Abstract
Ovarian cancer is one of the deadliest gynecological cancer, with a low overall 5-year survival rate. RDM1, RAD52 motif-containing protein 1, is sensitive to cisplatin, a common chemotherapy drug and it has an important role inDNA damage repair pathway. Until now, the effect of RDM1 in ovarian cancer is undiscovered. Here, clinical data shows that the tumour tissues of ovarian carcinoma patients with higher mRNA and protein expression of RDM1. Knockdown of RDM1 in ovarian carcinoma cells reduces cell proliferation and promotes apoptosis, consistent with the role RDM1 in the overexpression experiments. The research of xenograft mouse model shows stable knockdown of RDM1 significantly inhibits ovarian cancer tumour growth. These in vitro and in vivo results conclude that RDM1 plays an oncogenic role in human ovarian carcinoma. Interestingly, p53/RAD51/RAD52 signalling pathway can be regulated by RDM1, and the negative regulation of p53 by RDM1 may be one of major mechanisms for RDM1 to accomplish its oncogenic functions in ovarian carcinoma. Therefore, RDM1 may be a new target for the treatment of ovarian carcinoma.
Keywords:
Introduction
Ovarian cancer is a common cancer with high degree of malignancy in females, and the 5-year survival rate is only 25–35% [Citation1]. The pathogenesis of ovarian cancer is complex and the recurrence rate is high. Current treatments of ovarian cancer include radiotherapy and chemotherapy combined with debunking surgery. Although the treatment of ovarian cancer has made obvious progress, its long-term prognosis is poor [Citation2]. The high rate of relapse and chemo-resistance make the management of ovarian cancer particularly challenging [Citation3]. Therefore, understanding the pathogenesis of ovarian cancer can provide a theoretical basis for clinical treatment.
Cancer incidence can be significantly increased by DNA damage, dysfunction of DNA or disruption of genomic integrity. DNA damage repair can significantly restore the damage of DNA caused by factors such as the chemotherapeutic drug cisplatin, which increases the resistance of cancer cells to anticancer drugs. As drug resistance caused by cisplatin chemotherapy becomes more and more common, it is urgent to study the key factors in DNA damage repair pathways in cancer therapy. RDM1 (RAD52 motif-containing protein 1, RDM1) plays a role in damage repair and recombination of DNA double-strand fractures. Same with RAD52, the key regulator of DNA recombination repair, the RD motif RDM1 is similar in function to the N-terminal region of RAD52 [Citation4–7]. It is important that the cells without RDM1 expression exhibited the increased sensitivity to cisplatin [Citation8]. And here’s an interesting finding that the analysis of gene expression of ovarian patients from the cancer genome atlas (TCGA) tells us RDM1 as one of the significantly up-regulated genes in human ovarian cancer. However, even with these findings, the role of RDM1 in human cancer is still poorly understood.
In this study, we found that RDM1 were over-expressed in human ovarian cancer samples both at the mRNA and protein levels. Significantly, the RDM1 mRNA level of human ovarian cancer tumours was higher than that normal ovarian tissues, as analysed from TCGA database. We elected two kinds of ovarian carcinoma cell lines, H8910 and SKOV3, and then knocked down or overexpressed RDM1 in these two cells to detect some cancer-related phenotypes, such as cell proliferation and apoptosis. We further evaluated the in vivo tumour growth of the RDM1 stable knockdown cells in a mouse xenograft model. The current results support that RDM1 plays an oncogenic role in human ovarian cancer, supporting by the observation that RDM1 down-regulated the expression of p53 at mRNA and protein levels. Our study revealed that RDM1 has an oncogenic function in human ovarian cancer.
Materials and methods
Reagents
Antibodies were purchased from proteintech (RDM1), Abcam (RAD51/RAD52), Sigma (β-actin), Santa Cruz (p53) and Cell Signalling Technology (IR DyeR 800 goat anti-Mouse, IR DyeR 800 goat anti-Rabbit).
