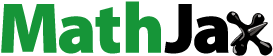
Abstract
Prunella vulgaris L. is one of the therapeutic herbs containing various polyphenolics, which is used for multiple medicinal purposes. In this study, plant growth regulators (PGRs)-induced calli cultures from seed-derived leaf explants were exploited for the production of stress enzymes and polyphenolics. A growth curve was plotted for each PGR for 49 days period, which showed a distinct lag, log and decline phases. Here, the combination of naphthalene acetic acid (NAA) and 6-benzyleadenine (BA; 0.5 and 2.0 mg l−1) produced maximum fresh (6.32 FW-g/100 ml) and dry biomass (0.75 DW-g/100 ml) in contrast to control. The maximum synthesis of SOD (0.0154 FW-nM/min/mg) was detected on media comprising mixture of NAA and BA (1.5 mg l−1), while POD enzyme (0.366 FW-nM/min/mg) was higher at 0.5 mg l−1 NAA and 2, 4-dichlorophenoxy acetic acid. Further, NAA and BA (1.5 and 2.0 mg l−1) boosted up the synthesis of phenolics (18.83 GAE-mg/g-DW) and flavonoids content (18.05 RE-mg/g-DW) than control. Moreover, NAA of 1.0 and 2.0 mg l−1 were found supportive for maximum antioxidant activity (87.4%) and total protein (716 µg BSAE/mg-DW). This study will contribute in the development of cell culture in fermenter and synthesis of antioxidant secondary metabolites for commercial uses.
Introduction
Prunella vulgaris L. is perennial, edible and wound healing plant from Lamiaceae family. Ustakhudus is its traditional name, but due to its wound healing properties, it is also known by ‘self-heal and all-heal’. This plant was traditionally utilised by various tribes for the treatment of various ailments since unknown time in countries including North America, China and Europe [Citation1,Citation2]. Numerous studies revealed that P. vulgaris have a broad spectrum of therapeutic potentials, which include antiseptic, anti-cancerous, antispasmodic, antirhematic and anti-HSV etc. [Citation3,Citation4]. Some reports showed that it is used to treat sore throat, inflammations, pains, fevers and also useful in decreasing high blood pressure [Citation5]. Recent studies conducted by many scientists suggest that this herb is very potentials against Human immune virus and Herpes viruses [Citation6]. The biological activities of P. vulgaris are because of the presence of many active phytochemicals. Recently its demand for therapeutic industries is higher than its production. But interestingly, lesser information on its synthesis under field and in vitro environments is available. For the synthesis of consistent plantlets and active secondary metabolites, this therapeutically significant herb needs reliable techniques.
During unfavourable conditions, various reactive oxygen species (ROS) are produced which interfere with biological molecules and cause multiple problems such as inhibition of growth, differentiation and metabolite production. The antioxidant system either utilised endogenous enzyme, which includes Superoxide dismutase (SODs) and Peroxidase (PODs) or non-enzyme compound, which include polyphenolics that counter act the detrimental impact of Reactive oxygen species [Citation7]. SODs enzymes have a potential role in the development of plant [Citation8]. SODs are the members of metallo enzymes family. In plants SODs carry out the conversion of the oxygen radicals to hydrogen peroxide in unfavourable conditions [Citation9]. The H2O2 is one of the most significant ROS that can causes severe cell damage due to its ability to easily cross the cell. PODs enzyme is thought to have a crucial role in auxins catabolism, plant development, differentiation process, morphogenesis, and cell wall formation [Citation10]. Furthermore, non-enzymatic active components participate in extinguishing toxic free radicals, boost up immune system, apoptosis induction, turn on or off gene expression, interaction with cell-cycle and arrest enzyme activation [Citation1]. To protect the plants from UV radiation, physiological damage, environmental stresses, and pathogenic attacks, these compounds play a key role. To boost up the synthesis of such metabolites, which are either synthesise by parental plant in least quantities or hard to produce under laboratory conditions, so plant cell, tissue and organ culture is a competent system for these purposes [Citation1]. Polyphenols are the major groups among various classes of phytochemicals, which exhibited higher antioxidant activity in contrast to vitamins and carotenoids [Citation10]. Usually, there is a strong correlation between phenolic and flavonoids with antioxidant activity [Citation10].
The main aim of this study was to investigate the combination of PGRs on calli induction from leaf explants, growth kinetics, biomass production, biosynthesis of antioxidative enzymes, accumulation of polyphenolics, biosynthesis of total protein content and to test various PGRs-induced calli cultures for antioxidant activity By using leaf explants, this is the first feasible protocol for callus induction in P. vulgaris containing potential for up scaling to bioreactor for increased production of metabolite of interest.
