al. Zinc-doped copper oxide nanocomposites reverse temozolomide resistance in glioblastoma by inhibiting AKT and ERK1/2. Nanomedicine (Lond)). 2018;13(11):1303–1318.