Cell culture
Human ovarian carcinoma cell lines H8910 and SKOV3 were obtained from ATCC. Cells were cultured in RPMI 1640 medium supplemented with 2 mM L-glutamine, 10% foetal bovine serum (FBS), 100 U/ml penicillin, and 100 mg/ml streptomycin. Cell cultures were maintained in 37 °C and humidified atmosphere consisting 5% CO2.
Analysis of human ovarian cancer tumour samples
Ovarian cancer tumours of human patients were collected from Shanghai International Peace Maternity and Child Health Hospital. Collection protocols required that all participating patients have read and signed consent agreement. The sections were stained with specific antibodies and counterstained with haematoxylin. The staining intensity by specific antibodies was evaluated as negative (–), weak staining (+), moderate/strong staining (+ +) and very strong staining (+ + +) and quantified as described [Citation9].
RNA interference and real time quantitative RT-PCR
The sequence of siRNA against RDM1 is 5′-GCACCAGACAUAAGGCAGUTT-3′ and shRNA against RDM1 is 5′-GCGAAUUACUACUUUGGUUTT-3′. The specificity of RDM1 siRNA or shRNA sequences was confirmed by BLAST search against the human genome database. siControl-RNA was purchased from Shanghai GenePharma company. Cells were homogenised in 1 ml RNAisoTM Plus lysis buffer (TAKARA). Total RNA was extracted and 2 mg RNA was transcribed into cDNA with M-MLV reverse transcriptase (Invitrogen) following the manufacturer’s instruction. The gene-specific primers are as follows:
RDM1 forward: 5′-GCCCATCCTGGTTTCTATGCCC-3′;
RDM1 reverse: 5′-AGACGAACCTTGACTGGAGAT-3′;
RAD51 forward: 5′-CAGTGATGTCCTGGATAATGTAGC-3′;
RAD51 reverse: 5′-TTACCACTGCTACACCAAACTCAT-3′;
RAD52 forward: 5′-TCAAGTACCGCGTGAAACCA-3′;
RAD52 reverse: 5′-CGATCTTTGTTGCGGAACGG-3′;
P53 forward: 5′-GGCAGACTTTTCGCCACAG-3′;
p53 reverse: 5′-CAGGCACAAACACGAACCTC-3′;
β-actin forward: 5′-CGTCATACTCCTGCTTGCTG-3′;
β-actin reverse: 5′- GTACGCCAACACAGTGCTG-3′.
Overexpression of RDM1
pcDNA3.1 vector was used to express human RDM1 cDNA. 1 μg pcDNA3.1-RDM1 or pcDNA3.1-vector were transfected into H8910 and SKOV3 cells by Lipofectamine 2000 (Thermo Fisher Scientific).
Cell proliferation analysis
Cell proliferation was detected by MTT assay and clone formation assay. MTT assay was performed by seeding 2 × 103 cells in a 96-well plate and cultured for 0 h, 24 h, 48 h, 72 h, 96 h. Then cells were incubated with MTT detection solution at 37 °C for 4 h and terminated by DMSO. Absorbance (490 nm) was measured and analysed.
Clonogenic assay was carried out by inoculating cells onto 6-well board at a density of 1000 cells per well and cultured for 7 days. Then cells were stained with crystal violet and the colony number of each group was observed under microscope and counted with ImageJ software.
Analysis of apoptosis
Double-staining of Annexin V-FITC-Propidium iodide (PI) was used for analysing apoptosis. Cells were collected and washed by cold PBS, and then re-suspended at a density of 1 × 106 cells/mL. Annexin V-Alexa Fluor 488 conjugate was added into the cell suspensions (100 µg/mL), followed by incubation at room temperature for 10 min. Next, the PI (100 µg/mL) was added into the cell suspensions and incubated for 5 min at room temperature. Finally, cell apoptosis was analysed by flow cytometry with the parameters of 494/518 nm set for Annexin V channel and 535/617 nm for PI.