Methods
Explant selection and combination of PGRs for callus induction
Fresh leaves were excised from P. vulgaris in vitro seed derived plantlets. About 3–4 mm2 leaf fragments were kept on MS media incorporated with various treatments of plant growth regulators (PGRs). In preliminary experiments (data not shown) various auxins and cytokinins were applied for callogenesis but further experiments were carried out by the addition of effective PGRs only. The rationale of the current study was to produce maximum callogenesis by the addition of selected concentrations of Naphthalene acetic acid (NAA; 0.5, 1.0, 1.5 and 2.0 mg l−1) or half MS with similar concentrations of NAA or mixture of NAA with 6-Benzyladenine (BA; 0.5, 1.0, 1.5 and 2.0 mg l−1) or various concentrations and mixture of 2,4-dichlorophenoxyacetic acid (2,4-D) and NAA, 2, 4-D individually or either in combination with BA or NAA, or in addition with gibberellic acid (GA3) for callus induction. The MS media was solidified by the addition of 8 g l−1 agar and improved with 30 g l−1 sucrose, the pH was set on 5.3, and for 20 min at 121 °C, all the media were sterilised. Entire cultures were sustained in a growth room for 16/8 h photoperiod provided through fluorescent white-cool tube lights with light intensity of ∼40–50 µmol m−2 s−1 and at 25 ± 1 °C. As a control, MS basal media (MS0) was used lacking any PGRs. In the 100 ml flask comprising ∼25 ml of media, around 5–6 explants were inoculated. Various parameters concerning to data of calli were recorded after ∼4 weeks.
Growth kinetics
Growth curve was plotted for the biomass production of the fast growing calli obtained from five sets of Plant growth regulators. Set-1 containing different concentrations of NAA, Set-2 containing half MS with different concentrations of NAA, Set-3 containing combination of auxin and cytokinine (NAA and BA), Set-4 containing mixture of strong and weak auxin (NAA and 2, 4-D) and Set-5 comprising 2, 4-D, BA, NAA and GA3. Solid calli was collected for fresh biomass (FW). These calli was pragmatically washed to eradicate excess of water and then it was pressed slightly on filter paper and weighed. Similarly, at 50 °C the calli was desiccated in an oven for determination of dry biomass (DW), and finally weighed. The calli fresh and dry biomass were taken in gram/flask.
Quantification of antioxidative enzyme
Quantification of enzymatic activities (POD and SOD) was investigated by adopting the Fazal et al. [Citation1] protocol. Practically, with an extraction buffer of 10 ml (1% PVPP with 50 mM KH2PO4 buffer at pH 7), the fresh sample (1 g) was added to a test tube and gently mixed. For 30 min at 4 °C, the solution was then centrifuged (10,000 rpm). In sterile tube, the supernatant was obtained and afterward it was applied for enzyme assay.
Quantification of non-enzymatic compounds
Dried calli was converted to powder using fruit grinder. Accurately, 10 ml of ethanol, and 10 mg powdered was mixed and left for 3 days on shaker. The supernatant was obtained through centrifugation at 10,000 rpm for 15 min and then applied for TFC and TPC. In every sample, TPC was observed by applying Fazal et al. [Citation1] recent procedure. At a wavelenghth of 760 nm, the absorbance of solution was determined. Gallic acid (Sigma; 1.0–10 mg/ml; R2 = 0.9878) was utililzed to plot standard calibration curve. The total TPC as Gallic acid equivalent (GAE) mg/g on dry weight basis was determined as
where the sample absorbance is indicated by AS and absorbance of blank is indicated by AB. The conversion factor is denoted by CF and dilution factor by DF.
By applying the Fazal et al. [Citation1] protocol, the TFC in dried biomass was observed. Through UV-visible spectrophotometer, at 510 nm, the absorbance was observed. Rutin (Sigma; 1.0–10 mg/ml; R2 = 0.9866) was utilised to established standard calibration curve. The TFC was taken as rutin equivalent (RE) mg/g-DW of extracts.
Investigation of total protein content
The procedure applied by Giri et al. [Citation11] was applied for protein analysis preparation. Practically, in a chilled mortar and pestle, 4 g of calli were grounded and 0.2 g was mixed with methanol (1 ml) and then for 5 min, it was incubated in a cold room. The solution was subsequently applied for 5 min to sonication (Toshiba, Japan), 20 min to vortexing and then centrifuged for 5 min at 10,000 rpm to obtain supernatant. For various concentrations of the standard solutions, a regression curve was plotted in contrast to their respective absorbance. The procedure followed by Fazal et al. [Citation12] was practiced for investigation of total protein content. Through spectrophotometer at 650 nm, the supernatant absorbance was measured.