Mouse xenograft tumour model
H8910 shN and shRDM1 cells were injected into 4-6 weeks old BALB/c nude mice by subcutaneously inoculating with xenografts of 2 × 106 cells in 100 μl per spot. Animal studies were conducted under specific pathogen free (SPF) conditions and experimental mice were handled according to the ethical and scientific standards by the Animal Centre at the institute following procedures approved by the Institutional Animal Care and Use Committee. Mice were continuously monitored for 6 weeks so the tumour size and weight were recorded. End-point mice were euthanized by CO2 asphyxiation, followed by removal of tumours. IHC was performed after the samples were embedded in paraffin, sectioned, and stained with haematoxylin and eosin (H&E) or IHC antibodies.
Western blotting analysis
The cells were lysed in SDS sample buffer and resolved in 10% gradient SDS-PAGE gels. Separated proteins were transferred to nitrocellulose membranes and immunoblotted with primary antibodies specific for RDM1, p53, RAD51, RAD52 or β-actin (1:1000–1:2000 dilutions) overnight at 4 °C. After incubation with a fluorescent-labelled secondary antibody (1:5000 dilutions), specific signals for proteins were visualised by a LI-COR Odyssey Infra-red Imaging System.
Immunohistochemistry (IHC)
Anonymous human normal ovary tissues, human ovarian tumour tissues or mouse xenografts ovarian tumour tissues were fixed with 4% paraformaldehyde for 12 h, transferred into gradient ethanol, rolled, processed and embedded into paraffin. Tumours were cut in 4 mm sections with a microtome (Leica, Germany) followed by immunohistochemistry as described [Citation10].
Statistical analysis
Student’s t-tests were performed to obtain the statistical significance. A p value < .05 was considered significant.
Results
RDM1 is up-regulated in human ovarian carcinoma tumours
Recently, some new works have revealed that RDM1 has high expression level in papillary thyroid carcinoma and lung adenocarcinoma [Citation11–13]. However, the expression of RDM1 in ovarian cancer remains to be explored. We therefore analysed the cancer genome atlas (TCGA) database to verify the RDM1 expression levels in human ovarian carcinoma tumour tissues and normal ovarian tissues (). Interestingly, RDM1 is significantly higher expressed in ovarian carcinoma tumour tissues than the normal ovarian tissues (). Consistent with this results, the immunohistochemistry (IHC) analysis data showed the protein level expression of RDM1 in human ovarian tumour in increased compared to that of normal ovary ().
Figure 1. RDM1 is up-regulated in human ovarian carcinoma tumours. (A) The RDM1 gene expression information from ovarian cancer patients in The Cancer Genome Atlas (TCGA). T: ovarian tumour tissue, n = 426; N: normal ovarian tissue, n = 88. Data are expressed as mean ± SD. *p < .05. (B) Representative Immunohistochemistry (IHC) images of RDM1 in normal and ovarian tumour tissues of human patients. (C) Summary and statistical analysis of experiments in (B) with human normal ovary (n = 7) and ovarian tumour tissues (n = 9). Scale bar, 25 μm (magnification, ×40).
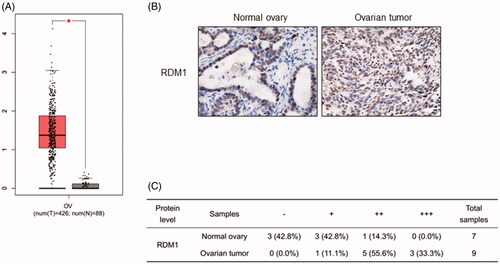
RDM1 positively regulates human ovarian carcinoma cell growth
Base on the results that RDM1 was up-regulated in human ovarian carcinoma tumour, the cell proliferation was detected with or without RDM1 regulation firstly. The two kinds of ovarian carcinoma cell lines, H8910 and SKOV3, were used to do the in vitro experiments. The knockdown efficiency was confirmed by qRT-PCR showing that the mRNA levels of RDM1 were significantly inhibited after the transfection of siRNA-RDM1 (siRDM1) compared with that of siRNA-Control (siN) (). Obviously, for both two cell lines, siRDM1 cells grew significantly slower than siN cells at later time points (i.e. 96 h, p < .05) (). These results were further confirmed by clonogenic assay at 72 h under RDM1 knockdown condition () or overexpression condition (). These results show that cell proliferation of ovarian carcinoma cells can be regulated by RDM1 positively.
Figure 2. RDM1 positively regulates ovarian carcinoma cell growth. (A) The inhibition efficiency of RDM1 gene expression was evaluated by qRT-PCR in H8910 and SKOV3 cells. (B) Normal control group and knockdown group of H8910 and SKOV3 cells were examined for cell proliferation ability by MTT assay. (C and D) Clonogenic assay for H8910 (C) and SKOV3 (D) cells at Day 7 after plating the cells with transfection of normal control or RDM1 siRNAs. (E) RDM1 Overexpression efficiency was evaluated by qRT-PCR in H8910 and SKOV3 cells which were transfected 1 μg plasmid of RDM1 or vector. (F) Clonogenic assay for H8910 and SKOV3 cell at Day 7 after plating the cells with normal control or overexpression of RDM1. Data represent means ± s.e.m.; *, p < .05; **, p < .01; ***, p < .001 as determined by Student’s t test. All experiments were repeated at least three times.
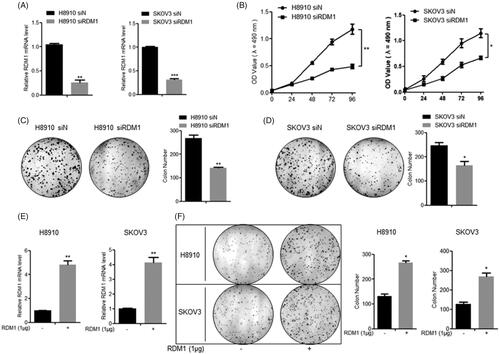
Knockdown of RDM1 inhibits human ovarian carcinoma cell growth in vivo
In order to further investigate the carcinogenic role of RDM1 in ovarian cancer in vivo, we silenced RDM1 with specific short hairpin RNA (shRNA-RDM1) in H8910 cells to get the stable knockdown cell line named H8910 shRDM1, and the control cell line named H8910 shN (). After that, the H8910 shN and shRDM1 cells were severally injected into a quantity of 6-week old immunocompromised nude mouse. At the same time to sacrifice those mouse, and recorded the size and weight of tumours. The results show that the tumours of shRDM1 group mouse were significantly smaller and lighter than the tumours of the control group, confirming the carcinogenic in vivo role of RDM1 in ovarian cancer (). RDM1 protein expression level was detected by IHC staining assay in order to confirm the knockdown efficiency of RDM1 in the shRDM1 tumours (). From the above, knockdown of RDM1 inhibits ovarian carcinoma cell growth in vivo.
Figure 3. Knockdown of RDM1 inhibits human ovarian carcinoma cell growth in vivo. (A) The stable RDM1-knockdown (shRDM1) and control (shN) of H8910 cell line was constructed by short hairpin RNA (shRNA) and then analysed the expression of RDM1 by immunoblotting with the antibodies against RDM1. (B) H8910 shN and shRDM1 cells were injected subcutaneously into 4-6 weeks old immunocompromised mice. When tumour growth enough, they were removed, taken photos and measured tumours’ weights. (C) The weights of tumours are presented as Mean ± SD (n = 6). (D) The images of IHC staining of RDM1 was performed for RDM1-knockdown (shRDM1) or control (shN) tumours.