Antioxidant activity
DPPH radical scavenging activity (DRSA) in various callus samples was investigated [Citation1]. Briefly, with DPPH free radical solution of 2.0 ml (0.25 mg/20 ml ×4) the ethanolic calli extract (1.0 ml; 5 mg/20 ml) of every sample was combined. For about 30 min in dark, the mixture was incubated. Through UV-visible spectrophotometer at 517 nm, the resulted mixture absorbance at room temperature was measured. In the end, as percentage of DPPH discolouration, the radical scavenging activity was determined through the equation given below:
In which AP indicated absorbance of calli extract and the DPPH solution absorbance without calli extract was indicated by AD.
Statistical analysis
Each treatment was comprised of three replicates and all experiments were repeated two times. Average values of different treatments were exposed to the analysis of variance (ANOVA). Statistix software (8.1 versions) was utilised for standard errors (±). For graphical presentation, Origin Lab (8.5) software was processed.
Results
Callus formation, biomass production and growth curve establishment
Callogenic efficiency of the leaf explants in P. vulgaris was monitored on MS media augmented with mixture of various PGRs. NAA was found the finest growth regulator for callogenesis either individually or in addition to other PGRs. Half MS-medium combined with various NAA applications (0.5–2.0 mg l−1) produced > 80% response, whereas, higher NAA concentrations (1.5–2.0 mg l−1) induced above 90% callogenic response (). Seed derived leaf explant () displayed responses to all tested PGRs. Here the combination of NAA either with 2, 4-D or BA induced more than 90% calli from leaf pieces. Though, the mixture of 2, 4-D, NAA and BA (0.5 mg l−1) showed 68.75% response. It means that strong (2, 4-D) and weaker auxin (NAA) along with strong cytokinine (BA) inhibited cell division and calli proliferation. The calli formation and colour (transparent-half white-creamy-yellowish-light greenish-brownish green-golden-brown) varied significantly between the control and PGRs containing treatments after 4–5 weeks of explants inoculation. Similarly, the morphological characteristics of induced calli varied significantly from friable and spongy to hairy and granular (; ).
Figure 1. The vegetative stage of P. vulgaris (a) flowering stage of P. vulgaris (b), and seed maturation stage of P. vulgaris (c).
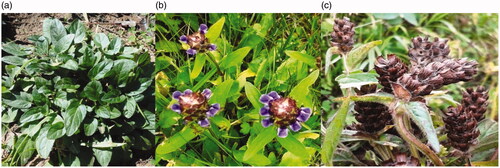
Figure 2. Effects of various plant growth regulators on callus growth in P. vulgaris (a) callus induction on MS medium containing 0.5 (b) 1.0 (c) 1.5 (d) 2.0 mg l−1 NAA (e) callus induction on half MS medium containing 0.5 NAA (f) 1.0 NAA (g) 1.5 NAA (h) 2.0 NAA (i) 0.5 NAA + BA (j) 1.0 NAA + BA (k) 1.5 NAA + BA (l) 2.0 NAA + BA (m) 0.5 NAA + 1.0 2, 4-D (n) 0.5 NAA + 2.0 2, 4-D (o) 1.0 NAA + 0.5 2, 4-D (p) 1.0 NAA + 1.0 2, 4-D (q) 2.0 NAA + 0.5 2, 4-D (r) 2.0 NAA + 2.0 2, 4-D (s) 1.0 2, 4-D (t) 2.0 2, 4-D (u) 1.0 2, 4-D + 1.0 BA (v) 0.5 2, 4-D + 0.5 BA + 0.5 NAA (w) 1.0 2, 4-D + 1.0 GA3 (x) 2.0 2, 4-D + 2.0 GA3.
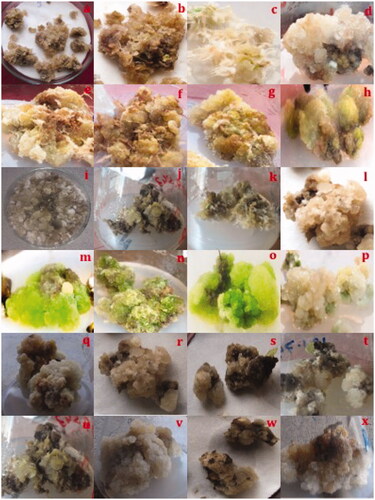
Table 1. Effects of combinations of PGRs on callus culture formation, morphological features, fresh and dry weights.