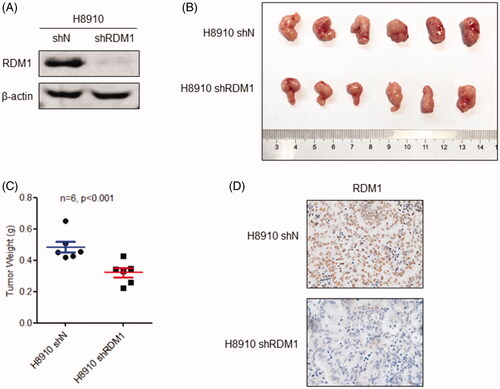
Knockdown of RDM1 induces cell apoptosis in human ovarian carcinoma cells
DNA damage that causes uncontrolled cell proliferation often leads to apoptosis. This suggests that apoptosis is a normal result of the whole mechanism of cell proliferation, that is, apoptosis and cell proliferation are coupled. We therefore detected apoptosis in the RDM1 gene silencing (siRDM1) and control (siN) cells by flow cytometry analysis with double-staining of Annexin V-FITC and propidium iodide (PI). The results showed that siRDM1 cells had higher apoptotic rate than siN cells. For siRDM1 H8910 cells (), higher rate of apoptotic cells (Annexin V+/PI− and Annexin V+/PI+ combined) were detected (8.53% in knockdown cells vs. 2.80% in the control cells). With this same, for SKOV3 cells (), the apoptotic rate of siRDM1 cells (9.57%) was higher than siN cells (4.72%) (Annexin V+/PI− and Annexin V+/PI+ combined). Consistent with the results of RDM1-knockdown cells, the cells overexpressed RDM1 reduce the apoptosis in H8910 and SKOV3 cells (). Therefore, apoptosis were negatively regulated by RDM1 in ovarian carcinoma cells. It is known that apoptosis is closely related to the occurrence of cancer, further suggests that RDM1 may have a carcinogenic function in ovarian carcinoma cells.
Figure 4. RDM1 negatively regulates cell apoptosis in human ovarian carcinoma cells. (A,C) Flow cytometry analysis of Annexin V-FITC and Propidium Iodide (PI) double-stained populations for apoptosis in H8910(A) or SKOV3(C) siRDM1/siN cells. (B,D) The statistic of apoptotic cells percentage to all cells were quantified based on three independent experiments of . Data are expressed as mean ± SD (n = 3). *p < .05; ***p < .001. (E–H) Flow cytometry analysis of H8910 (E,F) and SKOV3 (G,H) cell apoptosis after overexpression of RDM1. Methods were same with (A–D).
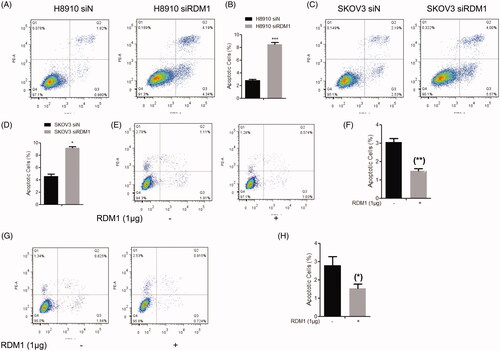
RDM1 regulates p53-RAD52-RAD51 in human ovarian carcinoma cells
TP53 mutation is an important risk factor for ovarian cancer, and it has diverse roles in DNA-repair system as central tumour suppressor. Given that RDM1 is an essential factor in DNA damage pathway, we explored the effect of RDM1 on p53 expression in H8910 and SKOV3 cells, which are human ovarian carcinoma cells expressing wild-type TP53. Western blot results showed that knockdown of RDM1 in H8910 and SKOV3 cells significantly increased the protein level of p53 (). The results suggest that down-regulated expression of p53 may be the reason that RDM1 is a carcinogen in human ovarian carcinoma cells. Currently known that RDM1 could interact with the typical DNA repair factors, such as Rad52. And Rad52 physically interacts with the Rad51 recombinase and serves as a mediator in the Rad51-catalyzed DNA strand exchange reaction [Citation14]. In our study, RDM1 was silenced or overexpressed in H8910 cells in order to investigate the effect of RDM1 on DNA repair factors Rad51 and Rad52. We found that lack RDM1 decreased the protein expression of Rad51 and Rad52 with the increased protein expression of p53 (). It is consistent with the results of human normal ovary and ovarian tumour tissues immunohistochemical staining of RDM1, p53, Rad51 and Rad52 (; Supplementary Figure 1A). In sum, RDM1 regulates p53-RAD52-RAD51 in human ovarian carcinoma cells.
Figure 5. RDM1 regulates p53-RAD51-RAD52 in human ovarian carcinoma cells. (A,B) Protein expression of P53 and RDM1 were detected by western blotting assay in H8910 or SKOV3 siRDM1/siN cells. (C,D) Protein expression of RDM1, P53 RAD51, and RAD52 were detected by western blotting assay in H8910 or SKOV3 siRDM1/siN cells. (E) Representative IHC images showing immunostaining of RDM1, P53 RAD51, and RAD52 in human normal ovary or ovarian tumour tissues.
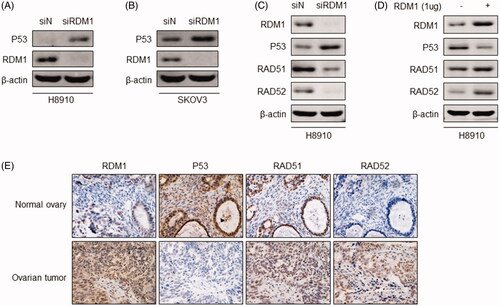
P53 negatively regulates the expression of RAD52 and RAD51 in human ovarian carcinoma cells
It was reported that p53 inhibit the expression of Rad51 at transcriptional level by its specific binding to DNA [Citation15], and p53 has binding sites on Rad51 or Rad52 promoter region. Therefore, we suspected that there may be effects of p53 on Rad51 and Rad52. So we overexpressed p53 and then detected the mRNA and protein expression of Rad51 and Rad52 (). Taken together, these results suggest that RDM1 potentiates RAD52-RAD51 signalling via p53-mediated transcriptional suppression.
Figure 6. p53 negatively regulates the expression of RAD51 and RAD52 in human ovarian carcinoma cells. (A) Protein expression of RAD51, RAD52 and P53 were detected by western blotting assay in H8910 cells with the overexpression of p53. (B) mRNA expression of RAD51, RAD52 and P53 were tested by qRT-PCR analysis in H8910 cells with the overexpression of p53.
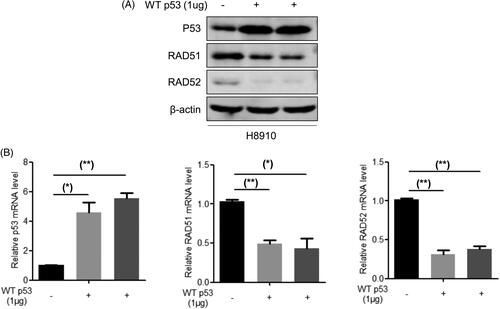
RDM1 regulates the mRNA expression and protein stability of p53 in human ovarian carcinoma cells
Considering that p53 dysregulation may be part of reason of the RDM1 oncogenic mechanism in ovarian carcinoma cells, we next to examine the relationship of regulation between p53 and RDM1. Knockdown of RDM1 increased the mRNA and protein expression of p53-RAD52-RAD51 in H8910 cells (). Meanwhile, p53 protein was more stable after knockdown of RDM1 (). In sum, these results revealed that P53 can be regulated by RDM1 at the transcriptional level in human ovarian carcinoma cells.