For a period of 7 weeks with 7 days intervals, the calli biomass accumulation and growth kinetics in response to various PGRs were determined (). When various NAA concentrations (0.5–2.0 mg l−1) were used alone, the lag, log and decline phases shows variation in biomass accumulation (). From 7 to 21 days, increments in biomass accumulation were slower, however, maximum biomass accumulation was observed at day 35 and subsequently the decline phase started later on day 42 and the calli colour started brown. Among all the concentrations tested, 1.0 mg l−1 of NAA induced maximum fresh biomass (0.81 g/100 ml) during growth kinetics at day 35, however, at the end of the experiment, the accumulated dry (4.03 g/100 ml) and fresh biomass (0.29 g/100 ml) were found maximum at 1.5 mg l−1 NAA. Once half MS media was augmented with various NAA concentrations, the biomass formation exhibited a 7–18-day lag phase with moderately slower development (). Maximum fresh biomass (0.76 g/100 ml) was observed at 42-day and the decline phase started after 42 days. A similar observation was recorded in case of combinations of NAA and BA with similar lag, log and decline phases (). However, a completely different lag, log and decline phases in terms of biomass accumulation were observed when multiple NAA concentrations and 2, 4-D was applied (). But with BA, when lower concentrations of 2, 4-D and NAA were mixed, the culture exhibited a 7–21 days lag phases and then 3–4-time surge in fresh biomass was determined on day 35 and then decline phase started ().
Figure 3. Effect of full strength MS media with different concentrations of NAA (a) or half strength MS media with NAA (b) or either, NAA with BA (c) or various concentrations of NAA with 2, 4-D (d) or 2, 4-D individually or 2, 4-D in addition with GA3 (e) on growth kinetics and fresh biomass production. Data was collected for & 7 weeks with 7 days intervals. From three replicates, values are the mean ± standard error.
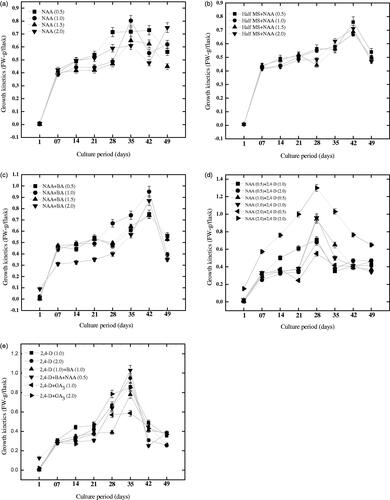
Biosynthesis of antioxidative enzymes
In this study, the main enzymatic components (SOD and POD) and different PGRs applied were investigated and correlated with biomass accumulation. PGRs significantly influenced FW, SOD and POD production during callogenesis. Half MS + NAA alone or full MS + NAA in mixture with BA (2.0 mg l−1) boosted up FW (5.91 and 6.32 g/100 ml) but the synthesis of SOD (0.0032 and 0.0023 FW-nM/min/mg) and POD (0.121 and 0.2134 FW-nM/min/mg) was lower as compared to biomass accumulation (). It means that SOD and POD production is reliant on PGRs applied rather than biomass accumulation. Maximum SOD (0.0154 and 0.01526 FW-nM/min/mg) production was detected with mixture of 2, 4-D and GA3 (2.0 mg l−1), and NAA and BA (1.5 mg l−1) but at this time, the synthesis of POD (0.086 and 0.121 FW-nM/min/mg) was found lower. It means that SOD and POD production is not restricted to specific growth regulators but vary with application of different PGRs. The SOD and POD enzyme sometime worked together to combat with ROS or sometime exhibit antagonistic effect because when SOD increases the POD production decreases. Similarly maximum POD synthesis (0.366 and 0.344 FW-nM/min/mg) was detected on media containing mixture of either 2, 4-D with NAA (0.5 mg l−1) or 2, 4-D with BA (1.0 mg l−1) but the synthesis of SOD (0.0034 and 0.0104 FW-nM/min/mg) was lowered ().