Figure 7. RDM1 regulates mRNA expression and protein stability of p53 in human ovarian carcinoma cells. (A,B) Determination of protein (A) and mRNA (B) expression of RAD51, RAD52, P53 and RDM1 in H8910 stabal RDM1 knockdownand normal control cells. (C,D) Protein stability experiment between RDM1 and P53 in H8910 shRDM1/shN cells with Cycloheximide (CHX) treatment.
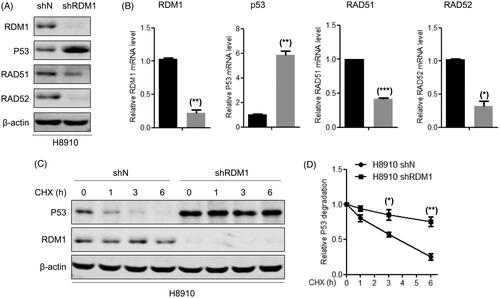
Discussion
RDM1, similar to RAD52, plays a role in DNA repair and homologous recombination. However, the role of RDM1 in the development of cancer is rarely reported. Recent studies showed that RDM1 expressed highly in thyroid papillary carcinoma, lung adenocarcinoma, and breast cancer. But the role of RDM1 in human ovarian cancer is unclear.
In this study, RDM1 was found the carcinogenesis in human ovarian cancer cells. Our results showed that RDM1 expression was up-regulated in mRNA and protein levels in human ovarian cancer. The carcinogenic effect of RDM1 in human ovarian cancer cells may be partly due to the down-regulation of p53 levels. Therefore, this study provides a new potential target for the treatment of ovarian cancer.
Firstly, in our analysis of human ovarian cancer tissue samples, we found that RDM1 was highly expressed in human ovarian cancer tumour tissue compared to human normal ovarian tissue. Therefore, we tentatively hypothesise that RDM1 may play a carcinogenic role in ovarian cancer. Secondly, in vitro experiments showed that the proliferation ability of ovarian cancer cells was decreased and the corresponding apoptosis was increased when RDM1 was knocked down. Conversely, ovarian cancer cells showed increased proliferation and decreased apoptosis when RDM1 was overexpressed. These results suggest that RDM1 may play an important role in the survival and development of ovarian cancer cells. Finally, via mouse xenograft tumour model exprement, we found that cells with low RDM1 expression were significantly inhibited from growing in vivo. These findings provide new evidence for resistance to chemotherapy in ovarian cancer.
Further, we found that RDM1 may regulate p53 expression, since p53 protein expression level is significantly up-regulated in RDM1 knockdown cells. It is well known that p53 have significant effect in regulating cell growth and apoptosis. It can trigger cell growth stagnation or apoptosis by regulating various downstream targets [Citation16]. Therefore, the finding that RDM1 regulates p53 expression may preliminarily explain the weak proliferation and strong apoptosis of cells with low RDM1 expression. However, it remains to be further studied that whether RDM1 regulation of p53 is the main mechanism leading to the decrease of RDM1 knockdown cell proliferation ability and the enhancement of apoptosis ability. At the same time, the specific way of RDM1 regulating p53 is still a problem to be solved. P53 can be regulated at different levels, including transcriptional, epigenetic or post-translational levels. We conducted experiments on the protein stability of p53, and the results showed that RDM1 could affect the protein stability of p53, suggesting that the regulation of RDM1 on p53 may be related to its post-translation level. Therefore, the regulation mechanism can be further studied in this aspect in the future.
In conclusion, our study reveals that RDM1 is a carcinogen in ovarian cancer. In view of the fact that RDM1 can increase the sensitivity of the chemotherapy drug cisplatin and plays an important role in the DNA damage repair pathway [Citation17,Citation18], the relationship between the role of RDM1 in DNA damage repair and its carcinogenic role in ovarian cancer may be investigated in the future. Meanwhile, the specific mechanism of RDM1 regulating p53 also needs to be further studied. In addition, it is of great significance to the question whether the reduction of cisplatin resistance in ovarian cancer patients during chemotherapy can be achieved through RDM1 regulation. These findings may provide new insights into the diagnosis and treatment of ovarian cancer.