Biosynthesis of polyphenolics content
Here, phenolics and flavonoids, a non-enzymatic defense system component was examined. These compounds were correlated with DW and in response to different PGRs applied. Herewith, dry biomass accumulation did not exhibit linear relationship with polyphenolics production because high DW (0.754 g/100 ml) was detected on medium having NAA and BA (0.5 mg l−1), but the synthesis of phenolics (13.99 GAE-mg/g-DW) and flavonoids (15.41 RE-mg/g-DW) was found lower as compared to other treatments (). Maximum NAA and BA concentrations (2.0 mg l−1) boosted up phenolics synthesis (18.83 GAE-mg/g-DW) but lowered flavonoids (13.62 RE-mg/g-DW) and DW (0.5 g/100 ml). Contrarily, NAA and BA (1.5 mg l−1) influenced the synthesis of flavonoids (18.05 RE-mg/g-DW) greater than phenolics (9.73 GAE-mg/g-DW) and dry biomass. Among the applied NAA concentrations, 1.0 mg l−1 enhanced biomass (0.297 g/100 ml-DW), phenolics (8.86 GAE-mg/g-DW) and content of flavonoids (16.97 RE-mg/g-DW). During the augmentation of different NAA concentrations to half MS media, NAA of 1.5 mg l−1 gave the best results in terms of biomass (0.436 g/100 ml-DW), TPC (14.38 GAE-mg/g-DW) and TFC (6.77 RE-mg/g-DW). Furthermore, other PGRs combinations significantly reduced dry biomass and the production of polyphenolics content ().
Protein content and antioxidant activity
Total protein content in P. vulgaris calli cultures exposed to various concentrations of PGRs was investigated (). Among various treatments of NAA (0.5–2.0 mg l−1) tested, 1.5 mg l−1 was found supportive in the biosynthesis of protein content (716 µg BSAE/mg-DW). Content of total protein was also tested in calli induced on half MS media containing various NAA concentrations. Here, 0.5 mg l−1 boosted up the production of total protein (834 µg BSAE/mg-DW) in contrast to control and other treatments (). Moreover, the mixture of NAA and BA (1.5 mg l−1) showed a positive effect on the total protein production (492 µg BSAE/mg-DW) than other NAA and BA combinations. From the current results, we concluded that the content of total protein showed positive addiction with applied PGRs like that of enzymatic and non-enzymatic components. Contrarily to the above PGRs, the addition of 2, 4-D individually, or either in mixture with BA or NAA or with GA3 significantly reduced the total protein content as earlier observed in the case of polyphenolics production. It means that PGRs boost up the synthesis of significant secondary metabolites that are autonomous from biomass production. In the current study, maximum DRSA (87.4%) was observed in calli obtained from half MS-media comprising NAA (1.0 mg l−1) (). Similarly, less NAA (0.5 mg l−1) also influenced DRSA (77.81%) in calli cultures. Other growth regulators individually or in combination have less potential in increasing DRSA but the mixture of 2, 4-D, NAA and BA (0.5 mg l−1) influenced DRSA (77.53%) respectively (Supplementary material).
Discussion
During culture establishment from leaf explants of P. vulgaris, NAA was found the best PGR for maximum callogenic frequency. Higher NAA concentration (1.5 mg l−1) produced > 90% calli cultures, however, the combination of NAA either with strong auxin (2, 4-D) or strong cytokinine (BA) also enhanced callus formation. No reports exist regarding calli induction in P. vulgaris in the literature, however, the organogenesis was reported on media having mixture of NAA and BA [Citation3]. The use of NAA individually or in addition to other PGRs boosted up callus formation in Artemisia absinthium [Citation13]. Recently, Fazal et al. [Citation12] stated that various NAA concentrations (0.5–2.0 mg l−1) enhanced adventitious root cultures in P. vulgaris.
In lag phases from day 7 to 21 days, the cell division and biomass accumulation were found slower in growth kinetics. In various NAA augmented cultures, maximum biomass production was detected in log phases on day 35. After 35 days, most of the cultures start browning that may possibly due to the depletion of nutrients. Here, 1.0 mg l−1 NAA was found potential for fresh biomass production, however at the end of experiment; 1.5 mg l−1 NAA boosted up dry (0.29 g/100 ml) and fresh biomass (4.03 g/100 ml) respectively. Half MS-media improved with NAA alone and mixture of NAA and BA has shown a similar pattern of biomass accumulation in lag, and decline period of growth kinetics. These results concluded that biomass production is significantly boosted up by NAA. These results are parallel with those reported by Ali and Abbasi [Citation13]. Fazal et al. [Citation12] observed that various NAA concentrations significantly influenced biomass accumulation of adventitious roots throughout lag, log and decline periods in P. vulgaris.
Cell differentiation and dedifferentiation because of unwanted production of ROS is hinder by various stresses during callogenesis and morphogenesis. This ROS directly destruct cells via the synthesis of toxic compounds [Citation14]. So, during cell division, the plant synthesise specific enzyme to overcome such oxidative stress [Citation15]. Toxic free radicals and their associated non-radical oxygen species are scavenged by complex antioxidant protection system like CAT, SOD, POD, and APX, which is form, by those enzymes [Citation14]. The SOD and POD defense enzymes were found to be PGRs dependent and biomass independent. Because when half and full MS medium was augmented either with NAA or mixture of NAA and BA (2.0 mg l−1), the SOD and POD enzymes decreases and biomass increases. However, mixture of BA and NAA (1.5 mg l−1) stimulate the production of SOD enzymes but reduced the POD enzymes. It means that each component of enzymatic defense system is not restricted to specific PGR but varies with the application of various groups of PGRs. Unfortunately, During callogenesis the role of P. vulgaris antioxidative enzymes is less known. Though, Abbasi et al. [Citation14] described clear association between antioxidative enzyme synthesis in Brassica rapa during organogenesis and callogenesis. In Prunus, Gladiolus and Solanum species, surge in POD and decline in SOD synthesis in organogenesis were also reported [Citation9,Citation16,Citation17]. Meratan et al. [Citation18] described that calli-secreted minimum SOD, whereas boosted during organogenesis of Acanthophyllum sordidum. Furthermore, under different conditions in various therapeutic plants, the enzymatic role of SOD and POD is extensively reported [Citation9,Citation19].
During unusual conditions, a chain reaction is initiated due to the released of toxic free radicals in larger quantities which inhibit cells or tissues. The active member of natural antioxidants such as phenols is release by different plant species [Citation20]. Hence scavengers of toxic free radicals and singlet O2 formation are the plant-based antioxidants. The ROS modified DNA molecule and stop manufacturing of proteins and lipids [Citation21]. The plant-based antioxidants are most efficient because of the existence of flavonoid and particularly phenolic compounds. Through a shikimic pathway and through carboxylic acid or benzoic acid, the polyphenolics are synthesised in intact plant or in vitro cultures. Polyphenols such as lignans, xanthones, caffeic acid derivatives, ellegitannins, gallotannins, and stilbenes are produced under in vitro cultures and utilised as antioxidants [Citation21].
Similar to enzymatic defense components, the non-enzymatic polyphenolics were also found to be PGRs dependent and biomass independent. The application of 0.5 mg l−1 NAA boosted up biomass production but reduced polyphenolics biosynthesis. Further, 2.0 mg l−1 NAA and BA improved synthesis of phenolics and 1.5 mg l−1 NAA and BA enhanced flavonoids on full MS-media. However, the half MS-media in combination with various NAA concentrations was found optimum for production of polyphenolics. Seldom changes in plant secondary metabolites occur during in vitro callogenesis. SODs, PODs, TPC and TFC defense compounds are produced during abiotic and biotic stresses and promote the development and growth of plant cells and tissues. It is beneficial for the production of TPC and TFC to used plant tissue culture. In the literature, specific reports on the P. vulgaris calli cultures for the production of TPC and TFC are not available. Though, in various cultures of therapeutic herbs, different researchers reported similar TPC and TFC biosynthesis [Citation22,Citation23]. Naz et al. [Citation24] described that callus cultures of Cicer arietinum yielded higher phenolics like the current study. Ghasemzadeh et al. [Citation25] reported maximum production of polyphenolics content in two varieties of Zingiber officinale. Hemm et al. [Citation26] and Liu et al. [Citation27] reported that the organogenic potential is not the only one affected by PGRs and elicitors, but it also affects metabolites production.
On full MS-media, 1.5 mg l−1 of NAA was found efficient for the production of total protein content in P. vulgaris calli culture while 0.5 mg l−1 NAA boosted up the production of protein than control. From the current results, we concluded that the content of total protein showed positive addiction with applied PGRs like that of enzymatic and non-enzymatic components. DPPH is cost-effective, simplest and quick procedure that is utilised for investigation of antioxidant potential of plant cells/derived products [Citation28]. For high valued secondary metabolites production, plants in vitro cultures are extensively exploited [Citation29]. Calli obtained from half MS-media containing 1.0 mg l−1 NAA boosted up the DRSA (87.4%) than other treatments and control. Various factors affect the metabolites production during in vitro cultures [Citation30]. Reliant on plant species, the metabolites accumulation is affected by auxin and cytokinine individually or their mixture. Gulluce et al. [Citation31] reported that in Satureja hortensis calli the DPPH activity was higher in contrast to synthetic butylated hydroxytoluene agent. In previous studies, it has been observed that the addition of NAA along with elicitors (nanoparticles) enhanced the production of antioxidant metabolites in cell cultures of P. vulgaris [Citation1]. Similarly, the NAA along with nanoparticles also stimulated the production of biomass and secondary components in calli cultures of P. vulgaris [Citation10]. The combination of polyamines and NAA also plays a key role in the micropropagation of P. vulgaris [Citation2]. The addition of NAA helps in the formation of callus and adventitious roots in liquid media. The supplementation elevated biomass accumulation and secondary metabolites in adventitious root cultures of P. vulgaris [Citation12]. Moreover, Fazal et al. [Citation32] observed that the combination of NAA with different photoperiods, pH levels and differential sucrose enhanced the biosynthesis of antioxidant secondary metabolites in cell cultures of P. vulgaris. The exposure of calli to various spectral lights on solid MS media containing NAA enhanced the callogenesis frequency and morphological characteristics in P. vulgaris [Citation33]. In another study, Fazal et al. [Citation34] observed that NAA and melatonin in half and full strength media stimulated the production of stress enzymes. These studies and the current results suggest that NAA play a key role in various cultures development of P. vulgaris and biosynthesis of valuable secondary metabolites.