Author contributions
Tianying Zhong and Lihua Wang conceived and designed the experiments, Lu Tong, Wenjiao Cao, Jun Sheng, Yajun Chen, Enhao Zhu and Ying Yu performed the experiments and analysed the data. Yajun Chen, Tianying Zhong and Lihua Wang wrote the manuscript.
___1.pdf
Download PDF (289.1 KB)Disclosure statement
No potential conflict of interest was reported by the author(s).
Additional information
Funding
References
- Siegel R, Naishadham D, Jemal A. Cancer statistics, 2013. CA Cancer J Clin. 2013;63(1):11–30.
- Marcus CS, Maxwell GL, Darcy KM, et al. Current approaches and challenges in managing and monitoring treatment response in ovarian cancer. J Cancer. 2014;5(1):25–30.
- Touboul C, Vidal F, Pasquier J, et al. Role of mesenchymal cells in thenatural history of ovarian cancer: a review. J Transl Med. 2014;12:271.
- Anurag M, Punturi N, Hoog J, et al. Comprehensive profiling of DNA repair defects in breast cancer identifies a novel class of endocrine therapy resistance drivers. Clin Cancer Res. 2018;24(19):4887–4899.
- van den Bosch M, Lohman PH, Pastink A. DNA double-strand break repair by homologous recombination. Biol. Chem. 2002;383(6):873–892.
- West SC. Molecular views of recombination proteins and their control. Nat Rev Mol Cell Biol. 2003;4(6):435–445.
- Messaoudi L, Yang Y-G, Kinomura A, et al. Subcellular distribution of human RDM1 protein isoforms and their nucleolar accumulation in response to heat shock and proteotoxic stress. Nucleic Acids Res. 2007;35(19):6571–6587.
- Hamimes S, Arakawa H, Stasiak AZ, et al. RDM1, a novel RNA recognition motif (RRM)-containing protein involved in the cell response to cisplatin in vertebrates. J Biol Chem. 2005;280(10):9225–9235.
- He J, Cui L, Zeng Y, et al. REGγ is associated with multiple oncogenic pathways in human cancers. BMC Cancer. 2012;12:75.
- Li L, Dang Y, Zhang J, et al. REGγ is critical for skin carcinogenesis by modulating the Wnt/β-catenin pathway. Nat Commun. 2015;6:6875.
- Li W, Huang Q, Sun D, et al. RDM1 gene overexpression represents a therapeutic target in papillary thyroid carcinoma. Endocr Connect. 2017;6(8):700–707.
- Tong L, Liu J, Yan W, et al. RDM1 plays an oncogenic role in human lung adenocarcinoma cells. Sci Rep. 2018;8(1):11525.
- Chen Y, Sun Z, Zhong T. RDM1 promotes critical processes in breast cancer tumorigenesis. J Cell Mol Med. 2019;23(8):5432–5439.
- Krejci L, Song B, Bussen W, et al. Interaction with Rad51 is indispensable for recombination mediator function of Rad52. J Biol Chem. 2002;277(42):40132–40141.
- Lazaro-Trueba I, Arias C, Silva A. Double bolt regulation of Rad51 by p53: a role for transcriptional repression. Cell Cycle. 2006;5(10):1062–1065.
- Meek DW. The p53 response to DNA damage. DNA Repair (Amst.). 2004;3(8–9):1049–1056.
- Ciccia A, Elledge SJ. The DNA damage response: making it safe to play with knives. Mol Cell. 2010;40(2):179–204.
- Harper JW, Elledge SJ. The DNA damage response: ten years after. Mol. Cell. 2007;28(5):739–745.