Conclusion
In conclusion, the present results showed that callus formation frequency, production of phenolics, flavonoids, total protein content and antioxidant potential in vitro in calli cultures were positively regulated by mixtures of plant growth regulators instead of biomass accumulation. In the formation of calli, the antioxidative enzymes contribute up to some extent but their contribution has yet to be investigated at the molecular level which provides information for researcher to know their chemistry for future health problems. These results have the potential to be used for commercial synthesis of desirable secondary metabolites by exploiting cell cultures in bioreactor technology.
Data_of_Revised_LABB_2019_1993..docx
Download MS Word (69.2 KB)Disclosure statement
The authors declare that they have no conflict of interest regarding this study.
Additional information
Funding
References
- Fazal H, Abbasi BH, Ahmad N, et al. Sustainable production of biomass and industrially important secondary metabolites in cell cultures of selfheal (Prunella vulgaris L.) elicited by silver and gold nanoparticles. Artif Cells Nanomed Biotechnol. 2019;47(1):2553–2561.
- Fazal H, Shinwari ZK, Ahmad N, et al. Factors influencing in vitro seed germination, morphogenetic potential and correlation of secondary metabolism with tissue development in Prunella vulgaris L. Pak J Bot. 2016a;48(1):193–200. 2016a.
- Rasool R, Kamili AN, Ganai BA, et al. Effect of BAP and NAA on shoot regeneration in Prunella vulgaris. J Nat Sci Math. 2009;3(1):21–26.
- Chen CY, Wu G, Zhang MZ. The effects and mechanism of action of Prunella vulgaris L. extract on Jurkat human T lymphoma cell proliferation. Chin Ger J Clin Oncol. 2009;8(7):426–429.
- Golembiovska OI, Tsurkan AA. Anthocyanins profiling of Prunella vulgaris L. grown in Ukraine. Pharm Innov. 2013;2(6):42–48.
- Valko M, Leibfritz D, Moncol J, et al. Free radicals and antioxidants in normal physiological functions and human disease. Int J Biochem Cell Biol. 2007;39(1):44–48.
- Racchi ML, Bagnoli F, Balla I, et al. Differential activity of catalase and superoxide dismutase in seedlings and in vitro micropropagated oak (Quercus robur L.). Plant Cell Rep. 2001;20(2):169–174.
- Gupta SD, Datta S. Antioxidant enzyme activities during in vitro morphogenesis of gladiolus and the effect of application of antioxidant on plant regeneration. Biol Plant. 2004;47(2):179–183.
- Joo SS, Kim Y, Lee DI. Antimicrobial and antioxidant properties of secondary metabolites from white rose flower. Plant Pathol. J. 2010;26(1):57–62.
- Fazal H, Abbasi BH, Ahmad N, et al. Elicitation of medicinally important antioxidant secondary metabolites with silver and gold nanoparticles in callus cultures of Prunella vulgaris L. Appl Biochem Biotechnol. 2016b;180(6):1076–1092.
- Giri (1, ) L, Dhyani P, Rawat S, et al. In vitro production of phenolic compounds and antioxidant activity in callus suspension cultures of Habenaria edgeworthii: a rare Himalayan medicinal orchid. Ind Crop Prod. 2012;39:1–6.
- Fazal H, Abbasi BH, Ahmad N. Optimization of adventitious root culture for production of biomass and secondary metabolites in Prunella vulgaris L. Appl Biochem Biotechnol. 2014;174(6):2086–2095.
- Ali M, Abbasi BH. Thidiazuron-induced changes in biomass parameters, total phenolic content, and antioxidant activity in callus cultures of Artemisia absinthium L. Appl Biochem Biotechnol. 2014;172(5):2363–2376.
- Abbasi BH, Khan M, Guo B, et al. Efficient regeneration and antioxidative enzyme activities in Brassica rapa var. turnip. Plant Cell Tiss Organ Cult. 2011;105(3):337–344.
- Abbasi BH, Saxena PK, Murch SJ, et al. Echinacea biotechnology: challenges and opportunities. In Vitro Celldevbiol-Plant. 2007;43(6):481–492.
- Kumar GNM, Knowles NR. Changes in lipid peroxidation and lipolytic and free radical scavenging enzyme activities during aging and sprouting of potato (Solanum tuberosum) seed-tubers. Plant Physiol. 1993;102(1):115–124.
- Franck T, Kevers C, Gaspar T. Protective enzymatic systems against activated oxygen species compared in normal and hyperhydric shoots of Prunus avium L. raised in vitro. Plant Growth Regul. 1995;16(3):253–256.
- Meratan AA, Ghaffari SM, Niknam V. In vitro organogenesis and antioxidant enzymes activity in Acanthophyllum sordidum. Biol Plant. 2009;53(1):5–10.
- Sreelatha S, Padma PR. Antioxidant activity and total phenolic content of Moringa oleifera leaves in two stages of maturity. Plant Foods Hum Nutr. 2009;64(4):303–311.
- Kosar M, Goger F, Baser K. In vitro antioxidant properties and phenolic composition of Salvia halophila Hedge from Turkey. Food Chem. 2011;129(2):374–379.
- Matkowski A. Plant in vitro culture for the production of antioxidants-a review. Biotechnol Adv. 2008;26(6):548–560.
- Parsaeimehr A, Sargsyan E, Javidnia K. A comparative study of the antibacterial, antifungal and antioxidant activity and total content of phenolic compounds of cell cultures and wild plants of three endemic species of Ephedra. Molecules. 2010;15(3):1668–1678.
- Costa P, Gonçalves S, Valentão P, et al. Thymus lotocephalus wild plants and in vitro cultures produce different profiles of phenolic compounds with antioxidant activity. Food Chem. 2012;135(3):1253–1260.
- Naz S, Ali A, Iqbal J. Phenolic content in vitro cultures of chick pea (Cicer arietinum L.) during callogenesis. Pak. J. Bot. 2008;40(6):2525–2539.
- Ghasemzadeh A, Jaafar HZE, Rahmat A, et al. Effect of different light intensities on total phenolics and flavonoids synthesis and anti-oxidant activities in young ginger varieties (Zingiber officinale Roscoe). Int J Mol Sci. 2010;11(10):3885–3897.
- Hemm MR, Rider SD, Ogas J, et al. Light induces phenylpropanoid metabolism in Arabidopsis roots. Plant J. 2004;38(5):765–778.
- Liu CZ, Guo C, Wang YC, et al. Effect of light irradiation on hairy root growth and artemisinin biosynthesis of Artemisia annua. Process Biochem. 2002;38(4):581–585.
- Khan MA, Abbasi BH, Ahmed N, et al. Effects of light regimes on in vitro seed germination and silymarin content in Silybum marianum. Ind. Crop Prod. 2013;46:105–110.
- Abouzid SF, El-Bassuon AA, Nasib A, et al. Withaferin A production by root cultures of Withania coagulans. Int J Appl Res Nat Prod. 2010;3(1):23–27.
- Duangporn P, Siripong P. Effect of auxin and cytokinin on phyllanthusol A production by callus cultures of Phyllanthus acidus Skeels. American-Eurasian J Agric Environ Sci. 2009;5:258–263.
- Güllüce M, Sökmen M, Daferera D, et al. In vitro antibacterial, antifungal, and antioxidant activities of the essential oil and methanol extracts of herbal parts and callus cultures of Satureja hortensis L. J Agric Food Chem. 2003;51(14):3958–3965.
- Fazal H, Abbasi BH, Ahmad N, et al. Sucrose induced osmotic stress and photoperiod regimes enhanced the biomass and production of antioxidant secondary metabolites in shake-flask suspension cultures of Prunella vulgaris L. Plant Cell Tiss Organ Cult. 2016c;124(3):573–581.
- Fazal H, Abbasi BH, Ahmad N, et al. Correlation of different spectral lights with biomass accumulation and production of antioxidant secondary metabolites in callus cultures of medicinally important Prunella vulgaris L. J Photochem Photobiol B, Biol. 2016d;159:1–7.
- Fazal H, Abbasi BH, Ahmad N, et al. Exogenous melatonin trigger biomass accumulation and production of stress enzymes during callogenesis in medicinally important Prunella vulgaris L. (Selfheal). Physiol Molecul Biol Plant. 2018;24:1307–1315.